« Prev Next »

Until recently, most discussions of modern global warming have looked only as far ahead as 2100 AD. Now, new investigations by pioneering climate modelers are beginning to tell another story, one in which the legacy of our heat-trapping carbon emissions lasts not just decades or centuries but long enough to interfere with future ice ages. As science-journalist Mason Inman (2005) puts it, with only slight exaggeration, "carbon is forever."
Specialists are now investigating the long-term future of our greenhouse gas pollution with the help of a new generation of sophisticated climate models with names like CLIMBER, GENIE, and LOVECLIM. But the basics of that future boil down to one simple principle: what goes up must come down.
Climate Whiplash
Greenhouse gas concentrations and global temperatures will not increase indefinitely — today's carbon dioxide buildup and warming trend must eventually top out and then reverse as the atmosphere gradually recovers. The first stage of this process will occur when the rate at which we burn coal, oil, and natural gas levels off and then declines, either because we switch to alternative energy sources soon, or because we run out of affordable fossil fuels later. As a result, CO2 concentrations in the atmosphere will also eventually peak and then decline. This, in turn, will cause a series of linked environmental responses in which other currently rising trends reverse one by one in a "climate whiplash" phase that follows the lead of our carbon emissions. For example, as CO2 dissolves into the oceans, it combines with water to form carbonic acid, which alters the chemistry of seawater and makes limestone, chalk, and other carbonate-rich substances more likely to dissolve. Ocean acidification will peak shortly after atmospheric CO2 concentrations do, threatening marine species that have acid-soluble carbonate shells or skeletons, including corals, shellfish, and crustaceans (Figure 1).
After a delay due to slow response times in the atmosphere and oceans (Wigley 2005), global average temperatures will pivot into cooling mode as CO2 concentrations continue to fall. However, global mean sea level will still rise long after the thermal peak passes, because even though temperatures will be falling, they will still be warmer than today. Therefore, land-based glacial ice will continue to melt and the oceans will continue to expand even though Earth's atmosphere has begun to recover. Sea level will only return to today's position when it finally becomes cool enough for large, land-based ice sheets to build up again on Antarctica and in the Arctic.
Where Does the Carbon Go?
In order to work out the timing of these processes in more detail, one must consider where CO2 goes after it leaves our smokestacks and exhaust pipes. Some of it will be taken up by soils and organisms but most of it will dissolve into the oceans, with between two thirds and half of our emissions perhaps going into solution during the next millennium or so (Inman 2008, Eby et al. 2009). In many computer simulations, maximum ocean acidification lasts 2000 years or more, depending on the amount of CO2 we emit in the near future. Marine species living in the polar regions and deep sea basins and trenches will be the most rapidly and severely impacted because the solubility of such gases is greatest in cold waters. But after the seas have absorbed as much CO2 as they can, roughly a fifth of our fossil carbon emissions will still be left adrift in the air (Tyrell et al. 2007, Inman 2008).
The next stage of the cleanup will proceed more slowly. As atmospheric CO2 dissolves into raindrops, the carbonic acid that it produces will react with calcite and other carbonate minerals in rocks and sediments. Over thousands of years, those geochemical weathering processes will transfer many of the formerly airborne carbon atoms into groundwater and runoff, finally delivering them to the oceans in the form of dissolved bicarbonate and carbonate ions. Meanwhile, carbonate-rich deposits on the sea floor will experience similar reactions with overlying seawater as the oceans become more acidified. This slow addition of acid-buffering substances to marine ecosystems will act much like an antacid pill that allows the seas to consume more CO2 from the overlying atmosphere. These processes are generally expected to dominate the long-term recovery for 5,000 years or so.
But even this second, lengthier phase won't remove the very last fraction of our carbon pollution. Only tens of thousands of years later, or possibly even hundreds of thousands if we burn most of our enormous coal reserves, the last remnants of our CO2 will finally be scrubbed away by even slower reactions with resistant silicate minerals, such as the feldspars found in granite and basalt. This is what University of Chicago oceanographer David Archer calls "the long tail of the carbon curve" (Archer 2005), and it will be dominated by gradual global cooling, albeit at higher temperatures than those of today.
Choices Before Us
The intensity and duration of the warming peak and recovery will depend upon choices we make during this century. If we switch to carbon-free energy sources during the next several decades, then approximately 1000 gigatons of fossil carbon will have been released into the atmosphere since the start of the Industrial Revolution (1 gigaton = 1 billion tons). Atmospheric CO2 concentrations will peak close to 550–600 parts per million (ppm) by 2200 AD or so, and then begin to fall (Figure 2; Archer 2005, Archer & Brovkin 2008).


