« Prev Next »
The first systematic studies of whether human behaviors are hereditary were conducted over 100 years ago by Sir Francis Galton, Charles Darwin's cousin. Galton was interested in determining whether human abilities, such as intelligence, could be inherited, and he realized that in order to do this, he must distinguish between those behaviors that were innate and those that were influenced by the environment. To make this distinction, Galton studied the behavioral characteristics of genetically identical twins who were raised in different environments. If twins who had similar traits at birth became dissimilar when reared under differing conditions, these results would support the idea that environment was the primary influence on human behavior. Using this method, Galton deduced that nature (genetics) rather than nurture (environment) played a significant role in behavior (Galton, 1874).
Galton's twin studies and other research involving inherited abilities made him a pioneer in the field of behavioral genetics. Today, modern behavioral geneticists have the same basic goal as Galton: to understand the genetic and environmental contributions to individual variations in behavior. However, current theories support the role of both genes and environment in behavioral outcomes (Robinson, 2004).
Can Single Genes Determine Our Actions?
Many people know about single-gene disorders, but few people know that these conditions are actually far less common than the numerous disorders and nondisease phenotypes that result from the intricate interplay between multiple genes and the environment. Furthermore, even with single-gene disorders (in which a mutation in just one gene manifests as disease), it is often the case that modifier genes at other loci contribute to the severity of the disease phenotype. Thus, sensational reports suggesting that scientists have found the individual gene responsible for a particular behavioral trait (be it alcoholism, sexual orientation, or another complex phenotype) are misleading. In reality, specific animal behaviors—including those of humans—are the result of multiple genes interacting with numerous environmental factors. Awareness of this complexity has shifted researchers' focus from individual genes to entire genomes, thus giving rise to the field of behavioral genomics.
From Behavioral Genetics to Behavioral Genomics
Twin studies are still a prevalent way to research potentially heritable human behaviors, but today, scientists use these studies in the attempt to identify specific genes that can be linked to behavioral phenotypes. This approach has been facilitated by traditional genetic and molecular techniques (e.g., linkage mapping), as well as by newer methods of whole-genome association. Use of model organisms (e.g., fruit flies) has also allowed investigators to directly manipulate and quantify gene expression and observe its effects on behavior.
The Candidate Gene Approach
Traditionally, research efforts involving the potential impact of genes on inherited disorders have been advanced using the "candidate gene" approach, in which investigators ask whether a specific allele is more common in subjects who display a particular trait or behavior than in those subjects who do not (Fitzpatrick et al., 2005). For a gene to be considered as affecting behavior, some a priori knowledge is usually required, including either pathological mechanisms (in the case of disorders) or how a specific gene affects similar behaviors in other organisms. The latter information can sometimes be obtained from one or more of the numerous databases detailing the genomes of a variety of species, including humans, mice, chickens, fruit flies, honeybees, worms, and zebrafish.
After a gene is selected as a "candidate," its expression pattern (most often in the brain) in subjects who display the behavior of interest is compared to that in subjects who do not display the behavior. This comparison can be performed under natural conditions (i.e., without manipulation) in order to gather correlative data regarding the gene and the behavior. However, a more causal link can be established if expression of the gene is manipulated via transgenic or knock-in/knock-out techniques and the resulting behavior is observed. Indeed, these techniques have been essential in determining the genes associated with adaptive behaviors that are evolutionarily conserved in diverse species (e.g., foraging and reproduction), and they have also helped pinpoint genes associated with neurobehavioral disorders like autism.
Large-Scale Approaches
Unfortunately, a candidate-gene-by-candidate-gene approach is often inefficient. Thus, investigators are increasingly turning to the enormous amount of data available through the genome sequencing of many organisms. Use of this information allows researchers to adopt a more unbiased approach, simultaneously examining up to thousands of genes at once. Genomic tools also allow scientists to associate genetic variants, including single nucleotide polymorphisms (SNPs), with specific behaviors or even with different gene expression patterns using microarray comparisons. Often, the genes identified through these large-scale genomic approaches are designated as candidate genes, and the effects of these candidates are then verified through more traditional approaches wherein individual genes and their functions are examined.

