Abstract
Spontaneously occurring canine mammary tumours (CMTs) are the most common neoplasms of female unspayed dogs and are of potential importance as models for human breast cancer as well. Mortality rates are thrice higher in dogs as compared to humans with breast cancer, which can partly be attributed to lack of diagnostic techniques for their early detection. Human breast cancer studies reveal role of autoantibodies in early cancer diagnosis and also the usefulness of autoantibody panels in increasing the sensitivity, as well as, specificity of diagnostic assays. Therefore, in this study, we took advantage of high-throughput Luminex technique for developing a multiplex assay to detect autoantibody signatures against 5 canine mammary tumour-associated autoantigens (TAAs). These TAAs were expressed separately as fusion proteins with halo tag at the N-terminus, which allows easy and specific covalent coupling with magnetic microspheres. The multiplex assay, comprising a panel of candidate autoantigens (TPI, PGAM1, MNSOD, CMYC & MUC1) was used for screening circulating autoantibodies in 125 dog sera samples, including 75 mammary tumour sera and 50 healthy dog sera. The area under curve (AUC) of the combined panel of biomarkers is 0.931 (pā<ā0.0001), which validates the discriminative potential of the panel in differentiating tumour patients from healthy controls. The assay could be conducted in 3hrs using only 1ul of serum sample and could detect clinical cases of canine mammary tumour with sensitivity and specificity of 78.6% and 90%, respectively. In this study, we report for the first time a multiplexed assay for detection of autoantibodies in canine tumours, utilizing luminex technology and halo-tag coupling strategy. Further to the best of our knowledge, autoantibodies to CMYC and MUC1 have been reported for the first time in canines in this study.
Similar content being viewed by others
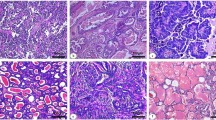
Introduction
The immune system responds to low, usually undetectable levels of tumour-associated autoantigens (TAAs) by mounting a very specific antibody response, providing opportunities for early cancer detection1,2,3,4. Autoantibodies against TAAs have previously been identified in cancers of the colon5, breast6, lung7, ovary8, prostate3, head & neck9, oesophagus10 and pancreas11. Interest in the use of anti-TAA antibodies as serological biomarkers for cancer diagnosis and prognosis derives from the recognition that these autoantibodies are either absent or present in very low titres in healthy individuals. Their measurement in serum is minimally invasive and cost effective using established technologies. Their persistence and stability in the serum of cancer patients is an advantage over other potential markers, including the TAAs themselves, which are released by tumours, but are rapidly degraded or cleared after circulating in the blood for a limited time12. Thus, cancer-associated autoantibodies might be regarded as reporters, identifying aberrant de novo or dysregulated cellular mechanisms in tumorigenesis2,3 and holds great potential as an ideal cancer screening and diagnostic tool13,14. However, most autoantibodies discovered so far have limited diagnostic value alone as frequencies of autoantibodies specific for a particular TAA in a cancer population are often low, ranging from 10ā30%14,15,16. Further, a single antibody may not discriminate between cancer types and may arise as a consequence of molecular events associated with cancer or other diseases. Therefore, multiplexing of autoantibody biomarkers is required to increase the sensitivity and specificity of the diagnostic assays. Microsphere-based suspension array technology based upon the LuminexĀ® xMAP⢠system, offers a new multiplexing platform for high-throughput analyte detection17,18,19. Some benefits of suspension array technology over conventional immunoassays include rapid data acquisition (few hours), excellent sensitivity and specificity, multiplexed analysis capability and low sample requirement. Canine mammary tumour (CMT) is the most common malignancy of unspayed female dogs leading to at least three times higher mortality rates than human breast cancer20. Most of the techniques currently used for CMT diagnosis are invasive and provide diagnosis when the tumour burden has crossed a threshold level. In humans, a number of autoantibody biomarkers have been identified and panel assays against breast cancer21,22,23, lung cancer24, ovarian cancer25 etc., have been developed, demonstrating increased sensitivity and specificity for cancer detection. However, in dogs very few autoantibody biomarkers associated with cancer have been identified and no studies have been conducted so far to evaluate diagnostic utility of autoantibody panels. Considering the significance of autoantibodies as markers for early cancer detection, and multiplexing as a strategy to increase sensitivity, the present study was designed to develop a multiplex autoantibody based panel assay for diagnosis of CMT in dogs. The assay was developed using commercially available magnetic micropsheres (MagPlexĀ® microspheres) which have advantages of maximal recovery during handling and facilitates assay automation. The candidate autoantigens chosen for developing the five-plex assay were triose phosphate isomerase (TPI), manganese-superoxide dismutase (MNSOD), phosphoglycerate mutase1 (PGAM1), avian myelocytomatosis viral oncogene homolog (CMYC) and mucin1 glycoprotein (MUC1). TPI and PGAM1 are glycolytic enzymes involved in the glycolysis metabolism, which plays a critical role in supply of ATP to the neoplastic cells26. TPI & PGAM1 coordinates glycolysis and carbohydrate biosynthesis to promote cancer growth and metastasis. Parallel to glycolytic metabolism, high level of MNSOD is expressed by the cancer cells to cope up higher oxidative stress and MNSOD is now considered as a potential marker for tumour progression & metastasis27,28,29. MUC1 is a transmembrane mucin, which is aberrantly overexpressed in over 90% of breast tumours and is well studied as a diagnostic marker for metastatic progression30,31,32. CMYC (MYC) is a transcription factor regulating more than 15% of human genes, involved in cell proliferation, differentiation, adhesion, apoptosis, and migration33. Deregulated expression of CMYC has been found in multiple cancer types, including human breast cancer34 and canine mammary cancer35. Thus in recent years, MNSOD36, TPI37, PGAM138, MUC132 and CMYC34 have emerged as new targets for cancer diagnosis and therapy. TPI, PGAM1, MNSOD were selected for this study based upon the studies of Zamani-Ahmadmahmudi et al.39, who by serological proteome analysis (SERPA) identified these as potential autoantigens with significantly higher immunoreactivity in canine mammary cancer sera samples. Also in our lab, we have demonstrated diagnostic potential of TPI, MNSOD, PGAM1, MUC1 & CMYC autoantibodies in canine mammary tumours by ELISA (Supplementary TableĀ S8). The autoantibodies to these five antigens were found to be present in higher frequency in canine mammary cancer sera, as compared to healthy dog sera. Further autoantibodies to these five antigens have been identified by various researchers as potential biomarkers in human cancers also. Yang and colleagues identified TPI and MNSOD panel to have potential for diagnosis of early-stage cancers with 47% sensitivity and 90% specificity40. Using immunoproteomic techniques, TPI autoantibodies were demonstrated in high frequency of human breast cancers41,42. Tamesa et al.41 detected autoantibodies against TPI in 90% of the human breast cancer sera samples. High-titre circulating anti-Mucin1 (MUC1) antibodies have been reported in human breast tumor patients43. In other study, autoantibodies against MNSOD have been demonstrated in 37.5% of human breast cancer sera samples44. Similarly, researchers have also reported presence of anti-myc antibodies as diagnostic marker in early stage human cancer patients45. Thus autoantibodies against CMYC45, MUC143,46, MNSOD40, TPI40 have been implicated in human cancers to have relevance for early cancer diagnosis, however the diagnostic suitability of these biomarkers as a panel has not been attempted previously. Therefore, in this study microsphere based multiplex assay was developed for detection of TPI, MNSOD, PGAM1, MUC1 & CMYC autoantibody signatures in dogs suffering from CMT. A total of 125 dog sera samples, including 75 from clinical cases of canine mammary tumour (CMT) and 50 healthy dogs, were analysed to assess the diagnostic potential of the panel assay.
