Abstract
Β-asarone is a phenylpropane derivative present in the rhizomes of Acorus calamus, that was proved to exhibit toxic effects in humans. Because of its presence the whole plant that is commonly used in traditional medicine for its sedative, anti-inflammatory, neuroprotective and other properties has limited application nowadays. In the study, qualitative and quantitative analysis of a collection of nine essential oil (EO) samples of European and Asian origin was performed. The final content of β-asarone in the tested samples ranged between 0.265 and 1.885 mg/mL. Having in mind a possible application of the EO as a biopesticide, this research aimed at the development of CPC-based purification protocol that could help remove β-asarone from EO. It was proved that the biphasic solvent system composed of n-hexane/EtOAc/MeOH/water, 9:1:9:1 (v/v/v/v) was capable of the removal of the toxic constituent in the CPC chromatograph operated in the ascending elution mode with 2200 rpm and a flow rate of 5 mL/min. The chromatographic analysis that lasted only 144 min effectively separated β-asarone (purity of 95.5%) and α-asarone (purity of 93.7%) directly from the crude Acorus calamus rhizome EO.
Similar content being viewed by others
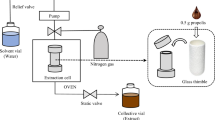
Introduction
Maintaining high quality crop products parallelly with reduced synthetic pesticides content is the biggest challenge for the modern agriculture. The toxic pesticide residues accumulate in crop yields, soil and ground waters what triggers the development of pests’ and weeds’ resistance, but simultaneously, affects soil microbiome and well-being of people and animals. However, the plant derived products of the secondary metabolism which include, inter alia, volatile components/ essential oils (EO) usually recovered from plant matrix by hydrodistillation seem to be a promising alternative for the synthetic pesticides used so far. Additionally, the EOs were proved to be efficient both in the prevention and treatment of different diseases1. Formerly their antibacterial, antifungal and insecticidal properties were discussed in the scientific literature2. Furthermore, their strong allelopathic properties such as the influence on germination and seedling growth should not be underestimated. The richness and variety of EOs components affects their diversified mechanisms of action and consequently hampers the resistance development in pathogens, pests, and weeds towards the oils. In the end, the effortless biodegradability of EO does not pose a risk to the environment.
The EO that exhibits dualistic, and thus intriguing properties is the calamus oil. It is extracted from rhizomes of sweet flag (Acorus calamus L.) belonging to Araceae botanical family. The leading constituents of calamus EO are phenylpropane derivatives (biosynthesized via the shikimate pathway), including propenylic isomers: a mixture of trans form of α-asarone, cis form of β-asarone ((E)-/(Z)-1,2,4-trimethoxy-5-prop-1-enylbenzene) and an allylic derivative γ-asarone (1,2,4-trimethoxy-5-prop-2-enylbenzene) (see Fig. 1). Methyleugenol, (Z)-methylisoeugenol, and elemicin are the remaining phenylpropenes detected in the calamus oil. The second group of EO constituents are terpenes: monoterpenes (α- and β-pinene, camphene, myrcene, limonene) and sesquiterpenes (cadinene, acorone, acorenone, calamine). Also, the presence of tannins, choline, and fatty acids in its rhizomes was previously described3,4,5,6.
The amount of phenylpropenes varies depending on the ploidy of A. calamus variant. In North America and in parts of Asia (Siberia) a diploid form (2n = 2x = 24) of the sweet flag may be found, which was proved to contain only 2% of EOs with no or trace amounts of β-asarone in its composition. A. calamus plants growing in Europe and temperate regions of Asia are primarily triploids (2n = 3x = 36) containing more EOs (3%) and little amounts of β-asarone (5–19%) in the EO. The third, tetraploid variety (2n = 4x = 48) that is most commonly used for the industrial production of calamus oil is widespread in East Asia, Japan and India and contains up to 6% of EOs and the largest amount of β-asarone (70–96%) in the EO from the rhizomes7,8.
For many years sweet flag extracts remained substances of interest due to their their medicinal properties. Calamus preparations exhibit neuroprotective, antidepressant, anticonvulsive, sedative, hypnotic, anxiolytic, memory enhancing, antiasthmatic, antihyperlipidemic, anticholestatic, antithrombotic, antiinflammatory, antioxidant, anticarcinogenic, antimicrobial and insecticidal properties9,10,11,12. Even if the traditional usage of the plant in medicine, fragrance industry and foods has been wide, the exact modus operandi of calamus phytochemicals is still unexplored. Also, the contradictory reports on the toxicological safety of calamus oil for humans still need a final conclusion. Some authors mention cardiotoxicity, hepatotoxicity, reproductive toxicity, mutagenicity and carcinogenicity of β-asarone7. Based on the reported adverse effects, legal regulations for the use of β-asarone have been established. Within the European Union, the use of β-asarone as a pure substance or in the form of the tetraploid calamus essential oil for flavoring purposes is currently prohibited. In the United States of America, the use of calamus oils and its preparations is utterly disallowed. Several scientific reports on the therapeutic application of β-asarone, like memory enhancing properties13 or antimicrobial action14 re questionable in the light of the regulations described above. The observed tendency in the number of scientific manuscripts in the Scopus database shows a decrease in the number of articles concerning Acorus calamus, while the number of scientific papers on β-asarone itself stays on the same level.
Despite the toxicological data presented above, calamus EO still bears potential to be used as a biopesticide15. It was proved that calamus oil contains a number of important active substances (other than β-asarone) with a synergistic effect when in mixture16,17,18,19. In the light of these findings, a good solution, safe for farmers and customers, might be to develop a method of effective removal of β-asarone from calamus oil aspiring to the role of a biopesticide—to be able to use it with no risk for poisoning of humans.