Candidate Genes for Adaptive Behaviors
As previously mentioned, various techniques have been used to successfully identify genes associated with a number of adaptive behaviors. Such behaviors include courtship and mating, foraging, and social interaction, all of which have been evolutionarily conserved.
Courtship and Mating
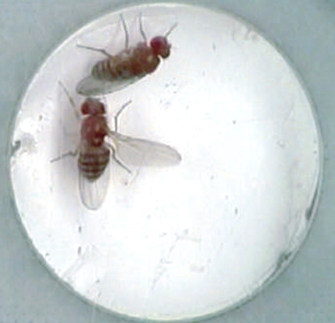

Male courtship in the fruit fly Drosophila melanogaster was one of the first behaviors for which researchers found strong evidence for specification by a gene—namely, the so-called "fruitless" gene (abbreviated fru). In male fruit flies, courtship is a complex, ritualistic behavior that involves many visual, olfactory, gustatory, tactile, and acoustic cues, as well as intricate motor output directed toward attracting a suitable mate (i.e., a female that has not recently mated). During courtship, the male fruit fly orients to the female, taps her with his forelegs, sings a courtship-specific song by vibrating one wing, probes the female's genitalia, and then curves his abdomen to mate (Figure 1; Hall, 1994).
The fruitless gene first emerged as a candidate gene for sexual behavior when it was discovered that male flies with fru mutations were sterile due to defective performance of courtship and copulation (Gill, 1963). Years later, the mutated gene present in these flies was cloned, and the protein that it encodes was identified as a transcription factor (Ito et al., 1996; Ryner et al., 1996). Researchers have also determined that the fru gene is spliced differentially in males and females and that this gene generates various sex-specific messenger RNAs (Demir & Dickson, 2005; Kyriacou, 2005). Interestingly, not only does the male pattern of splicing elicit male courtship behavior, but it also influences sexual orientation, as evidenced by the finding that male splicing in otherwise normal females generated male behavior (e.g., singing) that was directed toward other females (Figure 2; Demir & Dickson, 2005). Functional conservation of the fru gene has only been shown in one other species, the malaria mosquito Anopheles gambiae (Gailey et al., 2006), and a mammalian homologue for fru does not exist. Even so, fru is a good example of how genes can specify aspects of a complex inborn behavior.
Foraging
Once the for gene was associated with foraging behavior in fruit flies, it was used as a candidate gene to study similar behaviors in other species. For example, the fruit fly for gene shows high sequence homology to the Amfor gene in honeybees. Changing Amfor expression can alter the foraging behavior of a honeybee, thereby transforming the bee from a "nurse" (a bee that cares for the young) to a "forager" (a bee that leaves the hive to find pollen and nectar). Like the rover fruit fly, the forager honeybee has higher levels of the PKG enzyme than do nurse bees (Ben-Shahar et al., 2002). Researchers have also noted that an orthologue of for, called egl-4, is associated with food-related behavior in the roundworm C. elegans (Fujiwara et al., 2002). Taken together, these findings suggest that for could be used as a candidate gene for behaviors concerning food acquisition in other species.
Social Interaction
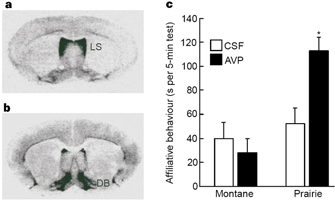

Researchers began their inquiry into these genes using voles, which are small rodents that look like mice, because there are two particular vole species that differ greatly in terms of social behavior. Specifically, prairie voles (Microtus ochrogaster) are affiliative and monogamous (i.e., each vole has only one mate), and both parents provide care for their young, whereas montane voles (Microtus montanus) are comparatively asocial, polygamous (i.e., each vole has multiple mates), and nonpaternal. In male prairie voles, living and/or mating with a female causes AVP release in the brain, and paternal and pair-bonding behavior is triggered. However, these behaviors can be disrupted by blocking the V1a receptor for AVP with an antagonist. Thus, comparing AVP receptor expression between these two vole species was proposed as a useful way to determine which genes contribute to social behavior.