Materials and Methods
Instruments and reagents for multiplex assay
Carboxy-functionalized MagPlex microspheres (#MC10028, MC10035, MC10045, MC10054, MC10064) were purchased from Bio-Rad laboratories India Pvt. Ltd, Gurgaon. The multiplexed assay was analysed on Bio-Plex 200 system, which is a suspension array system with two lasers: the classification laser (635ānm excitation), for identifying the bead signatures, and the reporter laser (532ānm excitation) for detecting the target. 1-Ethyl-3-(3-dimethylaminopropyl)-carbodiimide hydrochloride (EDC, #E6383), N-hydroxysulfosuccinimide (sulfo-NHS, #56485), anti-dog IgG-biotin (#SAB3700111), streptavidin-conjugated phycoerythrin (SA-PE, #42250), phosphate buffered saline (PBS, #P4417), bovine serum albumin (BSA, #A2153) and tween-20 (#P1379) were purchased from Sigma-Aldrich (St. Louis, USA). Rabbit polyclonals against PGAM1 (#SC-292579), TPI (#SC-30145), MNSOD (#SC-30080), CMYC (#SC-788) were purchased from SantaCruz (SantaCruz, USA). Rabbit polyclonal against MUC1 (#AV41445) was purchased from Sigma-Aldrich (St. Louis, USA). Anti-halo tag antibody, halo tag Amine (O4) ligand (#P-6741) and pH6HTN His6HaloTagĀ® T7 vector (#G7971) were purchased from Promega (Promega, USA).
Sera and tumour tissue samples
Sera and tissue samples were collected from dogs that were presented at the āReferral Veterinary Polyclinicā, ICAR-Indian Veterinary Research Institute, Izatnagar, U.P. India. Total 125 dog sera samples were collected including 75 from cases of canine mammary tumour (CMT) and 50 healthy female dogs. Healthy dogs were examined for the absence of any disease by clinico-physical & hematological parameters such as CBC, LFT, KFT and urinalysis. Tumour tissues obtained from tumour biopsy/surgical resections were fixed in neutral buffered formalin for histopathological analysis and immunohistochemistry. Histopathological classification and grading of the paraffin embedded tissue sections was done as per Goldschimdt et al.47. Based on histopathological analysis of hematoxylin and eosin stained tumour tissue sections, tissues were classified as malignant and benign. Serum samples were collected within 1 week of the first biopsy-proven mammary tumour diagnosis, and prior to removal of the tumour by a surgical procedure or start of chemotherapy regime. [Details for histopathological classification & grading of tumour tissues are provided in Supplementary TableĀ S1. Representative photographs for histopathological analysis of tumour tissues are also shown in Supplementary Fig.Ā S1].
Immunohistochemistry
Tissue samples were fixed, paraffin-embedded and then cut into 5āµm sections. Sections were mounted on 3-aminopropyl-triethoxy-silane (APTES) coated slides, and air-dried overnight at 37ā°C. Prepared slides were deparaffinized in three washes of xylene (10āmin each), and rehydrated in graded concentrations of ethanol. The slides were then washed with PBS and blocked with 5% horse serum for 1āhour and further steps were carried as per SuperPicture⢠polymer detection Kit (Thermofischer Scientific, USA) with slight modifications. Briefly, 100āμl of quenching solution was added to completely cover the tissue sections for 5āminutes, followed by three rinses in PBS. The sections were then incubated overnight with 1:50 dilution of protein specific antibody. [PGAM1 (#SC-292579, Santa Cruz USA), TPI (#SC-30145, Santa Cruz USA), MNSOD (#SC-137254, Santa Cruz USA), CMYC (#SC-788, Santa Cruz USA), MUC1 (#AV41445, Sigma Aldrich USA)]. Post incubation, slides were washed with PBST (PBS with 0.05% Tween-20) and HRP polymer conjugate was added to completely cover the sections for 30āminutes. DAB chromogen was then added and incubated for 5āminutes followed by washing in distilled water. The sections were counter-stained using Mayerās hematoxylin for 10ā15āseconds. The control used for IHC was process control/isotype control wherein IHC was performed using isotype-specific immunoglobulins at the same concentration as of primary antibody, with rest of the procedure similar to test. To determine overexpression of the candidate biomarkers, IHC staining was compared between CMT and healthy mammary tissues.
Real-time PCR
The primers were designed for qRT-PCR analysis using the Integrated DNA technologies- Primer Quest Tool. The details of primers sequences used for the study are mentioned in TableĀ 1. Total RNA was extracted from tumour tissues preserved in RNAlater (Ambion, Life Technologies) as described previously48. The cDNA was synthesized using Revert Aid First Strand cDNA synthesis kit (Thermofischer Scientific, USA) according to the manufacturerās instructions and qRT-PCR was performed using Applied Biosystems 7500 Fast system as described previously48. Gene expression in each sample was normalized against the expression of housekeeping gene (β-actin). The relative expression of each sample was calculated using the 2āĪĪCT method with healthy mammary tissue as calibrator and log2 fold change was plotted.
Generation of recombinant expression vectors and expression of halo-tagged fusion proteins
One microgram of the total RNA sample was used to synthesize cDNA using Revertaid cDNA synthesis kit (ThermoScientific, USA) according to the manufacturerās protocol. Primers were designed for amplification of immunodominant regions from the target genes using Primer Express Software v 3.0.1(Life Technologies, USA). (Primer details are provided in Supplementary TableĀ S2). The PCR products were cloned individually in pH6HTN His6HaloTagĀ® T7 prokaryotic expression vector (Promega, USA). The recombinant clones were confirmed by PCR, restriction endonuclease analysis and plasmid DNA sequencing. Recombinant plasmids were transformed individually in E.coli KRX cells (Promega, USA). The transformed colonies were inoculated into LB medium containing 100 ug/ml ampicillin, and induced using 0.1% rhamnose and 1āmM isopropy-β-D-thiogalactopyranoside (IPTG) and purified by affinity chromatography using AKTA pure 25āM Fast Performance Liquid Chromatography (FPLC) (GE healthcare, Sweden) as described earlier49. The purified proteins were characterized by SDS-PAGE and western blotting.