In the climate whiplash phase that follows this relatively moderate scenario, global mean temperatures are likely to climb 2–3°C higher than today by 2200–2300 AD, then enter a cooling recovery phase lasting as much as 100,000 years. Much of Greenland and western Antarctica's ice will melt into the oceans over millennia, lifting sea levels several meters higher than today before slowly receding.
On the other hand, if we burn through all remaining coal reserves before switching to alternative energy sources, then a far more extreme scenario will result. In one computer simulation of what could follow a 5000 gigaton emission (Figure 3; Schmittner et al. 2008), airborne CO2 concentrations reach 1900–2000 ppm, roughly five times greater than today, by 2300 AD. Global mean temperature jumps 6–9 °C above today's average and remains artificially high for much longer than it does in the more moderate scenario, with the warmest part of the broad maximum lasting from 3000 AD to 4000 AD. Atmospheric CO2 concentrations and temperatures then fall relatively steeply for several thousand years after the peak and whiplash phase, but they don't return to today's levels for at least 400,000 years. All land-based ice eventually melts, raising sea levels by as much as 70 meters until the world cools enough for large polar ice sheets to form again, roughly half a million years from now.


Life in a Hothouse
What might life on Earth be like under such conditions? Although no examples from the past perfectly illustrate the warmest phases of these two scenarios, several of them are nonetheless informative.
Immediately before the last ice age-between 130,000 and 117,000 years ago-a natural warming episode known in Europe as the Eemian Interglacial produced global average temperatures 2–3°C higher than those of today, much like what would be expected in our more moderate scenario. The surface area of the Greenland ice sheet shrank by at least a third, the Arctic Ocean lost some summer ice-cover but retained enough for ringed seals and polar bears to survive, elephants and water buffalo migrated northward into Britain and Europe, and trees that are now more typical of the southeastern United States, such as black gums and hickories, thrived in the Adirondack Mountains of upstate New York (Stager 2011). Although it was caused by cyclic changes in the orientation of the Earth relative to the sun rather than greenhouse gases, the Eemian example nonetheless shows that even a relatively moderate warming can melt enough land-based ice to raise sea levels by 6–9 meters if it persists long enough, which in this case was 13,000 years (Figure 4).


The more extreme of the two emissions scenarios is better illustrated by a super-hothouse that occurred 55 million years ago-roughly 10 million years after the demise of the dinosaurs. Geo-historical evidence shows that the Paleocene-Eocene Thermal Maximum (PETM) was triggered by greenhouse gas buildups, most likely from the release of icy methane hydrates or other carbon compounds buried in marine deposits (Katz et al. 1999). Global mean temperatures rose 5–6°C within several thousand years and did not fully recover for 100,000–200,000 years (Zachos et al. 2003, Rohl et al. 2007, Jaramillo et al. 2010). Both polar regions were completely ice-free, the Arctic Ocean was a warm, brackish pond rimmed by deciduous redwood forests, Antarctica was covered by beech trees, and carbonic acid burned a discolored, carbonate-free band into ocean sediments worldwide (Zachos et al. 2005). Some species became extinct during the PETM, especially in the most heavily acid-impacted portions of the oceans (Gibbs et al. 2006), but many others thrived, sometimes spreading so rapidly between latitudes and continents that they seemed to appear simultaneously in fossil records all over the world (Smith et al. 2006, Jaramillo et al. 2010).
In both of these cases, free migration seems to have been an important key to the survival of animals and plants of the time, and the lack of human-made barriers in the distant past made it easier for species to adjust to large climatic shifts. Unfortunately, our settlements, roads, and farms can make such migrations more difficult today, and will probably do so in the future as well.
Climate Ethics
Such long-term perspectives are not only scientifically interesting and important, they also raise new ethical questions, simply because human beings are now in the picture. Our carbon emissions will influence countless generations, as well as many species other than our own, in future versions of the world that will differ markedly from the one we know now. This realization may force us to weigh the needs of some generations against those of others.
For instance, having the Arctic Ocean become ice-free in summer may seem outlandish to us, but it may instead seem normal to people who will be born into a warmer world thousands of years from now. When the global cooling recovery sets in, the open-water ecosystems and human cultures that will by then have become dependent upon warmer climates could be threatened as the polar ocean begins to re-freeze. Will global warming seem preferable to cooling then?
Another potentially confusing situation arises when we consider that atmospheric CO2 concentrations will still be high enough in 50,000 AD to prevent the next ice age, which natural cyclic processes would normally be expected to trigger then (Figure 5; Berger & Loutre 2002, Archer & Ganopolski 2005). The next major cyclic cool period is due in 130,000 AD, by which time a moderate carbon emission will have dissipated. This suggests that preventing an extreme 5000 Gton hothouse scenario now could leave Canada and northern Europe vulnerable to being bulldozed by gigantic ice sheets in the deep future. How do we weigh the winners and losers in such a far-sighted view?