Indeed, prairie and montane voles have markedly different patterns of binding to the V1a receptors within their brains (Figure 3; Young et al., 1999). The polygamous montane vole has much more binding in the lateral septum of the brain than the prairie vole, whereas the monogamous prairie vole has a higher intensity of binding in the diagonal band of Broca, a brain area involved in appetitive reward (Young et al., 1999; Insel & Young, 2000). Furthermore, elevating the expression of the V1a receptor in the brains of promiscuous voles increased the pair-bonding behavior of males to females (Lim et al., 2004). Thus, the social behavior of these two species of voles seems to be due to variations in gene expression of the V1a receptor.
Candidate Genes for Human Disorders
Neurobehavioral disorders are debilitating psychiatric conditions that include depression, bipolar disorder, schizophrenia, and autism. Currently, diagnosis of these diseases is based primarily on clinical symptoms that vary immensely depending on the individual. This phenotypic variability makes effective treatment paradigms difficult to establish. For this reason, scientists have been searching for candidate genes that may influence the onset of these neuropsychiatric pathologies (Glatt & Freimer, 2002). Identifying these candidate genes is difficult, because there are often no clear biomarkers associated with a particular disease against which predictions about the effects of a gene could be tested. Despite such limitations, researchers have associated numerous genes with some neurobehavioral disorders, including autism and schizophrenia. In addition, microarray technology and new SNP-based, whole-genome association methods have helped combat these difficulties to some extent (Glatt & Freimer, 2002; Sutcliffe, 2008).
Autism
Autism is a severe neurobehavioral disorder characterized by impaired social skills and repetitive and stereotyped interests and behavior. Autism includes mental retardation in up to 70% of cases (Fombonne, 2003), and it affects many brain areas. Twin studies and examinations of family history suggest that the heritability of autism is roughly 90% (Freitag, 2007; Sutcliffe, 2008). Genetic aspects of the disorder have been substantiated by the identification of chromosomal translocations, inversions, and large deletions or duplications, which are more prevalent in individuals who have more severe cognitive problems. For example, several recent large-scale studies collectively screened almost 2,000 families with autism for recurrent copy number variations, which are small losses or gains in DNA. The consortium of researchers involved in these studies found that copy number variation in a gene called neurexin is linked to the presence of autism. The researchers also found a region on chromosome 11 that might contain sequences that are involved in the disorder (Autism Genome Project Consortium, 2007). Similarly, Weiss and colleagues (2008) found that a microdeletion and microduplication on chromosome 16p11.2 increased susceptibility for the disorder in 1% of cases. Other studies using DNA microarrays have identified many more copy number variations that can be sporadic or inherited and appear to be major genetic risk factors for autism in 10% to 20% of cases (Morrow et al., 2008; Sutcliffe, 2008).
Table 1 summarizes the genes that have been implicated in autism thus far (Sutcliffe, 2008). Although these genes are diverse, most researchers suggest that they may be linked by a common mechanism, namely dysregulation of gene expression that occurs after neural activity (Morrow et al., 2008). It is also evident that studying all of these genes, as well as the genes that they regulate and are regulated by, requires the large-scale analysis techniques employed in genomics.
