Abstract
Two- or one-electron-mediated difunctionalizations of internal alkenes represent straightforward approaches to assemble molecular complexity by the simultaneous formation of two contiguous Csp3 stereocentres. Although racemic versions have been extensively explored, asymmetric variants, especially those involving open-shell C-centred radical species, are much more limited both in number and scope. Here we describe enantioenriched arylsulfinylamides as all-in-one reagents for the efficient asymmetric, intermolecular aminoarylation of alkenes. Under mild photoredox conditions, nitrogen addition of the arylsulfinylamide onto the double bond, followed by 1,4-translocation of the aromatic ring, produce, in a single operation, the corresponding aminoarylation adducts in enantiomerically enriched form. The sulfinyl group acts here as a traceless chiral auxiliary, as it is eliminated in situ under the mild reaction conditions. Optically pure β,β-diarylethylamines, aryl-α,β-ethylenediamines and α-aryl-β-aminoalcohols, prominent motifs in pharmaceuticals, bioactive natural products and ligands for transition metals, are thereby accessible with excellent levels of regio-, relative and absolute stereocontrol.

Similar content being viewed by others
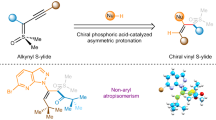
Main
Nature’s secondary metabolites, as well as de novo-designed small-molecule probes, are substantially populated with nitrogen atoms. HIV inhibitors1, ion-channel modulators2, opioids3,4 and endogenous neurotransmitters5 (Fig. 1a) are representative examples of relevant bioactive compounds showcasing N-containing motifs, many of which feature amines substituted with an aromatic group in the β-position. Access to these prominent chemical blueprints in enantiomerically pure form is crucial, not only for accurate target engagement studies, but also for the optimization of their pharmacological profiles. A representative example is R-(+)-dinapsoline, a selective and efficient D1 dopamine agonist5, which has been found to be 161-fold more potent than its S-(−)-enantiomer.
a, Examples of bioactive compounds featuring β-arylethylamines. TRPM8, transient receptor potential melastatin subtype 8. b, Previous examples of asymmetric three-component intermolecular alkene aminoarylations to attain β-arylethylamines38,39. Reactions are limited to the utilization of a single class of terminal olefins, either styrenes (R1 = Ar) or vinyl amides (R1 = NHCOR), and thus to the generation of a single stereogenic centre. N-fluoro-N-methylsulfonamides (NFAS) and O-acyl hydroxylmethylamine were used as N-atom donors, providing access exclusively to N-Me tertiary amine products. c, This work describes an asymmetric intermolecular alkene aminoarylation using arylsulfinylamides as multifunctional all-in-one reagents featuring a traceless chiral auxiliary. The reaction tolerates a wide variety of N-atom donors and is compatible with both 1,2-disubstituted styrenes, vinyl amides and vinyl ethers, thus providing access to valuable β,β-diarylethylamines, aryl-α,β-ethylenediamines and α-aryl-β-aminoalcohols. Excellent levels of both relative and absolute stereocontrol are achieved in the two newly forged stereogenic centres, governed by the configuration of the chiral sulfoxide tether. Characterization of the reaction mechanism revealed an interesting dichotomy in the initiation of the photoredox catalytic cycle wherein either electron-rich alkenes or sulfinylamides are preferentially activated at the expense of the Ir photocatalyst.