Immobilization of recombinant proteins on MagPlex microspheres
Covalent immobilization of the expressed recombinant proteins on MagPlex microspheres was accomplished using halotag coupling strategy. For coupling, the recombinant TAAs were expressed as a fusion protein with an engineered haloalkane dehalogenase (Halo protein), employing pH6HTN His6HaloTagĀ® T7 prokaryotic expression vector (Promega, USA), as described earlier. The strategy for immobilization of halo-tagged recombinant TAAs on MagPlex microspheres is depicted in Fig.Ā 1. Halo-tagged recombinant proteins were immobilized on MagPlex microspheres (BioRad, USA) by first conjugating HaloTag amine (O4) ligand to the beads, using amine coupling strategy. A total of 1.25āĆā106 beads were conjugated with 0.2āmg of halo amine (O4) ligand as per the protocol of Jia et al.50. The microspheres were suspended in 100āmmol/L solution of 2-(N-morpholino)ethanesulfonic acid (MES) [pH 6.0], containing 5āmg/mL 1-ethyl-3-(3-dimethylaminopropyl) carbodiimide and 0.2āmg halo amine ligand. After incubation for 2 hrs, the microspheres were washed and re-suspended in 100āmmol/L MES (pH 4.5), and stored at 4ā°C. Further the halo protein tagged recombinant TAAs were immobilized on the halo amine ligand coupled microspheres. Different MagPlex bead regions were coupled overnight on rotator at 4ā°C with 5āµg of different HaloTag recombinant proteins. Post incubation, beads were washed in bead wash buffer (BioRad, USA) and re-suspended in 150āµl of storage buffer (BioRad, USA). The coupling between halo-tagged protein (HTP) and HaloTagĀ® amine (O4) ligand is based on the nucleophilic attack by the chloroalkane to Asp 106 in the HTP resulting in the formation of an ester bond between the HaloTag ligand and the HTP. HTP contains a critical mutation in the catalytic triad (His 272 to Phe) so that the ester bond formed between HTP and HaloTag ligand cannot be further hydrolysed. Therefore, the bonding is highly specific and essentially irreversible, resulting in formation of a complex on microspheres that is highly stable even under stringent conditions.
Strategy for coupling of halotagged recombinant TAAs on MagPlex microspheres. Halotagged recombinant proteins (HTP) were immobilized on MagPlex microspheres (BioRad, USA) by first conjugating HaloTag Amine (O4) ligand to the microspheres, using amine coupling strategy. The halo ligand immobilized magnetic micropsheres were then coupled to HTP. The coupling between HTP and HaloTagĀ® Amine (O4) ligand was based upon the nucleophilic attack by the chloroalkane to Asp 106 in the HTP, resulting in the formation of an ester bond between the HaloTag ligand and the recombinant protein. HTP contains a critical mutation in the catalytic triad (His 272 to Phe) so that the ester bond formed between HTP and HaloTag ligand cannot be further hydrolysed.
Validation and standardization of coupling on MagPlex microspheres
Considering that coupled microspheres could be cleared during the washing steps, concentration of coupled microspheres was determined by performing a total bead count for each region using Neubauer counting chamber. For confirmation of coupling of recombinant proteins on the microspheres, rabbit polyclonal antibodies against different TAAs were used. The protein coupled microspheres were resuspended by brief vortexing for approximately 20āseconds and a working microsphere mixture was prepared by diluting the coupled microsphere stocks to a final concentration of 1000 microspheres per well in PBS buffer. Tenfold serial dilutions of each antibody were incubated with different protein conjugated microspheres in a 96-well plate for 1āhour. After washing with wash buffer [PBS with 1% BSA & 0.02% Tween-20], the microspheres were incubated with biotinylated secondary antibody (4āµg/ml) for 1āhr. The resulting complex was washed again and incubated with Streptavidin-Phycoerythrin (S-PE) for 10āminutes. Finally, the micropsheres were washed and resuspended in assay buffer [PBS with 0.1% BSA and 0.02% Tween] and read on Bio-plex 200 system (Biorad, USA). Background corrected mean florescence intensity (MFI) was recorded, which indicated the binding signal. To ensure that individual protein coupled beads reacts with corresponding antibodies only, the specificity of the multiplex assay was tested by equally mixing different protein coupled microspheres (PGAM1, TPI, MNSOD, MUC1 and CMYC) and distributing into a 96 well plate and reacting with individual protein specific rabbit polyclonal antibodies on the multiplex array. To compare uniplex and multiplex system, 12 randomly selected sera samples were diluted 1:200 in PBS/1% BSA. The serum samples were added in duplicates to wells containing single protein coupled microspheres, as well as, mixture of different protein coupled microspheres. MFI values for the randomly selected sera samples corresponding to the given biomarkers were then compared between the uniplex and multiplex systems. For determining the assay parallelism, known quantities of polyclonal antibodies against TAAs were spiked in healthy dog sera. Slopes obtained from spike concentration-response curve in healthy dog serum were compared with that of the standard polyclonal antibody concentration response curve using four-parameter logistic (4-PL) curve fitting. To assess, the assay performance, the reproducibility of the assay was examined by determining the inter-assay, as well as, intra-assay coefficients of variation (%CV). Intra-assay %CV values were calculated from the MFIs of all the three replicates in a single plate at each standard dilution point from representative serum samples. For calculation of inter-assay %CV, the antibody concentrations corresponding to the representative sera samples from three independent plate measurements were taken into consideration.
Detection of autoantibody biomarkers in dog sera
Different microspheres conjugated with recombinant proteins were mixed together and distributed into a 96-well plate (1000 microspheres/well) to detect corresponding autoantibodies in dog serum. The serum samples were diluted 1:200 in PBS with 1% BSA and incubated with the microspheres for 1āhour with shaking. Post incubation, microspheres were washed using wash buffer. Further, biotinylated anti-dog antibody [4āµg/ml] was added to the microsphere complex and incubated for 1āhour followed by washing with wash buffer. Then streptavidin-PE was added to the microspheres for 10āminutes. Finally, microspheres were washed, resuspended in 100āµl of the assay buffer and fed through the Bio-Plex⢠200 instrument following the manufacturerās instructions. The instrument was programmed to read MagPlex microspheres in regions 028, 035, 045, 054 and 064 for TPI, MNSOD, MUC1, PGAM1 and CMYC coupled microspheres, respectively. A minimum of 100 events per microsphere were read and the median value obtained for each reaction event per microsphere set was recorded. Unless otherwise stated, all samples were analysed in duplicate and average readings were taken into consideration. MFI values for all the samples were corrected for background levels. Schematic representation of strategy for developing multiplex immunoassay for detecting autoantibody biomarkers in dog sera samples is displayed in Fig.Ā 2.