Classical isolation methods used to separate EOs components, like HPLC or TLC chromatography, even if they are offering several advantages like the reproducibility of results, automation, quantitative determination, short analysis time, they are not suitable for the analysis of all secondary metabolites or in the purification on semi- or preparative scale20. One should mention here an irreversible adsorption on the chromatographic bed, which results in the sample loss and the damage of expensive preparative columns, tailing of compounds of interest on the stationary phase which decreases the purity of the isolates, or small loading capacities which limit the application of these techniques to the industrial scale isolation protocols. Also, the high consumption of solvents makes the classical separation process unprofitable and failing the requirements of green chemistry practice.
In the scientific literature there are only a few examples of β-asarone isolation. Previously, McGaw and co-investigators introduced a two-step fractionation (column chromatography and preparative thin layer chromatography) of the crude ethanol extract from Acorus calamus roots, rhizomes and leaves. In the proposed protocol they obtained 15.6 mg of the component from 1.14 g of extract14. In another work the same compound was purified from the rhizome volatile oil with chloroform: petroleum ether (4:1 v/v) using a silica gel chromatographic column13. The two mentioned above techniques were based on the application of classical chromatographic tools in the recovery of β-asarone and are bearing several limitations. The recovery rate and the loading capacity of these protocols was low, whereas the procedure tedious and the selectivity poor. Both techniques needed high volumes of solvents to be run, were time consuming, and the cited sources did not provide any information about the purity of the isolate. Based on these observations the authors of this manuscript found it important to search for a new isolation protocol that would provide high purity β-asarone directly from the crude EO sample to be either removed or isolated from EO. Easy scale-up, low solvents consumption and short analysis time would be preferred for future application in biopesticide production as well as in other calamus preparations.
Centrifugal Partition Chromatography (CPC) is a technique that is devoid of these drawbacks. This modern separation tool is the hydrostatic type of countercurrent chromatography, which uses the phenomenon of liquid–liquid partitioning of compounds between two non-miscible phases that stay at equilibrium21. One phase (stationary one) is retained in the column, while the other one (mobile phase) is pumped through it. Both immiscible liquids are in constant contact, emulsifying and separating repeatedly, creating the phenomenon of theoretical plates. The retention mechanism of stationary phase is determined by hydrostatic force, formed by the centrifugal field in the rotor in one-axis centrifuge22,23. The separation of constituents of a mixture is obtained as a function of partition coefficient Kd, defined as the concentration of target compound in the stationary phase divided by the concentration in the mobile phase24.
CPC is able to provide repetitive and selective separation conditions for the purification of single metabolites from plant extracts—also on preparative scale. High selectivity of applied methods, low solvent consumption, high sample loading capacity and lack of solid support that prevents sample loss is conducive to recover sufficient quantities of natural products even from complex plant extracts25,26. However, the scientific literature on the analysis of essential oils by counter current chromatography is limited. Interestingly, according to our knowledge only four scientific works are focusing on the separation of essential oils using Centrifugal Partition Chromatography were traceable27,28,29,30, while the remaining majority concerned the HSCCC technique. At this point it is worth mentioning that the CPC technique creates more advantageous conditions for a large-scale separations than the HSCCC technique.
Therefore the aim of this study is to develop precise single-step methodological conditions that would provide calamus oil free from β-asarone to be used on industrial scale. For this purpose CPC chromatography will be used to give hope for the successful exclusion of this potentially toxic phenylpropane derivative and possible application of the β-asarone-free EO as biopesticide in the future. Also, the determination of β-asarone content in the personally collected and commercially available EO samples will be performed with help of the HPLC-DAD and HPLC-ESI-QTOF-MS/MS techniques to confirm the actual content of the terpene of interest in the European and Asian samples and to emphasize the need for its removal.
Results and discussion
Essential oil composition by HPLC-ESI-QTOF-MS/MS
The performed HPLC-ES-QTOF-MS/MS analysis of the EO samples led to the identification of 22 compounds out of ca. 40 signals present in the mass chromatogram. The applied instrument was sensitive enough to show clear mass spectra and sharp peaks of unpolar constituents of Acorus calamus rhizomes using a standard RP-18 chromatographic column. The composition of a sample EO and the list of tentatively identified components that was prepared in consideration with literature results on different Acorus spp. are presented in the Fig. 2, in Table 1 and also Table S1 (Supplementary File).
Among the representatives of different classes of compounds, asarones belonged to the most abundant components of the tested essential oils, with β-asarone as the leading compound. The identified constituents—terpenes and other asarone-related phenylpropanoids, were previously mentioned by other authors in Acorus calamus and Acorus tatarinowii species, as shown in Table 1.
Quantitative analysis of asarone content in EO by HPLC-DAD
The application of a liquid chromatography in the analysis of phenylpropanoids’ content in the EO of A. calamus brought successful results. The HPLC-DAD based quantitative analysis of the asarones visualized the three forms of asarones: α-, β- and γ-asarone. These compounds were eluted from the column at 14.0, 11.5 and 12.8 min, respectively (see Fig. 7A). The quantitative analysis of asarones in the tested samples revealed marked differences between the samples. Considering the relative percentage content, β-asarone was found to be the major phenylpropanoid visible in the HPLC-DAD chromatogram, followed by α-asarone and γ-asarone in all tested samples (see Table 2). Interestingly, the total concentration of the β-asarone differed between the samples (see Fig. 3).
Its content was calculated as 1.32–1.41 mg/mL of the EO in the samples S1 and S2, 0.27 mg/mL in the sample S3, 0.19–0.41 mg/mL in the EOs from the commercially available rhizomes (samples S4-S6) and 1.75–1.89 mg/mL in the commercially available EOs from A. calamus (Samples S7-S9). Interestingly, the content of β-asarone differed depending on the place of collection of the rhizomes. The quantity of this compound in the Sample S3 that was collected in the central Poland in Małczew was significantly lower from the samples S1 and S2 (see Fig. 3). It could be explained by the presence of different ploidy plants on the area of Poland.
The obtained quantitative results for β-asarone in calamus oil are in line with some previously published data. A thorough study on its content in Finnish and Czech samples authored by Dušek et al.39 proved that this phenylpropanoid was present in the oil at the quantity of 9.7–21.3% that resembles the concentration of 0.97–2.13 mg/mL. In general, the samples from Finland contained lower quantities of β-asarone in comparison to the Czech samples, but still its content was higher than in the herein investigated samples from the central Poland.