Fortunately, long-term perspectives may also suggest possible win-win situations, as well. For instance, leaving most remaining coal untouched rather than using it all up now would reduce the severity of climate change in the near-term, and would also leave large stores of burnable carbon in the ground that later generations could use as a source of greenhouse gases for the prevention of future ice ages, should they so desire.
Whichever emissions scenario we choose-be it moderate or extreme-one thing is now clear. Our influence on the climatic future of the world is geological in scope. Little wonder, then, that many scientists are now referring to our chapter of Earth history with a term coined by ecologist Eugene Stoermer-the "Anthropocene Epoch" or the "Age of Humans" (Crutzen & Stoermer 2000, Stager 2011).
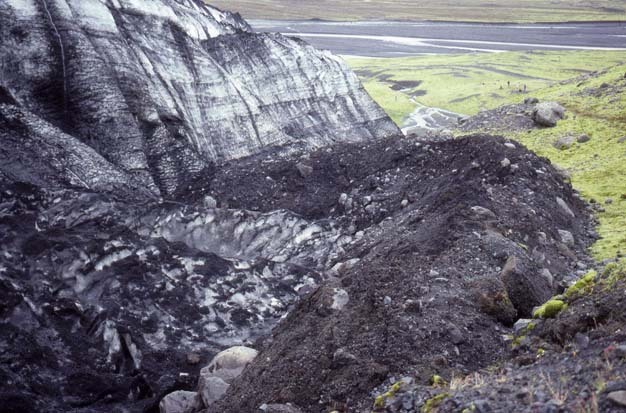

References and Recommended Reading
Archer, D. The fate of fossil fuel CO2 in geologic time. Journal of Geophysical Research 110, C09805 (2005). doi:10.1029/2004/C002625
Archer, D. & Ganopolski, A. A movable trigger: Fossil fuel CO and the onset of the next glaciation. Geochemistry, Geophysics, Geosystems 6, Q05003 (2005). doi:1029/2004GC000891
Archer, D. & Brovkin, V. The millennial lifetime of anthropogenic CO2. Climatic Change 90, 283–297 (2008).
Berger, A. & Loutre, M. F. An exceptionally long interglacial ahead? Science 297, 1287–1288 (2002).
Crutzen, P. J. & Stoermer, E. F. "The 'Anthropocene'". Global Change Newsletter 41, 17–18 (2000).
Eby, M. et al. Lifetime of anthropogenic climate change: Millennial time scales of potential CO2 and surface temperature perturbations. Journal of Climate 22, 2501–2511 (2009).
Gibbs, S. J. et al. Nannoplankton extinction and origination across the Paleocene-Eocene Thermal Maximum. Science 314, 1770–1773 (2006).
Inman, M. Carbon is forever. Nature Reports Climate Change (2008). doi:10.1038/climate.2008.122
Jaramillo, C. et al. Effects of rapid global warming at the Paleocene-Eocene boundary on neotropical vegetation. Science 330, 957–961 (2010).
Katz, M. E. et al. The source and fate of massive carbon input during the Latest Paleocene Thermal Maximum. Science 286, 1531–1533 (1999).
Montenegro, A. et al. Long-term fate of anthropogenic carbon. Geophysical Research Letters 34, L19707 (2007). doi:1029/2007GL030905
Röhl, U. et al. On the duration of the Paleocene-Eocene thermal maximum (PETM). Geochemistry Geophysics Geosystems 8, Q12002 (2007). doi:10.1029/2007GC001784
Schmittner, A. et al. Future changes in climate, ocean circulation, ecosystems, and biogeochemical cycling simulated for a business-as-usual CO2 emission scenario until year 4000 AD. Global Biogeochemical Cycles 22, GB1013 (2008). doi:10.1029/2007GB002953
Smith, T. K., Rose, D. & Gingerich, P. D. Rapid Asia-Europe-North America dispersal of the earliest Eocene primate Teilhardina. Proceedings of the National Academy of Sciences of the United States of America 103, 11223–11227 (2009).
Solomon, S. et al. Irreversible climate change due to carbon dioxide emissions. Proceedings of the National Academy of Sciences of the United States of America 106, 1704-1709 (2009).
Stager, C. Deep Future: The Next 100,000 Years of Life on Earth. New York, NY: Thomas Dunne Books, 2011. (link)
Tyrrell, T., Shepherd, J. G. & Castle, S. The long-term legacy of fossil fuels. Tellus, Series B: Chemical and Physical Meteorology 59B, 664–672 (2007).
Wigley, T. M. L. The climate change commitment. Science 307, 1766–1769 (2005).
Zachos, J. C. et al. A transient rise in tropical sea surface temperature during the Paleocene-Eocene Thermal Maximum. Science 302, 1551–1554 (2003).
Zachos, J. C. et al. Rapid acidification of the ocean during the Paleocene-Eocene thermal maximum. Science 308, 1611–1615 (2005).