Statistical analysis
As background fluorescence intensity between microsphere sets can vary, the background values for the limit-of-detection were measured specifically for the capture microsphere sets used for the assay and all MFI values were subjected to background correction. The correlation coefficients between uniplex and multiplex systems, as well as, between different autoantibody biomarkers were evaluated using Pearsonās correlation coefficient (r). The data sets were tested for normal distribution and found not to be normally distributed. Therefore, statistical significance was tested using the Mann-Whitney-Test. Significance was defined at pā<ā0.05. Data analysis was done using Bio-Plex Data Pro softwareTM, GraphPad Prism (version 7.0) and MedCalc (version 14.8.1) softwares. Receiver Operator Characteristic (ROC) curve analysis51 was performed using MedCalc software (version 14.8). Herein, the sensitivity of a test is its ability to determine the tumour cases correctly and is defined as the probability that a test result will be positive when the disease is present (true positive rate). To calculate sensitivity, number of cases in the canine mammary tumour group that test positive [True positive (TP)] and negative [False negative (FN)] were first calculated, and then sensitivity was calculated as: TP/(TPā+āFN). The specificity of a test is its ability to determine the healthy cases correctly and is defined as the probability that a test result will be negative when the disease is not present (true negative rate). To calculate specificity, the number of cases in the non-diseased (Healthy) group that test positive [False positive (FP)] and negative [True negative (TN)] were determined, and then specificity was calculated as: TN/(TNā+āFP). The cut-off value designating positive reactions was chosen as the mean MFI of the healthy seraā+ā2āSD.
Ethical Approval
All experimental procedures involving animals were in accordance with Breeding of and Experiments on Animals (Control and Supervision) Amendment Rules, Government of India, 2005. The experiments were conducted with due permission from the Institutional Animal Ethics Committee, Indian Veterinary Research Institute, Izatnagar and the Committee for the Purpose of Control and Supervision of Experiments on Animals (CPCSEA), Ministry of environment, forests and climate change, Govt of India.
Results
Overexpression of TPI, PGAM1, MUC1, MNSOD and CMYC in CMT tissues
Immunohistochemical analysis indicated the overexpression of TPI, MNSOD, PGAM1, MUC1 and CMYC in the mammary gland carcinoma (Fig.Ā 3AāF). Upon close examination, it was observed that PGAM1 showed moderate immunostaining in the tubular epithelia, while a strong expression of PGAM1 was observed in the myoepithelia. TPI presented strong cytoplasmic and nuclear immunostaining in both tubular epithelium and myoepithelium. Expression of MUC1 was strong in cytoplasm of tubular epithelium and inflammatory cells. MNSOD showed strong membrane immunostaining of the tubular epithelium while CMYC showed moderate nuclear reactivity in the myoepithelial components.
Overexpression of TPI, MNSOD, PGAM1, MUC1 & CMYC in canine mammary tumours. Immunohistochemical analysis at 10X (A1āE1) and 20X (A2āE2) revealed over expression of TPI (A1,A2), MNSOD (B1,B2), PGAM1 (C1,C2), MUC1 (D1,D2) and CMYC (E1,E2) in CMTs. F1(10X) & F2(20X) represent isotype controls. (G) qRT-PCR analysis also indicated overexpression of these biomarkers in CMT tissues.
qRT-PCR studies also revealed over-expression of PGAM1, MNSOD, TPI, MUC1 and CMYC in CMT tissues. A high level of concordance was observed between qRT-PCR and IHC for expression of MNSOD, TPI, MUC1 and CMYC in CMT tissues with correlation coefficient(r) values ranging between 0.79ā0.86 for all the biomarkers. The relative gene expression levels for these five genes as calculated by 2āĪĪCT method in malignant (nā=ā10) and benign CMT tissues (nā=ā10), as compared to healthy mammary gland tissues (nā=ā2) are depicted in Fig.Ā 3G. The mean expression levels of these genes (except PGAM1) were significantly (pā<ā0.05) higher in malignant CMT tissues in comparison to benign CMT tissues.
Characterization of halo-tagged fusion proteins and confirmation of their immobilization on magnetic beads
The halo tagged recombinant TAAs were produced as described earlier. Immunoblot analysis of the recombinant proteins showed that the proteins reacted specifically with protein specific antibodies, as well as, with anti-halo antibodies confirming the presence of halo tag [SDS-PAGE and immunoblot analysis of recombinant TAAs are presented in Supplementary Fig.Ā S2]. The immobilization of recombinant proteins on individual MagPlex microspheres was tested using protein specific antibodies. MFI signals corresponding to different concentration of antibodies (10-fold serial dilutions) were recorded. As depicted in Fig.Ā 4, the MFI signals increased with the increasing concentration of antibodies. The regression coefficient (R2) values were calculated as 1, 0.996, 0.999, 0.997 and 1 for PGAM1, TPI, MNSOD, MUC1 and CMYC, respectively.
Validation of the coupling of MagPlex microspheres with the recombinant halotag fused TAAs. The protein coupled microspheres were reacted individually with increasing dilution of corresponding protein specific antibodies. The MFI signals generated by individual protein coupled microspheres increased with increasing concentration of antibodies in concentration dependent fashion.
Analysis of assay performance characteristics
The specificity of the multiplex assay was tested by equally mixing the individually protein coupled microspheres (PGAM1, TPI, MNSOD, MUC1 and CMYC) and distributing into a 96 well plate. Upon reacting with corresponding protein-specific antibodies on the multiplex array, the antibodies were only detected by their corresponding proteins bound on different bead regions and all other microspheres produced the background MFI signals, indicating no cross-reactivity between the individual protein-coupled microspheres. FigureĀ 5A shows that only the PGAM1 microspheres reacted with the PGAM1 antibodies, resulting in significantly higher MFI values (pā<ā0.0001), than the background signals observed with the remaining four bead regions. Similar results were observed with other bead regions as well. Further, the correlation between uniplex and multiplex systems was calculated by comparing the autoantibody signals generated from randomly selected serum samples (nā=ā12) by both the systems. As shown in Fig.Ā 5B,C, correlation coefficient (Pearson, r) was 0.83 for PGAM1 and 0.84 for CMYC (pā<ā0.005), indicating a significant correlation between the uniplex and multiplex systems.
No cross-reactivity between individual protein-coupled microspheres in the multiplex system. (A) Multiplex system containing five microspheres coupled with TPI, MNSOD, PGAM1, MUC1 and CMYC was incubated with PGAM1 antibody, and only the PGAM1 microspheres reacted with anti-PGAM1 antibody, while other microspheres detected the background MFI values. (B) The signal correlation of the PGAM1 coupled microspheres in the singleplex and multiplex system. (C) Same as (B) but using CMYC coupled microspheres. The correlation between uniplex and multiplex systems was calculated by comparing the autoantibody signals generated from randomly selected serum samples (nā=ā12) by both the systems. The correlation coefficient (Pearson, r) was 0.83 for PGAM1 and 0.84 for CMYC (pā<ā0.005), indicating a significant correlation between the uniplex and multiplex systems.