Also, in the herein presented study the content of β-asarone was high in the commercially available EOs. The concentration of this phenylpropanoid in the samples S7-S9 resembled its content in the samples S1-S2.
The quantitative analysis of β-asarone content in the analysed samples brought to light an interesting issue, namely the geographical occurrence of different ploidy A. calamus plants. Even if Poland was expected to host triploids containing more EOs with small share of β-asarone, as mentioned in the introduction section, the quantitative analysis showed marked quantity of this component in the calamus oil similarly to the, widely distributed in Asia, tetraploid variety. The calculated β-asarone content is close to S9 sample of the Indian origin. Based on the obtained results, we could assume there are different ploidy plants in European grounds.
According to Raal and co-investigators40 β-asarone was also the major constituent of the A. calamus rhizome essential oil that was obtained from the specimen grown in Estonia. The compound of interest constituted 85.3% in one sample—that was identified as a tetraploid sweet flag and 9.3–10.2% in other samples that were possibly triploids. These data confirmed the possibility to find in Europe different ploidy specimen growing in nature. The authors of this publication underlined that their data from 2016 mentioned for the first time the presence of β-asarone-rich chemotype of sweet flag in Europe. For sure, these specimens can also grow on the territory of Poland, as proved in our study.
Chemometric assessment
Since various extracts from Acorus calamus were collected at different dates and using different sources and techniques, for chemometric analysis it was decided to examine the relative concentrations of α-, β-, and γ-asarone in each sample, instead of the absolute values (Table 2).
Principal Component Analysis (PCA) has revealed that the studied dataset was only two-dimensional, since the first two principal components extracted almost 100% (~ 99.99998%) information of the original data matrix. It was revealed that the relative concentration of α-asarone was almost perfectly, yet reversely correlated to the relative concentration of β-asarone, which again confirmed that both compounds have common origin and possibly could be transformed from one to another via enzymatic or light-induced E/Z isomerization. Hence, the information on α- and β-asarone constituted the first dimension of the examined dataset after applied VARIMAX rotation algorithm. The second dimension of the data contained solely information on the relative concentration of γ-asarone, which displayed no correlations to its α- and β- counterparts (Fig. 4).
Figure 4 presents a linear map of samples in the space of the two dimensions established above. Fresh samples collected from northern Poland (S1 & S2) exhibited lower than average relative concentrations of γ-asarone with and medium relative concentrations of α- and β-asarone. The S3 sample, collected from central Poland presented much lower relative amounts of γ-asarone in comparison to the rest of the sources. Samples S4 and S5, coming from commercially available rhizomes, displayed average relative concentrations of all three traced asarones. In contrary, sample S6, while maintaining relative amounts of γ-asarone similar to the ones of S5 and S6, exhibited highest—by far—relative concentration of α-asarone and, therefore, lowest relative concentration of β-asarone. Finally, the commercially available essential oils (S7-S9) presented the highest relative amounts of β- and γ-asarones and the lowest relative amounts of α-asarone in the whole studied group.
Cluster dendrogram (Fig. 5), prepared on the basis of the relative concentrations of all three studied asarones, has furtherly proved that extracts obtained from collected and commercially available rhizomes might be considered similar, with sample S6 being a potential outlier in the group—as PCA has proven, due to significantly different α/β ratio in comparison to samples S1-S5. Nevertheless, in comparison to extracts S1-S6, samples S7-S9, coming from commercially essential oils, exhibited fundamentally different asarone compositions, hence formed a separate cluster on the diagram.
CPC method of removing β-asarone from A. calamus EO
Centrifugal partition chromatography (CPC) as an emerging purification technique is used in the separation process of natural products, protein mixtures, synthetic drugs, APIs and others. It belongs to green techniques due to a limited usage of solvents in the separation process, but most interestingly, it is more commonly selected by the industrial players as a technique reaching industrial-scale solutions41.
CPC chromatographs are used for industrial concentration and purification of several groups of natural products or single compounds, e.g. omega-3 fatty acids42 or acidic cannabinoids from Cannabis sativa43. High selectivity and repeatability of the CPC-based analyses is certainly a strong advantage of this technique, however, its good operation must be based on a proper selection of the biphasic solvent system that should harmonize with the analysed sample and be selective towards the single constituents of the mixture.
Solvent system selection
The selection of the appropriate biphasic system plays a key role in counter-current chromatography, and therefore, this step should commence any method development of the separation process. Herein, an appropriate solvent system was chosen according to the partition and separation factors (Kd and α). Due to the hydrophobicity of target compounds, a series of low-polar solvent systems composed of n-hexane, ethyl acetate, methanol and water in different volume ratios (HEMWat/ Arizona system) were tested to provide an efficient separation of the target compounds. The Arizona system was also selected as there is no need for preparing various biphasic solvent systems in separating funnels (Gilson Glider software combined with a 4-channel pump is able to mix relative portions of the selected solvents directly in the apparatus). This aspect is important in case of pilot and industrial-scale application of developed method as it lowers the consumption of solvents necessary for the separation process. Table 3 summarizes distribution coefficients (Kd) and separation factors (α) calculated for target compounds in the different volume ratios of HEMWat system.
Two of the tested two-phase solvent systems (system 2 and 3) met the first criterion of efficient separation with Kd in the range of 0.5–244. Among the two remaining systems, system 3 and 4 were selected for further evaluation on CPC chromatograph based on their beneficial differences between Kd values of the analysed components.
CPC separation
First, the experiment was run at different rotation speed of the column that was within a range of 1000–2300 rpm, at a constant flow rate of 5 mL/min to evaluate the impact of rotation speed on the selectivity. Finally 2200 rpm setting was selected as the most advantageous one, giving the best selectivity towards asarones with no drastic increase in the operating pressure. The selected relatively high rotation speed kept together with the flow rate of 5 mL/min generated the pressure of 50 bar that is half of the maximum operational pressure in CPC chromatographs. Sustaining a quicker flow rate as 5 mL/min markedly reduced the analysis time. During the optimisation time system No 4 was rejected, as it did not bring better selectivity towards both asarones, but only prolonged the analysis time of 20 min.