The asymmetric synthesis of β-arylethylamines has typically relied on additions6,7,8,9,10 or hydrogenations of alkenes11,12, as well as on ring-opening13,14,15 and condensation reactions16. These processes require multistep sequences encompassing highly tailored reaction conditions such as cryogenic temperatures and/or high pressures as well as precise metal/ligand combinations. This represents a significant limitation and hampers their broad applicability. In sharp contrast, two-17,18,19,20 or one-electron-mediated21,22,23,24,25,26,27 multicomponent aminoarylation reactions featuring alkenes as highly abundant feedstocks represent a powerful, atom-economic alternative to access these ubiquitous motifs as two contiguous Csp3–C and Csp3–N bonds can be forged in a single operation28,29,30,31,32,33,34. Despite the intrinsic potential to impart both relative and absolute stereocontrol in the newly formed stereocentres35, asymmetric variants of these transformations, especially in intermolecular settings, are extremely scarce. Benzohydroxamic acid derivatives18,36 and ortho-iodoanilines37 have been showcased as non-cleavable C,N-tethered reagents to orchestrate asymmetric annulations with alkenes. Additionally, a handful of examples featuring three-component reactions have been reported (Fig. 1b). In 2017, the addition of N-fluoro-N-alkylsulfonamides (NFSA)-derived radicals and (hetero)arylboronic acids across the π-system in the presence of a chiral BOX-ligated copper catalyst to yield β,β-diarylethylamines with excellent levels of absolute stereocontrol was demonstrated38. More recently, an asymmetric Minisci reaction involving quinoline derivatives and O-acyl hydroxylmethylamine with N-vinylacetamide as a radical acceptor was reported39. Notwithstanding the undisputable synthetic utility of these transformations, limitations regarding both the type of N donors and the olefinic partners justify the quest for alternative, more flexible strategies in this context.
An elegant light-mediated intermolecular aminoarylation of alkenes using arylsulfonylacetamides as bifunctional reagents has been demonstrated40,41,42,43. The reaction, only applicable to electron-rich styrenes, furnished the corresponding β,β-diarylethylamines in racemic form40. Recently, our group exploited the ability of chiral N-sulfinyl moieties to impart absolute stereocontrol in the challenging assembly of all-C quaternary centres44.
Inspired by these results, we hypothesized that the addition of a nitrogen atom bound to a chiral arylsulfoxide group onto the terminal position of a 1,2-disubstituted alkene could control the absolute stereochemistry in the formation of the newly created Csp3–N bond as well as on the neighbouring Csp3–Csp2 centre generated upon a radical Truce–Smiles rearrangement of the corresponding aryl moiety. In this Article we describe enantioenriched arylsulfinylamides as multifunctional all-in-one reagents able to forge, regio- and stereoselectively, two contiguous Csp3–C and Csp3–N bonds across a variety of π-systems. A photochemically enabled addition of the nitrogen atom onto the terminal position of styrenes, vinyl amides and vinyl ethers furnishes a C-radical intermediate, which, upon translocation of the aromatic ring, delivers enantioenriched β,β-diarylethylamines, aryl-α,β-ethylenediamines and α-aryl-β-aminoalcohols, respectively. The mild reaction conditions and broad functional-group tolerance, combined with excellent regio-, diastereo- and enantioselectivity, highlight both the generality and synthetic utility of these transformations in the assembly of relevant blueprints populating pharmaceuticals, bioactive natural products and ligands for transition-metal catalysis.
Results
Reaction optimization
Enantiopure (SS)-N-(p-tolylsulfinyl)butyramide 1a and trans-anethole were chosen as model substrates for our initial investigations. Reactions under blue light-emitting diode irradiation in the presence of different photocatalysts were performed, combining these two starting materials in a 1:1.2 ratio (experimental details are provided in Supplementary Table 1). Extensive screening revealed that, using 1 mol% of (Ir[dF(CF3)ppy]2(dtbpy))PF6 and 0.3 equiv. of potassium benzoate as the base in an isopropanol/trifluoroethanol/water mixture at ambient temperature, the desired β,β-diarylethylamine 2.1 could be produced in 53% yield with excellent diasteroselectivity (>20:1 d.r.) and promising 89:11 enantiomeric ratio (e.r.). Adjusting the stoichiometry between 1a and the olefin to a 1:2 ratio and decreasing the reaction temperature to −20 °C furnished 2.1 in an improved 83% yield with almost perfect levels of both relative and absolute stereocontrol (>20:1 d.r.; >99:1 e.r.). Furthermore, the efficiency of stereochemical information transfer was maintained when the reaction was scaled up tenfold, affording 2.1 in 58% yield (>20:1 d.r. and 98:2 e.r.; experimental details are provided in Supplementary Fig. 4). Additional experiments in the presence of radical inhibitors or excluding the photocatalyst, the light or the base resulted in the recovery of both unreacted starting materials (experimental details are provided in Supplementary Table 2).