Next, the assay parallelism was determined by spiking known quantities of polyclonal antibodies in healthy dog sera and then comparing slopes obtained from spike concentration-response curve in healthy dog serum with that of the standard polyclonal antibody concentration response curve, using four-parameter logistic (4-PL) curve fitting. The percentage differences in slope value with respect to standard curve were less than 18% for all the analytes in the panel.
To further assess, the assay performance, the reproducibility of the assay was examined by determining the inter-assay, as well as, intra-assay coefficients of variation (%CV). Intra-assay %CV values were calculated from the MFIs of replicates in a single plate at each standard dilution point from representative serum samples. All the analytes exhibited <7.4%CV. For calculation of inter-assay %CV, the antibody concentrations observed in the representative sera samples from three independent plate measurements were taken into consideration. The inter- assay %CV was found to be <9.6%.
Assay validation with clinical sera samples
The validity of an assay is defined as its ability to distinguish between diseased and healthy individuals. Thus, to determine assay validity, the multiplex assay, comprising a panel of 5 candidate autoantigens, was used for screening of circulating autoantibodies in total 125 dog sera samples, including 75 canine mammary tumour (CMT) sera and 50 healthy dog sera. At 1:200 dilution of sera samples; TPI, PGAM1, MUC1, MNSOD, & CMYC coupled microspheres, produced significantly higher average MFI signals in CMT sera, as compared to healthy dog sera samples (Mann-Whitney, p valueā<ā0.0001) (Fig.Ā 6AāE). Taking the cut-off value as average MFI of healthy seraā+ā2āSD, the individual assays were found to be highly specific, with specificities ranging from 94% to 98%. However, the frequencies of autoantibodies to single TAA were relatively low, leading to lower sensitivities ranging from 34.6 to 60% for the individual TAAs (Fig.Ā 6F). Among all TAA, sensitivity and specificity exhibited by MUC1 assay was found to be highest (Supplementary TablesĀ S3āS7). Comparison of heat maps of the MFI signals generated from CMT and healthy dog sera revealed that majority of CMT sera have MFI values higher than 2,500 for all the five biomarkers, while most of the healthy dog sera have MFI values below 2,500 (Fig.Ā 7). ROC curve analysis, showed that the area under the curve (AUC) of each TAA is more than 0.8 (Fig.Ā 8), indicating the ability of individual assays to discriminate between tumour sera and healthy dog sera. Upon comparison of ROC curves, the maximum AUC (0.92) was observed with MUC1. There were significant differences in AUC of MUC1 and other autoantibody biomarkers (pā<ā0.05) indicating the usefulness of anti-MUC1 antibodies in detection of canine mammary tumour. The Pearson correlation coefficient, r was found to be greater than 0.5 for all biomarkers, indicating significant (pā<ā0.0001) correlation among different TAAs (TableĀ 2). A maximum positive correlation coefficient (r) was observed between MNSOD and MUC1 biomarker (rā=ā0.75). Upon analysis of the expression patterns of these autoantibody biomarkers in different types (benign and malignant) and grades (IāIII) of canine mammary tumours, it was observed that these 5 autoantibody biomarkers were present across all tumour grades and types. A significant positive correlation (rā=ā0.25, pā<ā0.05) was observed for presence of malignancy and TPI biomarker in clinical cases of canine mammary tumour. Among malignant CMTs, autoantibodies to TPI, MUC1, PGAM1, CMYC and MNSOD, were present across all tumour grades. MUC1 antibodies were found to be associated with 80% of grade III cancers as compared to 50% of early grade cancers (including grade I & II cancers), indicating their role in aggressiveness of canine mammary tumours.
Comparison of MFI values corresponding to autoantibody response (against five TAAs) in 50 healthy and 75 canine mammary tumour sera. (AāE) Scatter diagrams representing CMYC, MNSOD, PGAM1, TPI & MUC1 autoantibody response in canine mammary tumour (CMT) sera (nā=ā75) and healthy dog sera (nā=ā50). (F) At 1:200 dilution of sera samples, the multiplex assay comprising of 5 autoantigens, namely TPI, PGAM1, MUC1, MNSOD, & CMYC coupled microspheres, produced significantly higher average MFI signals in CMT sera (nā=ā75) compared to healthy dog sera samples (nā=ā50) (Mann-Whitney, p valueā<ā0.0001). At cut-off value (avg. MFI of healthy seraā+ā2āSD), individual assays were found to be highly specific (96ā98% specificity). The bars represent average MFI values and error bars represent standard error.
Heat maps of the MFI signals generated from CMT group and healthy group Comparison of heat maps of the MFI signals obtained from CMT (nā=ā75) and healthy dog sera (nā=ā50) by the multiplex assay revealed that majority of CMT sera have MFI values higher than 2,500 for all the five biomarkers, while most of the healthy dog sera have MFI values below 2,500. Heat map scale indicates the range of MFI values.
ROC analysis for individual autoantibody biomarkers: The area under the curve (AUC) for each individual autoantibody biomarker is more than 0.8 indicating the ability of individual assays to discriminate between CMT (nā=ā75) and healthy dog sera (nā=ā50). Comparison of ROC curves reveals maximum AUC for MUC1 biomarker followed by CMYC & PGAM1.
Evaluation of diagnostic utility of the autoantibody biomarker panel in canine mammary tumour immunodiagnosis
Further, we determined the ability of the biomarker panel for detection of canine mammary tumours (CMTs). Out of 75 CMT sera samples analysed, 78.6% (59/75) had a detectable level of autoantibodies cumulatively to any of these five TAAs, which is significantly higher than the frequency in sera from healthy individuals (pā<ā0.001). The results depicted in Fig.Ā 9A clearly establish that, with the successive addition of TAAs in the multiplex panel to a total of 5, there is a stepwise increase in sensitivity reaching upto 78.6%. The MFI scores generated by all TAAs were analysed by ROC curve, which provides an index for testās accuracy by plotting the sensitivity against 1āspecificity for each result value of the test. Upon analysis it was observed that AUC of the combined panel of five biomarkers was 0.931 (pā<ā0.0001), greater than AUC for individual biomarkers, demonstrating the strong discriminative power of panel of 5 biomarkers. Comparison of ROC curves reveals that with increase in the number of biomarkers in the panel, there is also an increase in AUC, with maximum AUC of 0.931(pā<ā0.0001) for the combined panel of five biomarkers (Fig.Ā 9B). Considering the healthy average MFIā+ā2āSD as cut-off limit for individual biomarkers, the multiplex assay was found to be 78.6% sensitive and 90% specific (TableĀ 3). Further, no healthy sample was positive for more than 1 autoantibody biomarker above the cut-off limits, whereas 60% (45/75) of the tumour sera samples were positive for more than 1 biomarker. Thus, assuming the presence of more than 1 biomarkers above the cut-off limit as a criterion for positivity, the five-plex assay was 100% specific and 60% sensitive. To further address the question of how valuable is the approach of antibody detection to a panel of five TAAs in separating dogs with and without mammary tumours, a group of parameters, such as Youdenās index, positive and negative predictive value (+PV/āPV), etc, were calculated and summarized in TableĀ 3. The combined biomarker panel showed a positive predictive value of 87.88% and the negative predictive value of 71.19%. Taken together, these data show the usefulness of the multiplex assay in increasing the clinical diagnostic quality and value for mammary cancer diagnosis in dogs.