As a result of the CPC-based fractionation of Acorus calamus EO using the solvent system composed of n-Hex/EtOAc/MeOH/H2O (9:1:9:1 v/v/v/v), the complete separation of both α- and β- asarones from the essential oil was obtained in a short time of 144 min in the elution mode (see Fig. 6 for the chromatogram from CPC chromatograph). The calculated Sf value of 72% is consistent with the stationary phase retention suggested for counter-current separations45. It should be mentioned, that for all CPC columns, the Sf maximum value is necessarily much lower than 100% with the maximum value reaching 80%. Such values are dictated by the construction of the hydrostatic apparatus46.
The fractions containing asarones were separated, whereas all remaining fractions were collected together. After the completion of the fractionation process the purity check of the isolates was performed using the HPLC-DAD instrumentation, compared with the total EO sample (see Fig. 7) and the purity of asarones was calculated as 93.7% for α- and 95.5% for β- asarone (see Table S4 in the Supplementary File). Interestingly, both compounds were eluted from the column in the elution mode, so as the remaining constituents of the essential oil could be easily pushed out from the column with a higher flow rate of 30 mL/min. This way, the performed fractionation of the EO succeeded in the separation of asarones from the remaining constituents of the oil. Collecting together all fractions except those containing asarones provides EO with no presence of asarones bearing toxic properties.
The elaborated purification protocol is the first one dedicated to the hydrostatic counter-current instrumentation, like CPC chromatographs. Previously, successful trials to recover asarones were performed on a different species, namely on Acorus tatarinowii EO and were published by Wang and co-investigators. The researchers used a different type of chromatograph in their study—an HSCCC chromatograph that is operated in the hydrodynamic mode. The used mixture of n-hexane–ethyl acetate–methanol–water (1:0.2:1:0.3, v/v) fractionated 1.0 g of the essential oil. The analysis was conducted for 6 h to obtain α- and β-asarones in the eluate. The authors used 800 rpm rotation speed of the column and the flow rate of only 1.5 mL/min. The selected parameters had impact on an increased purification time and small sample loading. Even if the previously described purification protocol provided high purity asarones, the application of HSCCC chromatographs is limited to analytical and semi-preparative conditions due to high pressure that is generated during the run.
Asarone-free calamus oil still shows potential as an eco-friendly green biopesticide or as an bioherbicide against weeds as it contains a mixture of many biologically active chemicals. Identified substances such as calarene, shyobunone, safrol, cedrene were detected also in other EOs exhibiting bactericidal, fungicidal, insecticidal, repellent or allelopathic activities, inhibiting germination different species47,48,49,50,51. According to Liu and co-investigators52 α-asarone, methyleugenol and (E)-methylisoeugenol isolated from calamus oil were the strongest fumigants/insecticides and were found toxic against booklouse Liposcelis bostrychophila. In another study Chen et al.47 underlined a strong impact of shyobunone and isoshyobunone isolated from calamus oil on the development of Lasioderma serricorne (LS) and Tribolium castaneum (TC). The isolates were characterized by a strong contact toxicity against LS and TC. The calculated LD50 values against LS adults were 20.24 and 24.19 µg/adult specimen, respectively. The oil and the isolates were found to be strongly repellent already after 2 h of treatment.
The above examples show marked importance of calamus oil other than β-asarone. Based on this observation, the removal of this toxic component from the EO is logic and important especially these days, when new sources of repellent compounds that are neutral to environment are needed. Prior to extensive production, properties of the oil deprived of β-asarone, parallelly with the one containing β-asarone, must be thoroughly evaluated. Their impact on human cell lines, organisms present in the agrocenosis as well as germination and seedling development of various species should be analysed.
Conclusions
Chromatographic techniques are important tools suitable for the evaluation of plant extracts’ composition. They are capable of fingerprinting53 the extracts and also isolating single components from the matrix54. Also in this study the qualitative analysis of the tested EO samples performed by HPLC and HPLC–MS led to the identification of 22 metabolites including β-asarone as a major constituent. According to our study, the content of β-asarone in the European origin EO depends on the location and can resemble the high content of the compound observed in tetraploid specimen from Asia. Having the widespread use of calamus oil in traditional medicine on the one hand, and the data on the toxicity of β-asarone on the other, it is very important to implement a procedure of β-asarone elimination from plant material. The proposed herein technique of removing β-asarone from the rhizome oil of Acorus calamus—the CPC chromatography—gives hope for the potential use of calamus oil as a potential bioherbicide—that could be safe for the environment and humans. Previously published data indicate strong repellent activity of other ingredients than β-asarone present in calamus oil, so its removal will not affect the total biocidal effectiveness. The CPC method proposed in this paper is simple, quick, meets the requirements of green chemistry practice, due to the relative low solvent consumption and promises simple upscaling conditions that would provide high quantities of β-asarone free calamus oil.
Methods
Plant material and extraction procedure
For this study, several samples of Acorus calamus rhizomes—both commercially available and collected from natural stands (see Table 4 and Table 5) were analysed. The material from personal collection came from different regions in Poland (see Fig. 8/Table 5) and was authenticated by prof. Justyna Polit from the Faculty of Biology and Environmental Protection of the University of Łódź in Poland. Also, commercially available calamus oil samples were used for the study. The commercially available A. calamus rhizomes and oil were obtained from the local herbal shop and came from different producers. The collection of the plant material and related studies complies with relevant institutional, national, and international guidelines and legislation.
Map showing locations and origins of Acorus calamus accessions harvested from different regions of Poland. (S1–S3)—sample code, N—North, BY—Belarus, CZ—Czech Republic, DE—Germany, RU—Russian Federation, SK—Slovakia, UA—Ukraine55. The base map of Poland and the neighbouring countries was obtained from http://diva-gis.org/data.