Reaction scope
With the optimal conditions in hand, we set out to explore the compatibility of different N-atom donors and aryl migrating groups within the all-in-one arylsulfinylamide reagents. To this end, modifications on both the N-atom donor and the aryl migrating group were investigated. Alkyl amide derivatives featuring a diverse set of substitution patterns were also compatible with the standard reaction conditions, furnishing the corresponding adducts (CH2PMP, 2.2; CHEtPh, 2.3; Cy, 2.4; (CH2)2CO2Et, 2.5; CH2CH(OTBS)Me, 2.6) with excellent levels of regio- and both relative and absolute stereocontrol (Table 1). Furthermore, successful incorporation of aromatic and heteroaromatic substituted amides and even the tert-butyl carbamate derivative (2.7–2.9) emphasizes the functional-group compatibility of the method. Furthermore, carbamate derivative (2.9) could also be transformed under the standard conditions, providing access to the corresponding free amine upon acid hydrolysis, with complete retention of the stereochemical information (Supplementary Information, compound 2.39).
The scope with respect to the migrating aromatic groups was investigated next. Transposition of a simple phenyl group proceeded smoothly under standard conditions to give 2.10 in high yield. Interestingly, substrates bearing both electron-withdrawing and electron-donating groups in the para-position of the arene proved to be suitable precursors, furnishing the corresponding β,β-diarylethylamines (2.11–2.14) in good yields with outstanding levels of stereocontrol. The meta-methoxy and meta-bromo derivatives also delivered the desired products (m-OMe, 2.15; m-Br, 2.16), although with slightly lower stereoinduction. In contrast, more sterically hindered substrates bearing ortho-substituted aromatic rings (o-Me, 2.17; o-Br, 2.18) were obtained with excellent enantioselectivities. To our delight, the ortho-bromo adduct 2.18 was quantitatively converted into the corresponding indoline in the presence of the Pd catalyst with retention of configuration, highlighting the synthetic potential of the obtained aminoarylation products (Supplementary Information, compound 2.40). Moreover, heteroaryl migration also took place under the standard conditions, furnishing the corresponding thiophene derivatives 2.19 and 2.20 in good yields, with excellent levels of both relative and absolute stereocontrol.
X-ray crystallographic analysis of compounds 2.3 and 2.11 confirmed the syn addition of the N atom and the arene across the π-system. Adduct 2.11, stemming from an (SS)-arylsulfinylamide precursor containing a Br atom, enabled us to assign the absolute configuration of the major diastereoisomer produced in this reaction as (1S,2R). It is important to note that the substitution pattern in the aromatic ring affects the priority of the groups at the new asymmetric carbon atom. As a result, a (1R,2R) configuration can be assigned to most of the obtained compounds. The reaction proved to be stereospecific: when the (R)-enantiomer of the arylsulfinylamide (RS)-1a′ was used as a precursor, the opposite enantiomer of the β,β-diarylethylamine product (1S,2S)-2.1′ could be obtained in similar yield and e.r. (experimental details are provided in Supplementary Fig. 28).
The compatibility of the reaction between (SS)-N-(p-tolylsulfinyl)butyramide 1a with different styrene partners was also explored (Table 2). Although simple styrenes (R3 = H) were not competent substrates, phenethyl, cyclohexyl, 4-tetrahydropyranyl and carbinyl acetate groups at the terminal position of the double bond were effectively accommodated in the aminoarylation process. The corresponding β,β-diarylethylamines (2.21–2.25) were obtained in moderate to good yields with high enantioselectivity. Moreover, a chiral para-methoxy styrene derived from (R)-citronellal provided the corresponding aminoarylation adduct 2.26 in moderate yield but with excellent levels of regio- and both relative and absolute stereocontrol.
