Abstract
Skeletal muscle undergoes marked functional decay during aging in humans, but the cell biological mechanisms responsible for this process are only partly known. Age-related muscle dysfunction is also a feature of aging in the fruit fly Drosophila melanogaster. Here we describe a detailed step-by-step protocol, which takes place over 3 d, for whole-mount immunostaining of Drosophila flight muscle. The skeletal muscle is fixed and permeabilized without any tissue freezing and dehydration so that antigens are accessible for staining with appropriate antibodies and the overall tissue ultrastructure is well preserved. This technique can be used to identify age-related cellular changes driving skeletal muscle aging and for characterizing models of human muscle disease in Drosophila.
This is a preview of subscription content, access via your institution
Access options
Subscribe to this journal
Receive 12 print issues and online access
$259.00 per year
only $21.58 per issue
Buy this article
- Purchase on Springer Link
- Instant access to full article PDF
Prices may be subject to local taxes which are calculated during checkout


Similar content being viewed by others
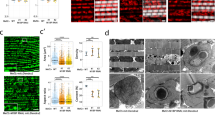
References
Nair, K.S. Aging muscle. Am. J. Clin. Nutr. 81, 953–963 (2005).
Demontis, F., Piccirillo, R., Goldberg, A.L. & Perrimon, N. Mechanisms of skeletal muscle aging: insights from Drosophila and mammalian models. Dis. Model Mech. 10.1242/dmm.012559 (2013).
Demontis, F., Piccirillo, R., Goldberg, A.L. & Perrimon, N. The influence of skeletal muscle on systemic aging and lifespan. Aging Cell 10.1111/acel.12126 (2013).
Miller, M.S. et al. Aging enhances indirect flight muscle fiber performance yet decreases flight ability in Drosophila. Biophys. J. 95, 2391–2401 (2008).
Rhodenizer, D., Martin, I., Bhandari, P., Pletcher, S.D. & Grotewiel, M. Genetic and environmental factors impact age-related impairment of negative geotaxis in Drosophila by altering age-dependent climbing speed. Exp. Gerontol. 43, 739–748 (2008).
Kenyon, C.J. The genetics of ageing. Nature 464, 504–512 (2010).
Bate, M. The embryonic development of larval muscles in Drosophila. Development 110, 791–804 (1990).
Demontis, F. & Perrimon, N. Integration of insulin receptor/Foxo signaling and dMyc activity during muscle growth regulates body size in Drosophila. Development 136, 983–993 (2009).
Abmayr, S.M., Erickson, M.S. & Bour, B.A. Embryonic development of the larval body wall musculature of Drosophila melanogaster. Trends Genet. 11, 153–159 (1995).
Morriss, G.R. et al. Analysis of skeletal muscle development in Drosophila. Methods Mol. Biol. 798, 127–152 (2012).
Dutta, D., Anant, S., Ruiz-Gomez, M., Bate, M. & VijayRaghavan, K. Founder myoblasts and fibre number during adult myogenesis in Drosophila. Development 131, 3761–3772 (2004).
Metzger, T. et al. MAP and kinesin-dependent nuclear positioning is required for skeletal muscle function. Nature 484, 120–124 (2012).
Cripps, R.M., Lovato, T.L. & Olson, E.N. Positive autoregulation of the myocyte enhancer factor-2 myogenic control gene during somatic muscle development in Drosophila. Dev. Biol. 267, 536–547 (2004).
Gilsohn, E. & Volk, T. A screen for tendon-specific genes uncovers new and old components involved in muscle-tendon interaction. Fly 4, 149–153 (2010).
Maqbool, T. & Jagla, K. Genetic control of muscle development: learning from Drosophila. J. Muscle Res. Cell Motil. 28, 397–407 (2007).
Schonbauer, C. et al. Spalt mediates an evolutionarily conserved switch to fibrillar muscle fate in insects. Nature 479, 406–409 (2011).
Piccirillo, R., Demontis, F., Perrimon, N. & Goldberg, A.L. Mechanisms of muscle growth and atrophy in mammals and Drosophila. Dev. Dyn. 10.1002/dvdy.24036 (2013).
Kucherenko, M.M. et al. Paraffin-embedded and frozen sections of Drosophila adult muscles. J. Vis. Exp. 46, e2438 (2010).
Llamusi, B. et al. Muscleblind, BSF and TBPH are mislocalized in the muscle sarcomere of a Drosophila myotonic dystrophy model. Dis. Model Mech. 6, 184–196 (2013).
Mukherjee, P., Gildor, B., Shilo, B.Z., VijayRaghavan, K. & Schejter, E.D. The actin nucleator WASp is required for myoblast fusion during adult Drosophila myogenesis. Development 138, 2347–2357 (2011).