First, the personally collected rhizomes were finely cut and dried in the air in the shade. The obtained plant material and the purchased samples of dried rhizomes were subjected to hydrodistillation. Every time fifty grams of plant material were covered with 100 mL of water, sonicated for 15 min at room temperature and subjected to hydrodistillation in Deryng apparatus for 4 h. The obtained essential oil was later collected from the apparatus to a glass vial, dried from water using sodium sulfate, and diluted 100 times with methanol. The extracts were filtered through a nylon syringe filter (pore diameter of 0.22 µm, Sigma Aldrich, St. Louis, MO, USA) and immediately subjected to compositional studies. The purchased essential oil samples were diluted 100 times prior the compositional analysis, filtered and analysed as described above.
Identification of components in the EO by HPLC-ESI-QTOF-MS/MS
Qualitative analysis of the analysed samples and fractions from CPC analyses was performed by an analytical platform—the HPLC-ESI-QTOF-MS/MS chromatograph 6500 Series (Agilent Technologies, Santa Clara, CA, USA) composed of a binary pump, a degasser, an autosampler, a thermostat, a DAD detector and mass spectrometer (G6530B). The following chromatographic conditions were applied in the study: flow rate of 0.2 mL/min, temperature of 21 °C, injection volume of 2 µL, the UV signals: 280, 254, 210, 230 and 320 nm and the run time of 55 min. The gradient of acetonitrile (solvent B) and water—both with the addition of 0.1% formic acid were used in the following program: 0 min—1% B, 4 min—30% B, 34 min—60% B, 40 min—95% B, 44 min 95% B, 45—1% B. The chromatographic separation was performed on a Zorbax Eclipse Plus RP-18 column (150 mm × 2.1. mm; dp = 3.5 μm) produced by Agilent Technologies (Santa Clara, Ca, USA).
Freshly calibrated and tuned mass spectrometer was operated within the m/z range of 40–1200 u, in the further described settings: gas temperature of 250 °C, sheath gas temperature of 300 °C, gas and sheath gas flows of 12 L/min, nebulizer pressure of 35 psig, capillary voltage of 2500 V, nozzle voltage of 1000 V, fragmentor voltage of 100 V, collision energies of 10 and 20 V, skimmer voltage of 65 V. Mass Hunter Workstation Software (v. B.08.00) was used to record and process the collected data. The structure elucidation was based on the comparison of the obtained data with those present in the scientific literature (retention time, high accuracy mass measurement, the analysis of their MS/MS spectra) in a constructed method that aimed at the fragmentation of two the most intensive m/z signals in each scan, in two collision energies.
Quantitative analysis of β-asarone content in EO by HPLC-DAD
The quantitative analysis of asarones in EO samples was performed by HPLC-DAD chromatograph produced by Shimadzu (Kyoto, Japan). The instrument was composed of a quaternary pump (LC-20AD), a degasser (DGU-20A), an autosampler (SIL-20A HT), a Photodiode Array Detector (SPD-M20A), and a column oven (CTO-10AS VP). The collection and handling of data was supported by the LabSolutions program. The chromatographic separation was performed on Phenomenex Luna 100 C18 (2) column (150 × 4.6 mm, 5 µm) (Phenomenex, Torrance, CA, USA). The isocratic elution mode of 55% methanol in water was applied (v/v). The flow rate was set at 1 mL/min and the detection wavelength used to calculate the content of asarones was selected as 235 nm. First, the concentration of β-asarone in the tested samples was calculated based on the calibration curve of the authentic standard. For this purpose a freshly prepared 2 mg/ mL solution of β-asarone standard in methanol (95% HPLC purity, Sigma Aldrich, St. Louis, MO, USA) was injected in the concentration range of 0.01–2 mg/mL from 5 individual solutions prepared by the dilution of the stock. The obtained calibration curve equation was calculated as y = 160320853x—5933.5 and the regression coefficient value as R2 = 0.9999. Also, the relative amount of asarones (α-, β- and γ-asarone) was calculated based on the HPLC chromatograms.
Statistical analysis
All the chemometric analyses and visualizations were performed using R v4.2.056 programming language in RStudio57 software with pracma58, factoextra59, and matlib60 packages installed.
Principal component analysis (PCA)
Before the assessments, all peak areas of the three traced asarones of all nine samples were summed to 100%, hence the amount of every asarone was represented as a percentage share of a total detector response of a given source (Table 2). After the percentage rescaling of samples, all the variables (i.e. α-, β- and γ-asarone) were autoscaled in order to enable a formal decomposition of the covariance matrix. As a result of the application of standard principal component analysis (PCA) algorithm, first two principal components (PCs) were considered relevant in the presented case. In the next step, their vectors were rotated in space in order to maximize values of correlation coefficients between the original variables and the two orthogonal factors using VARIMAX approach. The linear map of samples (sources) in the space of the resulting varivectors (referred to as ‘dimensions’ in the main text) was prepared by multiplying the matrix of original variables’ loadings in the space of varivectors by the matrix of the autoscaled dataset (see Tables S2 and S3 in the Supplementary File).
Hierarchical clustering
Hierarchical clustering for the studied asarones’ case was executed using Ward method, basing on a standard matrix of Euclidean distances between the sources.
Centrifugal partition chromatography method of removing β-asarone
Biphasic solvent system selection
The biphasic solvent system to be used for the fractionation of Acorus calamus EO by means of Centrifugal Partition Chromatography was established by determining its partition coefficient (Kd) in several biphasic solvents mixtures, using the shake-flask method. The selection criteria for the system were based on the differences in partition coefficient values of single constituents of the extract and the selectivity (α) of every system for α-asarone and β-asarone. These parameters were determined as follows: 4.95 mL of upper and lower phases of the corresponding solvent system were placed in a test tube and 0.1 mL of the Acorus calamus EO was added. The tubes were vigorously shaken and after equilibrium was achieved, both phases were separately filtered through a syringe filter (pore diameter of 0.22 µm) into separate vials and analysed by HPLC-DAD using the chromatographic conditions described in section Quantitative analysis of β-asarone content.