To further expand the scope of this multicomponent radical cascade, different electron-rich olefins were surveyed. To our delight, aromatic vinyl amides turned out to be suitable partners, providing efficient access to aryl-α,β-ethylenediamines. These motifs are not only present in biologically active compounds2,45, but have also been prominently used as bidentate ligands in transition-metal complexes46,47. Thus, α,β-diamine derivatives (p-OMe, 2.27; p-Me, 2.28; o-OMe, 2.29; o-Me, 2.30) were accessed in good yields and with moderate to excellent enantioselectivities. Furthermore, α- and β-methyl-substituted vinyl benzamides were efficiently transformed into the corresponding adducts (β-Me, 2.31–2.33; α-Me, 2.34) featuring an even more sterically demanding quaternary stereocentre with perfect stereochemical information transfer. Finally, vinyl ethers could be successfully engaged in the reaction, providing access to protected α-aryl-β-aminoalcohols (2.35–2.38) in good yields with moderate to excellent enantioselectivities.
Mechanistic investigations
Having demonstrated the synthetic utility of this methodology, we focused our investigations on the underlying reaction mechanism. First, Stern–Volmer fluorescence quenching studies were performed to shed light on the potential species activated by the photocatalyst at the outset of the reaction48. The experiments were conducted using [Ir[(dFCF3)ppy]2(dtbpy)]PF6 excited with light (430 nm) in the presence of the different reactants. In the case of trans-anethole, a decrease in fluorescence intensity was observed as a function of olefin concentration (Fig. 2, top left). In sharp contrast, (E)-N-(prop-1-en-1-yl)benzamide did not quench the excited photocatalyst, even at high concentrations (Supplementary Figs. 7–12). Cyclic voltammetry of this vinylamide (E1/2 = +1.45 V versus saturated calomel electrode (SCE) in MeCN) confirmed the mismatched redox potential with respect to that of the photocatalyst (E1/2 = +1.26 V versus SCE in MeCN) (Supplementary Fig. 25)40. Interestingly, fluorescence quenching was not observed at low concentrations of arylsulfinylamide 1a and potassium benzoate. Increasing the concentration of either arylsulfinylamide 1a or both 1a and base (Supplementary Figs. 13–18) led to oxidation of the reagent49. A more soluble tetrabutylammonium-conjugated sulfinylamide salt 3 proved to be an efficient quencher of the iridium photocatalyst (Fig. 2, top right), in line with the reduction potential measured by cyclic voltammetry (E1/2 = +0.57 V versus SCE in MeCN) (Supplementary Fig. 26).
Results of Stern−Volmer experiments using trans-anethole (top left) and arylsulfinylamide 3 (top right) as quenchers (I, intensity, e.u., arbitrary energy units). The proposed reaction mechanism is shown, featuring two different initiation pathways: formation of a radical cation for electron-rich olefins (grey) and formation of an amidyl radical in the case of vinylamide acceptors (pink). DFT calculations were performed on trans-anethole as a benchmark substrate. Optimized transition states, relevant structural parameters, starting materials, products, reaction intermediates and transition states were computed at the M062X/6-31+G(d,p) level (IEFPCM, integral equation formalism with the polarizable continuum model), with the solvent 2-propanol at −20 °C (R = PMP). Energies are given in units of kcal mol−1. For further details, see Supplementary section ‘DFT calculations’. TSI–III (SS,R) visualizes the enantiodetermining step in which I adds to the alkene radical cation II in line with the absolute configuration observed in the aminoarylation products. The alternative TSI–III (SS,S), is 1.3 kcal mol−1 higher in energy than TSI–III (SS,R). Conformations were calculated for intermediates III and III′. The conformer yielding the minor isomer is disfavoured by steric factors: the PMP group adopts an unfavourable syn-periplanar disposition with the methyl group, unlike the case of the major diastereomer experimentally obtained, in which these groups exhibit a less sterically demanding anti-periplanar geometry. PMP, p-methoxyphenyl, p-Tol, p-methylphenyl.
