- OUTLOOK
Designer viruses could be the secret to cheaper and better gene therapy
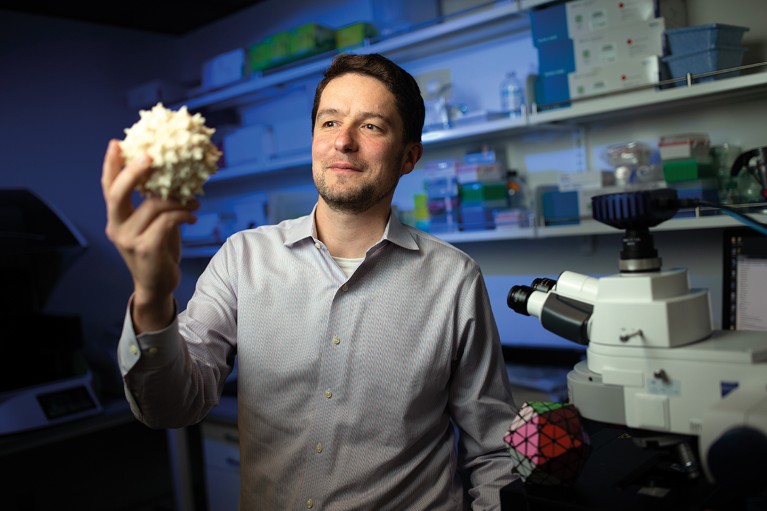
Luk Vandenberghe at Massachusetts Eye and Ear in Boston holds a model of an adeno-associated virus. Credit: Aram Boghosian/Massachusetts Eye and Ear
Access options
Access Nature and 54 other Nature Portfolio journals
Get Nature+, our best-value online-access subscription
$29.99 / 30 days
cancel any time
Subscribe to this journal
Receive 51 print issues and online access
$199.00 per year
only $3.90 per issue
Rent or buy this article
Prices vary by article type
from$1.95
to$39.95
Prices may be subject to local taxes which are calculated during checkout
Nature 564, S18-S19 (2018)
doi: https://doi.org/10.1038/d41586-018-07647-9
This article is part of Nature Outlook: Gene therapy, an editorially independent supplement produced with the financial support of third parties. About this content.
References
Gao, G. et al. J. Virol. 78, 6381–6388 (2004).
Zinn, E. et al. Cell Rep. 12, 1056–1068 (2015).
Pan, B. et al. Nature Biotech. 35, 264–272 (2017).