Diagnostic value of different autoantibody biomarker panels for canine mammary tumour: (A) With successive addition of TAAs to a total of 5 antigens in the multiplex assay, there is a stepwise increase in sensitivity. Out of 75 CMT sera samples analysed, 78.67% showed detectable level of autoantibodies cumulatively to any of these five TAAs, which was significantly higher than the frequency in sera from healthy individuals(nā=ā50) (pā<ā0.001). (B) Comparison of ROC curves reveals that with increase in the number of biomarkers in the panel, there is also an increase in AUC, with maximum AUC of 0.931(pā<ā0.0001) for the combined panel of five biomarkers.
Discussion
Exploitation of the immunological responses evoked against tumour associated autoantigens (TAAs) is an emerging strategy for developing new tools for non-invasive detection of cancers. Assay based on demonstration of anti-TAA antibodies in sera of patients could be of great importance for early detection of cancer because detectable amount of antibodies against TAA are formed well before the tumour phenotype arises2,52,53,54,55,56,57,58. Further, detection of autoantibodies in sera of animals is more reliable than detection of TAAs, which are not always present in sera in detectable levels and are relatively unstable as they can degrade with time in comparison to antibodies1,2,4.
Mammary cancer results from the derailment of multiple cell signalling pathways and regulatory processes. Thus, by elucidation of a single biomarker, accurate diagnosis of the disease cannot be made and multiple biomarkers need to be identified. Research in the past few years have established the fact that multiplexing autoantibody biomarkers could lead to significant increase in sensitivity and specificity for cancer detection24,25,50,58,59,60,61. Therefore, the aim of this study was to develop a multiplex assay for detecting autoantibodies against a panel of five TAAs in clinical cases of canine mammary tumour, which is well established as a model for human breast cancer studies. Three TAAs, including MNSOD, TPI, and PGAM1, were selected as potential autoantigens for canine mammary tumour panel based on findings of Zamani-Ahmadmahmudi et al.39. Autoantibodies against other 2 autoantigens namely CMYC and MUC1 were selected as biomarkers for panel assay based on the performance of in-house developed ELISA in distinguishing canine mammary tumours from healthy controls (Supplementary TableĀ S8).
The combined AUC for the panel of biomarkers used for magnetic bead based assay is 0.931 (pā<ā0.0001), which clearly reflects the ability of the five-plex assay in discriminating dog mammary tumour patients and healthy controls. Further, the assay could be conducted in 3āhours using only one microliter of serum sample and could detect clinical cases of dog mammary tumour with sensitivity and specificity of 78.6% and 90%, respectively. In this study, we have reported for the first time a multiplexed assay for detection of autoantibodies in canine tumours, utilizing luminex technology and halo-tag coupling strategy. An interesting feature of this study was that with an increase in the number of autoantibody biomarkers in the multiplex immunoassay, the likelihood of detecting antibody in the serum samples increased. The likelihood of detecting CMT (95% confidence interval for sensitivity) was 24.04ā46.54% when only 1 biomarker (CMYC) was used, which increased to 66.21ā86.21% when five biomarkers were used. Further, no healthy sample had more than 1 autoantibody biomarker above the cut-off limits, whereas 60% (45/75) of the tumour sera samples were positive for more than 1 biomarker. Thus, assuming the presence of more than one biomarker above the cut-off limit as a criterion for positivity, the five-plex assay is 100% specific and 60% sensitive. Similar results were observed in a human breast cancer study, where researchers have found that successive addition of TAAs to a total of six antigens, led to a stepwise increase in positive antibody reactions reaching a sensitivity of 67.3% and specificity of 92.2%59,60,61. Therefore, both the sensitivity and specificity of the assay could be improved by expanding the autoantibody panel to include more autoantibodies which might be more selectively associated with canine mammary tumour. For this, more autoantibody biomarker candidates associated with canine mammary tumour needs to be identified, as only a few autoantibody biomarkers have been reported so far in dogs. Several studies have reported that dog and human breast cancer share common tumour antigens. Therefore, based on leads from human cancer studies, we have identified autoantibodies to CMYC and MUC1 in canine tumours. In future, more efforts need to be diverted towards identification of autoantibody biomarkers in canines.
To conclude, the multiplex autoantibody assay holds great promise for canine mammary tumour diagnosis. To adapt the technique for mass screening, a detailed follow-up study needs to be conducted with more number of samples, with different tumour types and stages to further validate the performance of the autoantibodies across tumour histology and type. This multiplex luminex assay could be envisaged for screening of the high-risk population with subsequent confirmatory tests. Due to the similarity of tumour proteome profile in dogs with that of humans, the canine mammary tumour serves as an excellent model for studying human cancer biology and therapy. Therefore, further investigations with a number of samples are required to determine the efficacy of these serum biomarkers for early diagnosis or prognosis of canine, as well as, human mammary cancer.
Data Availability statement
All the relevant data pertaining to the study shall be made available upon request.
References
Old, L. J. & Chen, Y. T. New paths in human cancer serology. J. Exp. Med. 187, 1163ā7 (1998).
Tan, E. M. Autoantibodies as reporters identifying aberrant cellular mechanisms in tumorigenesis. J. Clin. Invest. 108(10), 1411 (2001).
Wang, X. et al. Autoantibody signatures in prostate cancer. N. Engl. J. Med. 353(12), 1224ā35 (2005).
Tan, E. M. & Zhang, J. Autoantibodies to tumorāassociated antigens: reporters from the immune system. Immunol. Rev. 222, 328ā40 (2008).
Scanlan, M. J. et al. Characterization of human colon cancer antigens recognized by autologous antibodies. Int. J. Cancer. 76, 652ā8 (1998).
Disis, M. L. et al. Existent T-cell and antibody immunity to HER-2/neu protein in patients with breast cancer. Cancer Res. 54, 16ā20 (1994).
Zhong, L. et al. Identification of circulating antibodies to tumorāassociated proteins for combined use as markers of nonāsmall cell lung cancer. Proteomics. 4, 1216ā25 (2004).