These results indicate that different mechanisms might be operating at the outset of the reaction, depending on the olefinic partner. In the case of electron-rich styrenes, the formation of a radical cation via single-electron oxidation can be confidently proposed as the initial step of the photocatalytic cycle. In contrast, single-electron oxidation of the deprotonated arylsulfinylamide by the excited Ir photocatalyst to form an N-centred radical seems to be a more likely first step in the case of poorly oxidizable olefins.
To gain additional insights into the stereochemical outcome of these transformations, several control experiments as well as density functional theory (DFT) calculations were performed using anethole derivatives as benchmark substrates. First, the standard reaction conditions were applied in three independent experiments featuring cis-, trans- and a 1:1 mixture of cis- and trans-anethole. The formation of the corresponding products was analysed by 1H NMR (experimental details are provided in Supplementary Fig. 29). In all three cases, the aminoarylation adduct 2.1 was obtained in comparable yields, with almost identical d.r. and e.r. values. Next, and this time in the absence of arylsulfinylamide 1a, cis- and trans-anethole were separately subjected to the standard reaction conditions and their potential isomerization50 was monitored by 1H NMR. A plot of temporal concentration versus time revealed that, after only 10 min, both isomers converge to an ~1.7:1 cis-to-trans ratio (Supplementary Figs. 5 and 6). Such a photostationary state, reached in a much faster regime than the aminoarylation reaction itself, suggests that both isomers will be present at the outset of the reaction, regardless of the initial alkene geometry. Following olefin oxidation to the corresponding radical cation II, addition of the arylsulfinylamide I will proceed at the β-carbon atom so that the absolute configuration of the first stereogenic centre is thus defined by that of the chiral sulfinyl moiety. DFT calculations revealed a low-energy transition state TSI–III (SS,R) (ΔGǂ = +4.8 kcal mol−1) for this step, which delivers the benzylic radical III in a net exothermic process (ΔG = −25.2 kcal mol−1). Intermediate III undergoes a 1,4-aryl shift. No radical Meisenheimer intermediate could be located along the reaction energy profile51. Instead, a spirocyclic transition state TSIII–IV was found to precede the exothermic formation of SO-centred radical IV (ΔG = −38.5 kcal mol−1). TSIII–IV can be considered an early transition state in which the new C–C bond between the benzylic radical and the migrating aromatic group is only marginally formed (dCbn−Csp2 = 2.11 Å in TSIII–IV versus dCbn−Csp2 = 1.52 Å in IV), and the S(O)–C bond is barely elongated (dS–Csp2 = 1.82 Å in TSIII–IV versus dS–Csp2 = 1.80 Å in III). Formation of the minor diastereoisomer can be traced back to the generation of intermediate III′ before the aryl transposition. Conformational analysis of the two intermediates suggests that the aryl translocation preferentially takes place through a trajectory in which the steric interactions between the PMP group and the methyl substituent within the anethole are minimized (ΔΔGIII/III′ = +5.8 kcal mol−1). DFT calculations support the notion of the aryl migration being the rate-determining step (TSIII–IV, ΔGǂ = +12.2 kcal mol−1). As a result, and regardless of any potential kinetic preference for the formation and/or subsequent reactivity of either a Z- or an E-anethole-derived radical cation, the fast interconversion of III′ into III by rotation along the Cα–Cβ bond supports the syn relative configuration observed in the aminoarylation products (additional details are provided in Supplementary Fig. 30). The photocatalytic cycle is closed thereafter by oxidation of IV to V by Ir(II) to recover the Ir(III) catalyst. The precise fate of the sulfur-based chiral linker is challenging to assess. However, having detected the bisulfite (HSO3−) anion using commercially available colorimetric test strips, we can confirm that sulfur(IV) species account at least in part for the SO lost52 (additional details are provided in Supplementary Fig. 27). Additionally, Fig. 2 shows TSI–III (SS,S), the alternative transition state for the enantiodetermining step, in which I adds to the alkene radical cation II. TSI–III (SS,S) is 1.3 kcal mol−1 higher in energy than TSI–III (SS,R), which rationalizes the absolute configuration observed in the aminoarylation products.
