« Prev Next »
Introduction
Polyploidy is the heritable condition of possessing more than two complete sets of chromosomes. Polyploids are common among plants, as well as among certain groups of fish and amphibians. For instance, some salamanders, frogs, and leeches are polyploids. Many of these polyploid organisms are fit and well-adapted to their environments. In fact, recent findings in genome research indicate that many species that are currently diploid, including humans, were derived from polyploid ancestors (Van de Peer & Meyer, 2005). These species that have experienced ancient genome duplications and then genome reduction are referred to as paleopolyploids. This article discusses the mechanisms underlying polyploidy, and both the advantages and disadvantages of having multiple sets of chromosomes.
Mechanisms of Polyploidy
Researchers usually make a distinction between polyploids that arise within a species and those that arise due to the hybridization of two distinct species. The former are known as autopolyploids, while the latter are referred to as allopolyploids. Autopolyploids are essentially homozygous at every locus in the genome. However, allopolyploids may have varying degrees of heterozygosity depending on the divergence of the parental genomes. Heterozygosity is apparent in the gametes that polyploids produce. Allopolyploids can generally be distinguished from autopolyploids because they produce a more diverse set of gametes (Figure 2).
Different species exhibit different levels of tolerance for polyploidy. For example, polyploids form at relatively high frequency in flowering plants (1 per 100,000 individuals), suggesting that plants have a remarkably high tolerance for polyploidy. This is also the case for some species of fish and frogs. However, higher vertebrates do not appear to tolerate polyploidy very well; in fact, it is believed that 10% of spontaneous abortions in humans are due to the formation of polyploid zygotes.
Advantages of Polyploidy
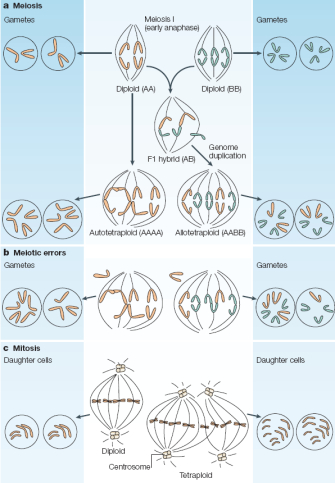

Due to the high incidence of polyploidy in some taxa, such as plants, fish, and frogs, there clearly must be some advantages to being polyploid. A common example in plants is the observation of hybrid vigor, or heterosis, whereby the polyploid offspring of two diploid progenitors is more vigorous and healthy than either of the two diploid parents. There are several possible explanations for this observation. One is that the enforced pairing of homologous chromosomes within an allotetraploid prevents recombination between the genomes of the original progenitors, effectively maintaining heterozygosity throughout generations (Figure 3). This heterozygosity prevents the accumulation of recessive mutations in the genomes of later generations, thereby maintaining hybrid vigor. Another important factor is gene redundancy. Because the polyploid offspring now have twice as many copies of any particular gene, the offspring are shielded from the deleterious effects of recessive mutations. This is particularly important during the gametophyte life stage. One might envision that, during the haploid stage of the life cycle, any allele that is recessive for a deleterious mutation will not be masked by the presence of a dominant, normally functioning allele, allowing the mutation to cause developmental failure in the pollen or the egg sac. Conversely, a diploid gamete permits the masking of this deleterious allele by the presence of the dominant normal allele, thus protecting the pollen or egg sac from developmental dysfunction. This protective effect of polyploidy might be important when small, isolated populations are forced to inbreed.
Another advantage conferred by gene redundancy is the ability to diversify gene function over time. In other words, extra copies of genes that are not required for normal organism function might end up being used in new and entirely different ways, leading to new opportunities in evolutionary selection (Adams & Wendel, 2005).
Interestingly, polyploidy can affect sexuality in ways that provide selective advantages. One way is by disrupting certain self-incompatibility systems, thereby allowing self-fertilization. This might be the result of the interactions between parental genomes in allopolyploids (Comai et al., 2000). Another way is by favoring the onset of asexual reproduction, which is associated with polyploidy in both plants and animals. This switch in reproductive strategies may improve fitness in static environments.