Pantoja, M., Fischer, K.A., Ieronimakis, N., Reyes, M. & Ruohola-Baker, H. Genetic elevation of sphingosine 1-phosphate suppresses dystrophic muscle phenotypes in Drosophila. Development 140, 136–146 (2013).
Demontis, F. & Dahmann, C. Characterization of the Drosophila ortholog of the human Usher Syndrome type 1G protein sans. PLoS ONE 4, e4753 (2009).
Perkins, A.D. et al. Integrin-mediated adhesion maintains sarcomeric integrity. Dev. Biol. 338, 15–27 (2010).
Pitsouli, C. & Perrimon, N. The homeobox transcription factor cut coordinates patterning and growth during Drosophila airway remodeling. Sci. Signal. 6, ra12 (2013).
Schlichting, K., Wilsch-Brauninger, M., Demontis, F. & Dahmann, C. Cadherin Cad99C is required for normal microvilli morphology in Drosophila follicle cells. J. Cell Sci. 119, 1184–1195 (2006).
Demontis, F. & Perrimon, N. FOXO/4E-BP signaling in Drosophila muscles regulates organism-wide proteostasis during aging. Cell 143, 813–825 (2010).
Brandt, A., Krohne, G. & Grosshans, J. The farnesylated nuclear proteins KUGELKERN and LAMIN B promote aging-like phenotypes in Drosophila flies. Aging Cell 7, 541–551 (2008).
Schuster, C.M., Davis, G.W., Fetter, R.D. & Goodman, C.S. Genetic dissection of structural and functional components of synaptic plasticity. I. Fasciclin II controls synaptic stabilization and growth. Neuron 17, 641–654 (1996).
Gajewski, K.M. & Schulz, R.A. CF2 represses Actin 88F gene expression and maintains filament balance during indirect flight muscle development in Drosophila. PLoS ONE 5, e10713 (2010).
Budnik, V. et al. Regulation of synapse structure and function by the Drosophila tumor suppressor gene dlg. Neuron 17, 627–640 (1996).
Bryantsev, A.L., Baker, P.W., Lovato, T.L., Jaramillo, M.S. & Cripps, R.M. Differential requirements for myocyte enhancer factor-2 during adult myogenesis in Drosophila. Dev. Biol. 361, 191–207 (2012).
Bai, J., Hartwig, J.H. & Perrimon, N. SALS, a WH2-domain-containing protein, promotes sarcomeric actin filament elongation from pointed ends during Drosophila muscle growth. Dev. Cell 13, 828–842 (2007).
Clyne, P.J., Brotman, J.S., Sweeney, S.T. & Davis, G. Green fluorescent protein tagging Drosophila proteins at their native genomic loci with small P elements. Genetics 165, 1433–1441 (2003).
Buszczak, M. et al. The Carnegie protein trap library: a versatile tool for Drosophila developmental studies. Genetics 175, 1505–1531 (2007).
Quinones-Coello, A.T. et al. Exploring strategies for protein trapping in Drosophila. Genetics 175, 1089–1104 (2007).
Acknowledgements
F.D. is supported by funding from the American Lebanese Syrian Associated Charities (ALSAC) and the Ellison Medical Foundation New Scholar in Aging award.
Author information
Authors and Affiliations
Contributions
F.D. and L.C.H. wrote the manuscript.
Corresponding author
Ethics declarations
Competing interests
The authors declare no competing financial interests.
Rights and permissions
About this article
Cite this article
Hunt, L., Demontis, F. Whole-mount immunostaining of Drosophila skeletal muscle. Nat Protoc 8, 2496–2501 (2013). https://doi.org/10.1038/nprot.2013.156
Published:
Issue Date:
DOI: https://doi.org/10.1038/nprot.2013.156
This article is cited by
-
Fluvastatin-induced myofibrillar damage is associated with elevated ROS, and impaired fatty acid oxidation, and is preceded by mitochondrial morphological changes
Scientific Reports (2024)
-
An adaptive stress response that confers cellular resilience to decreased ubiquitination
Nature Communications (2023)
-
N-terminal acetylation shields proteins from degradation and promotes age-dependent motility and longevity
Nature Communications (2023)
-
Antagonistic control of myofiber size and muscle protein quality control by the ubiquitin ligase UBR4 during aging
Nature Communications (2021)
-
Epigenetic factors Polycomb (Pc) and Suppressor of zeste (Su(z)2) negatively regulate longevity in Drosophila melanogaster
Biogerontology (2018)
Comments
By submitting a comment you agree to abide by our Terms and Community Guidelines. If you find something abusive or that does not comply with our terms or guidelines please flag it as inappropriate.