The Kd values of α-asarone and β-asarone were defined as the peak area of the corresponding compound in the lower phase divided by the peak area of the compound in the upper phase. Next, Kd ratios of each compound were calculated to determine the selectivity (α) of each solvent system, according to Ito and Golden44 and the formula below.
where: Kd1, Kd2—partition coefficient of two asarones, where Kd1 > Kd2.
Acorus calamus EO fractionation
The removal of β-asarone from the essential oil was performed by means of centrifugal partition chromatography (CPC) on a Gilson CPC-250 apparatus (Gilson Inc., Middleton, WI, USA), equipped with the Ecom flash 14 DAD 600 detector (Gilson Inc., Middleton, WI, USA) and Gilson fraction collector LS-5600 (Gilson Inc., Middleton, WI, USA). The biphasic liquid system HEMWat/ Arizona X (n-hexane/EtOAc/MeOH/water, 9: 1: 9: 1 v/v/v/v) was prepared by mixing the respective portions of the solvents at room temperature. The separation was performed in ascending mode, using the upper phase as the mobile phase. First, the lower phase was loaded to the column at a flow rate of 30 mL/min, at rotational speed of 500 rpm and the time of 12 min. Next the upper phase was pumped through the stationary phase for the following 13 min at a flow rate of 5 mL/min, rotational speed of 2200 rpm, until equilibrium was achieved with a pressure of 50 bar. The stationary phase retention (Sf) was determined according to Berthod and Faure45 and the equation below.
where: Vs—retained volume of stationary phase in the column after the equilibrium; Vc—column volume.
Then, 1 mL of the Acorus calamus essential oil, dissolved in 9 mL of equal volumes of upper and lower phase (1:1, v/v), was introduced via manual injection valve and an injection loop of 10 mL. The upper phase was pumped at the analytical conditions during 150 min, while fractionation occurred (12 mL/fraction). Afterwards, the lower phase was introduced to the column at a flow rate of 30 mL/min and a rotational speed of 500 rpm during 10 min, as an extrusion step, totalizing 160 min of the fractionation. The effluent was monitored at a wavelength of 254 nm into 12 mL volume vessels. Data were collected and processed with Gilson Glider CPC V5.1d.01 software. The composition of fractions was confirmed by HPLC-ESI-QTOF-MS/MS instrumentation, whereas their purity with help of HPLC-DAD apparatus according to the previously described protocol. All the fractions except those corresponding to asarones were combined and the solvents evaporated under reduced pressure at the temperature not exceeding 40 °C.
Data availability
Most of the data generated or analyzed during this study are included in this published article (and its Supplementary Data files).
References
Samfira, I. et al. Characterization and identity confirmation of essential oils by mid infrared absorption spectrophotometry. Dig. J. Nanomater. Biostruct. 10, 557–566 (2015).
Nasrollahi, S., Ghoreishi, S. M., Ebrahimabadi, A. H. & Khoobi, A. Gas chromatography-mass spectrometry analysis and antimicrobial, antioxidant and anti-cancer activities of essential oils and extracts of Stachys schtschegleevii plant as biological macromolecules. Int. J. Biol. Macromol. 128, 718–723 (2019).
Satyal, P. et al. Chemical compositions, phytotoxicity, and biological activities of Acorus calamus essential oils from Nepal. Nat. Prod. Commun. 8, 1179–1181 (2013).
Sharma, V. et al. Role of Vacha (Acorus calamus Linn.) in neurological and metabolic disorders: Evidence from ethnopharmacology, phytochemistry, pharmacology and clinical study. J Clin. Med. 9, 1176 (2020).
Ni, G. & Yu, D.-Q. Chemical constituents from rhizomes of Acorus tatarinowii. Zhongguo Zhong Yao Za Zhi 38, 569–573 (2013).
Rana, T. S., Mahar, K. S., Pandey, M. M., Srivastava, S. K. & Rawat, A. K. S. Molecular and chemical profiling of ‘sweet flag’ (Acorus calamus L.) germplasm from India. Physiol. Mol. Biol. Plants 19, 231–237 (2013).
Uebel, T., Hermes, L., Haupenthal, S., Müller, L. & Esselen, M. α-Asarone, β-asarone, and γ-asarone: Current status of toxicological evaluation. J. Appl. Toxicol. 41, 1166–1179 (2021).
Ogra, R. K. et al. Indian calamus (Acorus calamus L.): Not a tetraploid. Curr. Sci. 97, 1644–1647 (2009).
Mukherjee, P. K. et al. Plants of Indian origin in drug discovery. Expert Opin. Drug Discov. 2, 633–657 (2007).
Rajput, S. B., Tonge, M. B. & Karuppayil, S. M. An overview on traditional uses and pharmacological profile of Acorus calamus Linn. (Sweet flag) and other Acorus species. Phytomedicine 21, 268–276 (2014).
Das, B. K., Swamy, A. V., Koti, B. C. & Gadad, P. C. Experimental evidence for use of Acorus calamus (asarone) for cancer chemoprevention. Heliyon 5, e01585 (2019).
O’Neill, A. R., Badola, H. K., Dhyani, P. P. & Rana, S. K. Integrating ethnobiological knowledge into biodiversity conservation in the Eastern Himalayas. J. Ethnobiol. Ethnomed. 13, 21 (2017).
Kaushik, R. et al. Isolation of β-asarone from Acorus calamus Linn. and evaluation of its anticonvulsant activity using MES and PTZ models in mice. Pharmacol. Toxicol. Biomed. Rep. 3, 21–26 (2017).
McGaw, L. J., Jäger, A. K., van Staden, J. & Eloff, J. N. Isolation of β-asarone, an antibacterial and anthelmintic compound, from Acorus calamus in South Africa. S. Afr. J. Bot. 68, 31–35 (2002).