Table 1: Putative and Known Autism-Related Genes
Genes Involved in Glutamatergic Synapse Function and/or Neuronal Cell Adhesion | |
FMR1A,B | Fragile X mental retardation 1 |
NLGN3B | Neuroligin 3 |
NLGN4B | Neuroligin 4 |
NRXN1B,C | Neurexin 1 |
SHANK3B,C | SH3 and multiple ankyrin repeat domains 3 |
CNTNAP2B,C,D | Contactin-associated protein-like 2 |
PCDH10C | Protocadherin 10 |
CNTN3C | Contactin 3 |
Genes Involved in Endosomal Trafficking | |
NHE9 (SLC9A9)B,C | Na+/H+ exchanger isoform 9 |
NHE6 (SLC9A6)B | Na+/H+ exchanger isoform 6 |
DIA1 (c3orf58)C | Deleted in autism 1 |
A2BP1C | Ataxin 2-binding protein 1 |
Genes Involved in Neuronal Activity Regulation | |
FMR1A,B | Fragile X mental retardation 1 |
MECP2B,C | Methyl CpG binding protein 2 |
DIA1 (c3orf58)C | Deleted in autism 1 |
PCDH10C | Protocadherin 10 |
NHE9 (SLC9A9)B,C | Na+/H+ exchanger isoform 9 |
A2BP1C | Ataxin 2-binding protein 1 |
UBE3AB,C | Ubiquitin protein ligase E3A |
Genes Implicated in Related Disorders | |
FMR1A,B | Fragile X mental retardation 1 |
MECP2B,C | Methyl CpG binding protein 2 |
NHE6 (SLC9A6)C | Na+/H+ exchanger isoform 6 |
A2BP1C | Ataxin 2-binding protein 1 |
UBE3AB,C | Ubiquitin protein ligase E3A |
Genes With Other Functions | |
EN2D | Engrailed homeobox 2 |
SLC6A4B,D | Serotonin transporter (SERT, 5-HTT) |
METD | Met proto-oncogene (c-Met, HGFR) |
SCN7AC | Na+ channel, voltage-gated, type VII |
RNF8C | Ring finger protein 8 |
Table adapted from Sutcliffe, 2008
Schizophrenia
Schizophrenia is a neuropsychiatric illness in which individuals have impaired perceptions of reality (e.g., hallucinations, delusions, and disorganized thoughts). As with autism, genetic implications for this disorder arose from twin studies. For instance, such studies show that 45% of identical twins who are adopted by different families will both be schizophrenic. Furthermore, if one sibling within a family has been diagnosed with schizophrenia, the other siblings have a 10-time greater chance of also developing the disorder (Plomin et al., 1994).
Identifying the genetic components of schizophrenia is complicated by the difficulties associated with diagnosing the disease itself, as different doctors often use different criteria to make the same diagnosis. Thus, when they perform studies of schizophrenia, investigators must be particularly careful to look at large populations of individuals with and without the illness, to ensure that all subjects have been diagnosed using the same criteria.
Recently, about 15% of patients with schizophrenia were found to have mutations in a large set of genes, many of which are important for brain development (Walsh et al., 2008). Disturbances have also been found in the expression of genes related to synaptic function, energy metabolism, and oligodendrocyte function (Bray, 2008). These findings implicate hundreds of potential gene candidates and suggest that each person with the disorder could have a different genetic cause. Thus, despite the power of whole-genome analysis to link diseases to genes, considerable work is often required to separate the "wheat" from the "chaff" in such data sets.
Genetic Components of Human Behavior and Disease
The genetic components of the behaviors and disorders described in the previous sections are by no means comprehensive. Moreover, genes have also been linked to many other diverse traits and diseases, including addiction (e.g., alcohol and tobacco), handedness, bipolar disorder, depression, and dyslexia. With genome sequencing costs plummeting and new discoveries about the genes and environmental factors that affect behavior being made each day, it seems certain that in the years to come, we will develop an even better understanding of why we behave the way we do.