Disadvantages of Polyploidy
For all the advantages that polyploidy can confer to an organism, there are also a great number of disadvantages, both observed and hypothesized. One of these disadvantages relates to the relative changes between the size of the genome and the volume of the cell. Cell volume is proportional to the amount of DNA in the cell nucleus. For example, doubling a cell's genome is expected to double the volume of space occupied by the chromosomes in the nucleus, but it causes only a 1.6-fold increase in the surface area of the nuclear envelope (Melaragno et al., 1993). This can disrupt the balance of factors that normally mediate interactions between the chromosomes and nuclear components, including envelope-bound proteins. The peripheral positioning of telomeric and centromeric heterochromatin may be disturbed as well, because there is less relative surface space on the nuclear envelope to accommodate this positioning (Fransz et al., 2002).
Polyploidy can also be problematic for the normal completion of mitosis and meiosis. For one, polyploidy increases the occurrence of spindle irregularities, which can lead to the chaotic segregation of chromatids and to the production of aneuploid cells in animals and yeast. Aneuploid cells, which have abnormal numbers of chromosomes, are more readily produced in meioses involving three or more sets of chromosomes than in diploid cells. Autopolyploids have the potential to form multiple arrangements of homologous chromosomes at meiotic metaphase I (Figure 2), which can result in abnormal segregation patterns, such as 3:1 or 2:1 plus one laggard. (Laggard chromosomes do not attach properly to the spindle apparatus and thus randomly segregate to daughter cells.) These abnormal segregation patterns cannot be resolved into balanced products, and random segregation of multiple chromosome types produces mostly aneuploid gametes (Figure 3). Chromosome pairing at meiosis I is more constrained in allopolyploids than in autopolyploids, but the stable maintenance of the two parental chromosomal complements also requires the formation of balanced gametes.
Another disadvantage of polyploidy includes potential changes in gene expression. It is generally assumed that an increase in the copy number of all chromosomes would affect all genes equally and should result in a uniform increase in gene expression. Possible exceptions would include genes that respond to regulating factors that do not change proportionally with ploidy. We now have experimental evidence for such exceptions in several systems. In one interesting example, investigators compared the mRNA levels per genome for 18 genes in 1X, 2X, 3X, and 4X maize. While expression of most genes increased with ploidy, some genes demonstrated unexpected deviations from expected expression levels. For example, sucrose synthase showed the expected proportional expression in 2X and 4X tissues, but its expression was three and six times higher, respectively, in 1X and 3X tissues. Two other genes showed similar, if less extreme, trends. Altogether, about 10% of these genes demonstrated sensitivity to odd-numbered ploidy (Guo et al., 1996).
Epigenetic instability can pose yet another challenge for polyploids. Epigenetics refers to changes in phenotype and gene expression that are not caused by changes in DNA sequence. According to the genomic shock hypothesis, disturbances in the genome, such as polyploidization, may lead to widespread changes in epigenetic regulation. Although there are few instances of documented epigenetic instability in autopolyploids, there are a couple of intriguing examples worth mentioning. In one case, transgene silencing occurred more frequently in Arabidopsis thaliana tetraploids than in A. thaliana diploids, suggesting an effect of ploidy on chromosome remodeling (Mittelsten Scheid et al., 1996). However, several factors cannot be ruled out in the observation of this phenomenon, including duplication of the strong 35S promoter from cauliflower mosaic virus in the transgene. In another case, the activation of a DNA transposon of the Spm/CACTA family was observed in autopolyploids. Unfortunately, the generality of this change could not be determined because multiple independent autopolyploids were not examined.