Hernández-Moreno, D. et al. Consumer and farmer safety evaluation of application of botanical pesticides in black pepper crop protection. Food Chem. Toxicol. 56, 483–490 (2013).
Sumalan, R. M. et al. Exploring ecological alternatives for crop protection using coriandrum sativum essential oil. Molecules 24, 1–14 (2019).
Kovaříková, K. & Pavela, R. United forces of botanical oils: Efficacy of neem and karanja oil against colorado potato beetle under laboratory conditions. Plants 8, 608 (2019).
Guimarães, A. C. et al. Antibacterial activity of terpenes and terpenoids present in essential oils. Molecules 24, 2471 (2019).
Pavela, R. & Benelli, G. Essential oils as ecofriendly biopesticides? Challenges and constraints. Trends Plant Sci. 21, 1000–1007 (2016).
Butnariu, M., Caunii, A. & Putnoky, S. Reverse phase chromatographic behaviour of major components in Capsicum Annuumextract. Chem. Cent. J. 6, 146 (2012).
Ito, Y. & Bowman, R. L. Countercurrent chromatography: Liquid–Liquid partition chromatography without solid support. Science 1979(167), 281–283 (1970).
Bojczuk, M., Żyżelewicz, D. & Hodurek, P. Centrifugal partition chromatography—A review of recent applications and some classic references. J. Sep. Sci. 40, 1597–1609 (2017).
Murayama, W. et al. A new centrifugal counter-current chromatograph and its application. J. Chromatogr. A 239, 643–649 (1982).
Berthod, A. & Carda-Broch, S. Determination of liquid–liquid partition coefficients by separation methods. J. Chromatogr. A 1037, 3–14 (2004).
da Silva, L. A. L. et al. A single-step isolation by centrifugal partition chromatography of the potential anti-inflammatory glaucolide B from Lepidaploa chamissonis. J. Chromatogr. A 1605, 460362 (2019).
Kukula-Koch, W., Koch, W., Angelis, A., Halabalaki, M. & Aligiannis, N. Application of pH-zone refining hydrostatic countercurrent chromatography (hCCC) for the recovery of antioxidant phenolics and the isolation of alkaloids from Siberian barberry herb. Food Chem. 203, 394–401 (2016).
Dang, Y. Y., Li, X. C., Zhang, Q. W., Li, S. P. & Wang, Y. T. Preparative isolation and purification of six volatile compounds from essential oil of Curcuma wenyujin using high-performance centrifugal partition chromatography. J. Sep. Sci. 33, 1658–1664 (2010).
Li, X.-C., Zhang, Q.-W., Yin, Z.-Q., Zhang, X.-Q. & Ye, W.-C. Preparative separation of patchouli alcohol from patchouli oil using high performance centrifugal partition chromatography. J. Essent. Oil Res. 23, 19–24 (2011).
Liakakou, A. et al. Isolation of volatile compounds with repellent properties against Aedes albopictus (Diptera: Culicidae) using CPC technology. Molecules 26, 3072 (2021).
Matsunaga, T. et al. Isolation of the antiulcer compound in essential oil from the leaves of Cryptomeria japonica. Biol. Pharm. Bull. 23, 595–598 (2000).
Sinha, A. K., Joshi, B. P., Sharma, A., Goel, H. C. & Prasad, J. Ultrasound-assisted conversion of toxic β-asarone into nontoxic bioactive phenylpropanoid: Isoacoramone, a metabolite of Piper Marginatum and Acorus Tararinowii. Nat. Prod. Res. 18, 219–223 (2004).
Jaiswal, Y., Liang, Z., Ho, A., Chen, H. & Zhao, Z. Metabolite profiling of tissues of Acorus calamus and Acorus tatarinowii rhizomes by using LMD, UHPLC-QTOF MS, and GC-MS. Planta Med. 81, 333–341 (2015).
Strzępek-Gomółka, M. et al. Identification of mushroom and murine tyrosinase inhibitors from Achillea biebersteinii Afan. Extract. Mol. 26, 964 (2021).
Zhang, F. et al. Qualitative and quantitative analysis of the major constituents in Acorus tatarinowii Schott by HPLC/ESI-QTOF-MS/MS. Biomed. Chromatogr. 29, 890–901 (2015).
Yang, R., Yan, Z., Chen, C. & Liu, J. Constituents and Activities of Acorus tatarinowii. Med Res Arch 5, (2017).
Yang, Q. et al. Development of an LC-MS/MS method for quantification of two isomeric phenylpropenes and the application to pharmacokinetic studies in rats. Biomed. Chromatogr. 32, e4115 (2018).
Yannai, S. Dictionary of Food Compounds with CD-ROM (Chapman and Hall/CRC, 2003). https://doi.org/10.1201/9781420068450.
Loying, R. et al. Chemical compositions, in-vitro antioxidant, anti-microbial, anti-inflammatory and cytotoxic activities of essential oil of Acorus calamus L. rhizome from North-East India. J. Essent. Oil Bear. Plants 22, 1299–1312 (2019).
Dušek, K., Galambosi, B., Hethelyi, E. B., Korany, K. & Karlová, K. Morphological and chemical variations of sweet flag (Acorus calamus L.) in the Czech and Finnish gene bank collection. Hortic. Sci. 34, 17–25 (2007).
Raal, A., Orav, A. & Gretchushnikova, T. β-Asarone content and essential oil composition of Acorus calamus L. rhizomes from Estonia. J. Essential Oil Res. 28, 299–304 (2016).
Lorántfy, L. et al. Continuous industrial-scale centrifugal partition chromatography with automatic solvent system handling: Concept and instrumentation. Org. Process Res. Dev. 24, 2676–2688 (2020).
Namal Senanayake, S. P. J. Methods of concentration and purification of omega-3 fatty acids. In Separation, Extraction and Concentration Processes in the Food, Beverage and Nutraceutical Industries 483–505 (Elsevier, 2013). https://doi.org/10.1533/9780857090751.2.483.