Chatterjee, M. et al. Diagnostic markers of ovarian cancer by high-throughput antigen cloning and detection on arrays. Cancer Res. 66, 1181ā90 (2006).
Lin, H. S. et al. Autoantibody approach for serum-based detection of head and neck cancer. Cancer Epidemiol. Biomarkers Prev. 16, 2396ā405 (2007).
Zhou, J. H., Zhang, B., Kernstine, K. H. & Zhong, L. Autoantibodies against MMP-7 as a novel diagnostic biomarker in esophageal squamous cell carcinoma. World J. Gastroenterol. 17(10), 1373 (2011).
Hamanaka, Y. Circulating antiāMUC1 IgG antibodies as a favorable prognostic factor for pancreatic cancer. Int. J. Cancer. 103(1), 97ā100 (2003).
Anderson, K. S. & LaBaer, J. The sentinel within: exploiting the immune system for cancer biomarkers. J. Proteome Res. 4(4), 1123ā33 (2005).
Casiano, C. A., Mediavilla-Varela, M. & Tan, E. M. Tumor-associated antigen arrays for the serological diagnosis of cancer. Mol. Cell Proteomics. 5(10), 1745ā59 (2006).
Lu, H., Goodell, V. & Disis, M. L. Humoral immunity directed against tumor-associated antigens as potential biomarkers for the early diagnosis of cancer. J. Proteome Res. 7(4), 1388ā94 (2008).
Yao, Y. Potential application of non-small cell lung cancer-associated autoantibodies to early cancer diagnosis. Biochem. Biophys. Res. Commun. 423(3), 613ā9 (2012).
Bei, R., Masuelli, L., Palumbo, C., Modesti, M. & Modesti, A. A common repertoire of autoantibodies is shared by cancer and autoimmune disease patients: Inflammation in their induction and impact on tumor growth. Cancer Lett. 281(1), 8ā23 (2009).
Kellar, K. L., Mahmutovic, A. J. & Bandyopadhyay, K. Multiplexed MicrosphereāBased Flow Cytometric Immunoassays. Curr. Protoc. Cytom. 35, 13ā21 (2006).
Reslova, N. xMAP Technology: Applications in Detection of Pathogens. Front. Microbiol. 8 https://doi.org/10.3389/fmicb.2017.00055 (2017).
Elshal, M. F. & McCoy., J. P. Multiplex bead array assays: performance evaluation and comparison of sensitivity to ELISA. Methods. 38(4), 317ā23 (2006).
Egenvall, A. et al. Incidence of and survival after mammary tumors in a population of over 80,000 insured female dogs in Sweden from 1995 to 2002. Prev. Vet. Med. 69, 109ā27 (2005).
Chapman, C. Autoantibodies in breast cancer: their use as an aid to early diagnosis. Ann. Onco. 18(5), 868ā73 (2007).
von Mensdorff-Pouilly, S. et al. Survival in early breast cancer patients is favorably influenced by a natural humoral immune response to polymorphic epithelial mucin. J. Clin. Oncol. 18(3), 574ā583 (2000).
Lu, H. et al. Evaluation of known oncoantibodies, HER2, p53, and cyclin B1, in prediagnostic breast cancer sera. Cancer Prev. Res. 5(8), 1036ā43 (2012).
Patz, E. F. Jr. et al. Panel of serum biomarkers for the diagnosis of lung cancer. J. Clin. Oncol. 25(35), 5578ā83 (2007).
Zhu, C. S. et al. A framework for evaluating biomarkers for early detection: validation of biomarker panels for ovarian cancer. Cancer Prev. Res. 4(3), 375ā83 (2011).
Lincet, H. & Icard, P. How do glycolytic enzymes favour cancer cell proliferation by nonmetabolic functions? Oncogene. 34(29), 3751 (2015).
Hart, P. C. et al. MnSOD upregulation sustains the Warburg effect via mitochondrial ROS and AMPK-dependent signalling in cancer. Nat. Commun. 6, 6053, https://doi.org/10.1038/ncomms7053. (2015).
Kattan, Z., Minig, V., Leroy, P., DauƧa, M. & Becuwe, P. Role of manganese superoxide dismutase on growth and invasive properties of human estrogen-independent breast cancer cells. Breast Cancer Res. Treat. 108(2), 203ā215 (2008).
Ennen, M. et al. Regulation of the high basal expression of the manganese superoxide dismutase gene in aggressive breast cancer cells. Free Radic. Biol. Med. 50(12), 1771ā9 (2011).
Rakha, E. A. Expression of mucins (MUC1, MUC2, MUC3, MUC4, MUC5AC and MUC6) and their prognostic significance in human breast cancer. Mod. Pathol. 18(10), 1295ā304 (2005).
Rahn, J. J., Dabbagh, L., Pasdar, M. & Hugh, J. C. The importance of MUC1 cellular localization in patients with breast carcinoma. Cancer 91(11), 1973ā1982 (2001).
Horm, T. M. & Schroeder, J. A. MUC1 and metastatic cancer: expression, function and therapeutic targeting. Cell Adh. Migr. 7(2), 187ā198, https://doi.org/10.4161/cam.23131 (2013).
Pelengaris, S., Khan, M. & Evan, G. c-MYC: more than just a matter of life and death. Nat. Rev. Cancer. 2, 764ā76 (2002).
Dang, C. V. MYC on the Path to Cancer. Cell. 149(1), 22ā35 (2012).
Borge, K. S. Canine mammary tumours are affected by frequent copy number aberrations, including amplification of MYC and loss of PTEN. PloS One. 10(5), e0126371, https://doi.org/10.1371/journal.pone.0126371 (2015).
Fu, A. et al. High expression of MnSOD promotes survival of circulating breast cancer cells and increases their resistance to doxorubicin. Oncotarget. 7(31), 50239, https://doi.org/10.18632/oncotarget.10360 (2016).
Hay, N. Reprogramming glucose metabolism in cancer: can it be exploited for cancer therapy? Nat. Rev. Cancer. 16(10), 635 (2016).
Zhang, D. et al. Phosphoglycerate mutase 1 promotes cancer cell migration independent of its metabolic activity. Oncogene. 36(20), 2900ā9 (2017).
ZamaniāAhmadmahmudi, M., Nassiri, S. M. & Rahbarghazi, R. Serological proteome analysis of dogs with breast cancer unveils common serum biomarkers with human counterparts. Electrophoresis. 35(6), 901ā10 (2014).
Yang, F. et al. Identification of tumor antigens in human lung squamous carcinoma by serological proteome analysis. J. Proteome Res. 6(2), 751ā8 (2007).
Tamesa, M. S. et al. Detection of autoantibodies against cyclophilin A and triosephosphate isomerase in sera from breast cancer patients by proteomic analysis. Electrophoresis. 30(12), 2168ā81 (2009).