Conversely, extensive evidence for epigenetic remodeling is available in allopolyploids. Structural genomic changes, such as DNA methylation, and expression changes are reported to accompany the transition to alloploidy in several plant systems, including Arabidopsis and wheat (Shaked et al., 2001). The most detailed information is available for the model system Arabidopsis. For instance, in a cross of A. thaliana and A. arenosa, epigenetically regulated genes were identified by comparing transcripts from the autotetraploid parents to transcripts from the neoallopolyploid progeny. A. thaliana genes affected by epigenetic regulation were defined as those that responded to the transition from autopolyploidy to allopolyploidy. Altogether, between 2% and 2.5% of A. thaliana genes were estimated to have undergone regulatory changes during the transition to allopolyploidy. A more detailed microarray study that examined the regulation of 26,000 genes in Arabidopsis neoallopolyploids detected a transcriptome divergence between the progenitors of more than 15%, due to genes that were highly expressed in A. thaliana and not in A. arenosa or vice versa. Significantly, expression of approximately 5% of the genes diverged from the mid-parent value in two independently derived allotetraploids, consistent with nonadditive gene regulation after hybridization (Wang et al., 2006). Taken together, these results suggest that the instability syndrome of neoallopolyploids may be attributed primarily to regulatory divergence between the parental species, leading to genomic incompatibilities in the allopolyploid offspring.
Aneuploidy might also be a factor in epigenetic remodeling in neoallopolyploids, either by altering the dosage of factors that are encoded by chromosomes that have greater or fewer than the expected number of copies leading to changes in imprinted loci, or by exposing unpaired chromatin regions to epigenetic remodeling mechanisms. In the latter case, this susceptibility of meiotically unpaired DNA to silencing was first reported for the fungus Neurospora crassa, but it appears to be a general phenomenon. Therefore, some of the epigenetic instability that is observed in allopolyploids might result from aneuploidy.
Evolutionary Potential of Polyploid Organisms
At first sight, the epigenetic changes observed in polyploids would seem to be deleterious because of their disruptive effects on regulatory patterns established by selection. However, these epigenetic changes might instead increase diversity and plasticity by allowing for rapid adaptation in polyploids. One example may be the widespread dispersal of the invasive allopolyploid Spartina angelica. However, it is not clear whether the success of this species can be attributed to fixed heterosis or to the increased variability that results from epigenetic remodeling. Polyploidy is also believed to play a role in the rapid adaptation of some allopolyploid arctic flora, probably because their genomes confer hybrid vigor and buffer against the effects of inbreeding. However, fertility barriers between species often need to be overcome in order to form successful allopolyploids, and these barriers may have an epigenetic basis.
Summary
Recent studies have provided interesting insights into the regulatory and genomic consequences of polyploidy. Together with the emerging evidence of ancestral duplication through polyploidization in model plant, fungus, and animal species, knowledge of these consequences has stimulated thinking about the relationship between early polyploidization events, the success of the polyploidy, and the long-term fate of new species.
References and Recommended Reading
This article was adapted from Comai, L., The advantages and disadvantages of being polyploid. Nature Reviews Genetics 6, 838-845 (2005) (link to article)
Adams, K. L., & Wendel, J. F. Polyploidy and genome evolution in plants. Current Opinion in Plant Biology 8, 135-141 (2005)
Comai, L. et al. Phenotypic instability and rapid gene silencing in newly formed Arabidopsis allotetraploids. Plant Cell 12, 1551-1568 (2000)
Fransz, P., et al. Interphase chromosomes in Arabidopsis are organized as well defined chromocenters from which euchromatin loops emanate. Proceedings of the National Academy of Sciences 99, 14584-14589 (2002)
Guo, M., Davis, D., & Birchler, J. A. Dosage effects on gene expression in a maize ploidy series. Genetics 142, 1349-1355 (1996)
Melaragno, J. E., Mehrotra, B., & Coleman, A. W. Relationship between endopolyploidy and cell size in epidermal tissue of Arabidopsis. Plant Cell 5, 1661-1668 (1993)
Mittelsten Scheid, O., et al. A change of ploidy can modify epigenetic silencing. Proceedings of the National Academy of Sciences 93, 7114-7119 (1996)
Shaked, H., et al. Sequence elimination and cytosine methylation are rapid and reproducible responses of the genome to wide hybridization and allopolyploidy in wheat. Plant Cell 13, 1749-1759 (2001)
Van de Peer, Y., & Meyer, A. Chapter 6: Large-scale gene and ancient genome duplications. In The Evolution of the Genome, ed. T. R. Gregory, 330-363 (San Diego, Elsevier, 2005)
Wang, J., et al. Genome-wide nonadditive gene regulation in Arabidopsis allotetraploids. Genetics 172, 507-517 (2006)