Popp, J. R. et al. Rapid isolation of acidic cannabinoids from Cannabis sativa L. using pH-zone-refining centrifugal partition chromatography. J. Chromatogr. A 1599, 196–202 (2019).
Ito, Y. Golden rules and pitfalls in selecting optimum conditions for high-speed counter-current chromatography. J. Chromatogr. A 1065, 145–168 (2005).
Berthod, A. & Faure, K. Separations with a Liquid Stationary Phase: Countercurrent Chromatography or Centrifugal Partition Chromatography. In Analytical Separation Science Vol. 4 1177–1206 (Wiley-VCH Verlag GmbH & Co. KGaA, 2015).
Foucault, A. P. Centrifugal Partition Chromatography Vol. 68 (Taylor & Francis Inc, 1995).
Chen, H. P. et al. Repellant and insecticidal activities of shyobunone and isoshyobunone derived from the essential oil of Acorus calamus rhizomes. Pharmacogn. Mag. 11, 675–681 (2015).
St-Gelais, A. et al. Preisocalamendiol, shyobunol and related oxygenated sesquiterpenes from Bolivian Schinus molle Essential Oil. Nat. Prod. Commun. 11, 547–550 (2016).
Prichoa, F. C., Leyser, G., Oliveira, J. V. & Cansian, R. L. Comparative allelopathic effects of Cryptocarya moschata and Ocotea odorifera aqueous extracts on Lactuca sativa. Acta Sci. Agron. 35, 197–202 (2013).
Dias, J. F. G., Miguel, O. G. & Miguel, M. D. Composition of essential oil and allelopathic activity of aromatic water of Aster lanceolatus Willd. (Asteraceae). Braz. J. Pharm. Sci. 45, 469–474 (2009).
El-Gawad, A., Elshamy, A., el Gendy, A., Gaara, A. & Assaeed, A. Volatiles profiling, allelopathic activity, and antioxidant potentiality of Xanthium strumarium leaves essential oil from Egypt: Evidence from chemometrics analysis. Molecules 24, 584 (2019).
Liu, X., Zhou, L., Liu, Z. & Du, S. Identification of insecticidal constituents of the essential oil of Acorus calamus rhizomes against Liposcelis bostrychophila Badonnel. Molecules 18, 5684–5696 (2013).
Pentea, M., Butu, M., Samfira, I., Cristina, R. & Butnariu, M. Extraction and analytical study of salvinorin a from leaves of salvia Divinorum. Dig. J. Nanomater. Biostruct. 10, 291–297 (2015).
Ianculov, I., Palicica, R., Butnariu, M., Dumbrava, D. & Gergen, I. The obtaining of chlorophyll in crystalline form from fir needles (Abies alba) and from pine needles (Pinus silvestris). Rev. Chim. 56, 441–443 (2005).
Hijmans, R. J., Cruz, M., Rojas, E. & Guarino, L. DIVA-GIS, version 1.4. A geographic information system for the management and analysis of genetic resources data. Manual. (2001).
R Core Team. R: A language and environment for statistical computing. https://www.R-project.org (2022).
RStudio Team. RStudio: Integrated development environment for R. http://www.rstudio.com/ (2020).
Borchers, H. W. pracma: Practical numerical math functions, version 2.3.8 from R-Forge. Preprint (2022).
Kassambara, A. & Mundt, F. Factoextra: Extract and visualize the results of multivariate data analyses. Preprint (2020).
Friendly, M., Fox, J. & Chalmers, P. matlib: Matrix functions for teaching and learning linear algebra and multivariate statistics. Preprint (2021).
Acknowledgements
The authors would like to thank for their invaluable help: Ms Aneta Rosik in obtaining calamus rhizomes from the natural environment and Agnieszka Rewicz, PhD (University of Lodz), for help with the preparation of the map in Fig. 8.
Funding
Financial support of these studies from Gdańsk University of Technology by the DEC-47/2021/IDUB/I.3.3 grant under the ARGENTUM—’Excellence Initiative—Research University’ program is gratefully acknowledged.
Author information
Authors and Affiliations
Contributions
P.S., J.T.P., Ł.S. and W.K.-K. conceived the experiments, M.W., T.B., J.G., M.K. and J.B.-B. conducted the experiments, P.S., T.L., J.T.P. and W.K.-K. analyzed the results. P.S., M.W., J.T.P., T.L. and W.K.-K. wrote the main manuscript and prepared Figs. 1, 3, 4, 5, 6, 7 and 8 and A.S. prepared Fig. 2. P.S, W.K.-K., M.W. and A.S. prepared revised version of the manuscript. All authors have reviewed the manuscript.
Corresponding author
Ethics declarations
Competing interests
The authors declare no competing interests.
Additional information
Publisher's note
Springer Nature remains neutral with regard to jurisdictional claims in published maps and institutional affiliations.
Supplementary Information
Rights and permissions
Open Access This article is licensed under a Creative Commons Attribution 4.0 International License, which permits use, sharing, adaptation, distribution and reproduction in any medium or format, as long as you give appropriate credit to the original author(s) and the source, provide a link to the Creative Commons licence, and indicate if changes were made. The images or other third party material in this article are included in the article's Creative Commons licence, unless indicated otherwise in a credit line to the material. If material is not included in the article's Creative Commons licence and your intended use is not permitted by statutory regulation or exceeds the permitted use, you will need to obtain permission directly from the copyright holder. To view a copy of this licence, visit http://creativecommons.org/licenses/by/4.0/.
About this article
Cite this article
Szczeblewski, P., Wróblewski, M., Borzyszkowska-Bukowska, J. et al. The role of centrifugal partition chromatography in the removal of β-asarone from Acorus calamus essential oil. Sci Rep 12, 22217 (2022). https://doi.org/10.1038/s41598-022-26726-6
Received:
Accepted:
Published:
DOI: https://doi.org/10.1038/s41598-022-26726-6
This article is cited by
Comments
By submitting a comment you agree to abide by our Terms and Community Guidelines. If you find something abusive or that does not comply with our terms or guidelines please flag it as inappropriate.