References and Recommended Reading
Autism Genome Project Consortium. Mapping autism risk loci using genetic linkage and chromosomal rearrangements. Nature Genetics 39, 319-328 (2007) doi:10.1038/ng1985 (link to article)
Ben-Shahar, Y., et al. Influence of gene action across different time scales on behavior. Science 296, 741-744 (2002)
Bray, N. J. Gene expression in the etiology of schizophrenia. Schizophrenia Bulletin 34, 412-418 (2008)
de Belle, J. S., & Sokolowski, M. B. Heredity of rover/sitter: Alternative foraging strategies of Drosophila melanogaster. Heredity 59, 73-83 (1987)
Demir, E., & Dickson, B. J. Fruitless splicing specifies male courtship behavior in Drosophila. Cell 125, 785-794 (2005) doi:10.1016/j.cell.2005.04.027
Fitzpatrick, M.J., et al. Candidate genes for behavioural ecology. Trends in Ecology and Evolution 20, 96-104 (2005)
Fombonne, E. Epidemiological surveys of autism and other pervasive developmental disorders: An update. Journal of Autism and Developmental Disorders 33, 365 (2003)
Freitag, C. M. The genetics of autistic disorders and its clinical relevance: A review of the literature. Molecular Psychiatry 12, 2-22 (2007) doi:10.1038/sj.mp.4001896
Fujiwara, M., et al. Regulation of body size and behavioural state in C. elegans by sensory perception and the EGL-4 cGMP-dependent protein kinase. Neuron 36, 1091-1102 (2002)
Gailey, D. A., et al. Functional conservation of the fruitless male sex-determination gene across 250 myr of insect evolution. Molecular Biology and Evolution 23, 633-643 (2006) doi:10.1093/molbev/msj070
Galton, F. English Men of Science: Their Nature and Nurture (London, Macmillan, 1874)
Gill, K. S. A mutation causing abnormal courtship and mating behavior in males of Drosophila melanogaster. American Zoologist 3, 507 (1963)
Glatt, C. E., & Freimer, N. B. Association analysis of candidate genes for neuropsychiatric disease: The perpetual campaign. Trends in Genetics 18, 307-312 (2002) doi:10.1016/S0168-9525(02)02670-7
Hall, J. C. The mating of a fly. Science 264, 1702-1714 (1994)
Insel, T. R., & Young, L. J. Neuropeptides and the evolution of social behavior. Current Opinion in Neurobiology 10, 784-789 (2000)
Ito, H., et al. Sexual orientation in Drosophila is altered by the satori mutation in the sex-determination gene fruitless that encodes a zinc finger protein with a BTB domain. Proceedings of the National Academy of Sciences 93, 9687-9692 (1996)
Kyriacou, C. P. Behavioural genetics: Sex in fruit flies is fruitless. Nature 436, 334-335 (2005) doi:10.1038/436334a (link to article)
Lim, M. M., et al. Enhanced partner preference in a promiscuous species by manipulating the expression of a single gene. Nature 429, 754-757 (2004) doi:10.1038/nature02539 (link to article)
Morrow, E. M., et al. Identifying autism loci and genes by tracing recent shared ancestry. Science 321, 218-223 (2008)
Osborne, K. A., et al. Natural behaviour polymorphism due to a cGMP-dependent protein kinase of Drosophila. Science 277, 834-836 (1997)
Plomin, R., et al. The genetic basis of complex human behaviors. Science 264, 1733-1739 (1994)
Robinson, G. E. Genomics: Beyond nature and nurture. Science 304, 397-399 (2004) doi:10.1126/science.1095766
Ryner, L. C., et al. Control of male sexual behavior and sexual orientation in Drosophila by the fruitless gene. Cell 87, 1079-1089 (1996)
Sokolowski, M. B. Drosophila: Genetics meets behaviour. Nature Reviews Genetics 2, 879-890 (2001) doi:10.1038/35098592 (link to article)
Sokolowski, M. B., et al. Evolution of foraging behaviour in Drosophila by density dependent selection. Proceedings of the National Academy of Sciences 94, 7373-7377 (1997)
Sutcliffe, J. S. Insights into the pathogenesis of autism. Science 321, 208-209 (2008) doi:10.1126/science.1160555
Walsh, T., et al. Rare structural variants disrupt multiple genes in neurodevelopmental pathways in schizophrenia. Science 320, 539-543 (2008)
Weiss, L. A., et al. Association between microdeletion and microduplication at 16p11.2 and autism. New England Journal of Medicine 358, 667-675 (2008) doi:10.1056/NEJMoa075974
Young, L. J., et al. Increased affiliative response to vasopressin in mice expressing the V1a receptor from a monogamous vole. Nature 400, 766-768 (1999) doi:10.1038/23475 (link to article)