Mojtahedi, Z., Safaei, A., Yousefi, Z. & Ghaderi, A. Immunoproteomics of HER2-positive and HER2-negative breast cancer patients with positive lymph nodes. OMICS. 15(6), 409ā18 (2011).
Tang, Y. et al. Detection of circulating anti-mucin 1 (MUC1) antibodies in breast tumor patients by indirect enzyme-linked immunosorbent assay using a recombinant MUC1 protein containing six tandem repeats and expressed in Escherichia coli. Clin. Vaccine Immunol. 17(12), 1903ā8 (2010).
Hamrita, B. et al. Identification of tumor antigens that elicit a humoral immune response in breast cancer patientsā sera by serological proteome analysis (SERPA). Clinica. Chimica. Acta. 393(2), 95ā102 (2008).
Li, P. et al. Serum anti-MDM2 and anti-c-Myc autoantibodies as biomarkers in the early detection of lung cancer. OncoImmunology. 5(5), e1138200 (2016).
Blixt, O. et al. Autoantibodies to aberrantly glycosylated MUC1 in early stage breast cancer are associated with a better prognosis. Breast Cancer Res. 13(2), R25, https://doi.org/10.1186/bcr2841 (2011).
Goldschmidt, M., Pena, L., Rasotto, R. & Zappulli, V. Classification and grading of canine mammary tumors. Vet. Pathol. 48(1), 117ā31 (2011).
Jena, S. C. et al. Sequence Characterization of baculoviral inhibitor of apoptosis repeat containing 5 (BIRC 5) gene from a case of canine mammary tumour. Asian J. Anim. Vet. Adv. 10(8), 394ā405 (2015).
Arora, R. et al. Genetic characterization and expression analysis of recombinant manganese superoxide dismutase (MnSOD) from spontaneously occurring canine mammary tumor. . Adv Anim Vet Sci. 4(7), 363ā369 (2016).
Jia, J. et al. Development of a multiplex autoantibody test for detection of lung cancer. PloS one. 9(4), e95444; 0.1371/journal.pone.0095444 (2014).
Greiner, M., Pfeiffer, D. & Smith, R. D. Principles and practical application of the receiver-operating characteristic analysis for diagnostic tests. Prev. Vet. Med. 45(1), 23ā41 (2000).
Tan, H. T., Low, J., Lim, S. G. & Chung, M. Serum autoantibodies as biomarkers for early cancer detection. FEBS. J. 276(23), 6880ā904 (2009).
Dorn, C., Knobloch, C., Kupka, M., Morakkabati-Spitz, N. & Schmolling, J. Paraneoplastic neurological syndrome: patient with anti-Yo antibody and breast cancer: a case report. Arch. Gynecol. Obste. 269(1), 62ā5 (2003).
Folli, F. et al. Autoantibodies to a 128-kd synaptic protein in three women with the stiff-man syndrome and breast cancer. N. Engl. J Med. 328(8), 546ā51 (1993).
Pittock, S. J., Lucchinetti, C. F. & Lennon, V. A. Antiāneuronal nuclear autoantibody type 2: paraneoplastic accompaniments. Ann. Neurol. 53(5), 580ā7 (2003).
Tomkiel, J. E. et al. Autoimmunity to the Mr 32,000 subunit of replication protein A in breast cancer. Clin. Cancer Res. 8(3), 752ā8 (2002).
Lacombe, J. Identification and validation of new autoantibodies for the diagnosis of DCIS and node negative earlyāstage breast cancers. Int. J. Cancer. 132(5), 1105ā13 (2013).
Desmetz, C., Mange, A., Maudelonde, T. & Solassol, J. Autoantibody signatures: progress and perspectives for early cancer detection. J. Cell Mol. Med. 15(10), 2013ā24 (2011).
MangĆ©, A. Serum autoantibody signature of ductal carcinoma in situ progression to invasive breast cancer. Clin Cancer Res. 18(7), 1992ā2000 (2012).
Liu, W. et al. Detection of autoantibodies to multiple tumor-associated antigens (TAAs) in the immunodiagnosis of breast cancer. Tumor Biol. 36(2), 1307ā12 (2015).
Zhong, L. et al. Autoantibodies as potential biomarkers for breast cancer. Breast Cancer Res. 10(3), R40 (2008).
Acknowledgements
The authors are thankful to Director ICAR-IVRI, Izatnagar for providing the necessary infrastructure facilities to carry out the research work. This work is a part of the PhD thesis of first author. This study was supported by the grants received from ICAR-IVRI (IVRI/BIOTECH/15-18/010), ICAR-Niche Area of Excellence Programme on Biosensors and Department of Biotechnology (DBT), Govt. of India (Grant No. BT/ADV/CANINE HEALTH/GADVASU/2017-18).
Author information
Authors and Affiliations
Contributions
Shahid Hussain, Sonal Saxena and Sameer Shrivastava contribute equally for the study. Shahid Hussain, Sonal Saxena and Sameer Shrivastava designed the study, conducted the experiments, analyzed data and prepared the manuscript. Richa Arora, Rajkumar James Singh and Subas Chandra Jena conducted the experiments related to cloning, expression and purification of recombinant proteins. Naveen Kumar collected the tumour tissue & serum samples and provided clinical diagnosis of the disease. Monalisa Sahoo and Anil Kumar Sharma performed histopathological and IHC studies. A.K. Tiwari, B.P. Mishra and Raj Kumar Singh contributed in data analysis & editing the manuscript.
Corresponding authors
Ethics declarations
Competing Interests
The authors declare no competing interests.
Additional information
Publisherās note: Springer Nature remains neutral with regard to jurisdictional claims in published maps and institutional affiliations.
Electronic supplementary material
Rights and permissions
Open Access This article is licensed under a Creative Commons Attribution 4.0 International License, which permits use, sharing, adaptation, distribution and reproduction in any medium or format, as long as you give appropriate credit to the original author(s) and the source, provide a link to the Creative Commons license, and indicate if changes were made. The images or other third party material in this article are included in the articleās Creative Commons license, unless indicated otherwise in a credit line to the material. If material is not included in the articleās Creative Commons license and your intended use is not permitted by statutory regulation or exceeds the permitted use, you will need to obtain permission directly from the copyright holder. To view a copy of this license, visit http://creativecommons.org/licenses/by/4.0/.
About this article
Cite this article
Hussain, S., Saxena, S., Shrivastava, S. et al. Multiplexed Autoantibody Signature for Serological Detection of Canine Mammary Tumours. Sci Rep 8, 15785 (2018). https://doi.org/10.1038/s41598-018-34097-0
Received:
Accepted:
Published:
DOI: https://doi.org/10.1038/s41598-018-34097-0
Keywords
Comments
By submitting a comment you agree to abide by our Terms and Community Guidelines. If you find something abusive or that does not comply with our terms or guidelines please flag it as inappropriate.