Conclusion
Here we have described an asymmetric intermolecular aminoarylation of alkenes. A photoredox-mediated radical cascade capitalizes on a chiral all-in-one arylsulfinylamide reagent featuring a traceless chiral auxiliary to forge two vicinal Csp3–Csp2 and Csp3–N bonds across the π-system in a stereocontrolled manner. Mechanistic investigations revealed the likelihood of multiple reaction pathways operating in these transformations. In the case of electron-rich styrenes, the formation of a radical cation via single-electron oxidation can be confidently proposed at the outset of the reaction. In contrast, the single-electron oxidation of the deprotonated arylsulfinylamide by the excited Ir photocatalyst to form an N-centred radical seems favoured in the case of poorly oxidizable olefins. The C–N bond formation is stereocontrolled by the chirality of the sulfoxide, whereas the subsequent transposition of the aromatic ring with concomitant elimination of the sulfinyl tether proceeds in a highly diastereoselective manner governed by steric factors. The β,β-diarylethylamines, aryl-α,β-ethylenediamines and α-aryl-β-aminoalcohols, ubiquitous motifs in bioactive molecules as well as in bidentate transition-metal ligands, are obtained herein with very high levels of regio- and both relative and absolute stereocontrol, thus highlighting the synthetic utility of this methodology.
Methods
To an oven-dried Schlenk tube (5 ml), the corresponding arylsulfinylamide (0.1 mmol, 1 equiv.), PhCO2K (4.8 mg, 0.03 mmol, 0.3 equiv.) and [Ir[(dFCF3)ppy]2(dtbpy)]PF6 (1.1 mg, 0.001 mmol, 1 mol%) were sequentially added under a flow of nitrogen. The flask was evacuated and then backfilled with N2 (three times). Trifluoroethanol (72 μl) and i-PrOH:H2O (0.5 ml, 9:1 (vol:vol)) were then added to the reaction mixture, followed by the olefin (0.2 mmol, 2.0 equiv.). The reaction was sparged with argon for 15 min. The Schlenk tube was placed in the photoreactor and stirred at 1,400 r.p.m. under blue-light irradiation (EvoluChem 30W, HCK1012-01-008) at −20 °C. After four days, the reaction mixture was diluted with ethyl acetate (10 ml) and transferred into a separatory funnel. The mixture was washed with a 5 wt% aqueous LiCl solution (3 × 10 ml). The organic phases were dried over MgSO4, filtered, and concentrated in vacuo. The residue was purified by flash chromatography using a mixture of ethyl acetate and n-hexane.
Data availability
All data collected as part of this study are available within the Article and its Supplementary Information. Crystallographic data for the structures reported in this Article have been deposited at the Cambridge Crystallographic Data Centre, under deposition nos. CCDC 2270374 (1s′), 2212927 (2.3) and 2212932 (2.11). Copies of the data can be obtained free of charge via https://www.ccdc.cam.ac.uk/structures/.
References
McCauley, J. A. et al. HIV protease inhibitors. PCT patent WO2014043019 A1 (2014).
González-Muñiz, R., Bonache, M. A., Martín-Escura, C. & Gómez-Monterrey, I. Recent progress in TRPM8 modulation: an update. Int. J. Mol. Sci. 20, 2618 (2019).
Sverrisdóttir, E. et al. A review of morphine and morphine-6-glucuronide’s pharmacokinetic-pharmacodynamic relationships in experimental and clinical pain. Eur. J. Pharm. Sci. 74, 45–62 (2015).
Hanessian, S., Parthasarathy, S. & Mauduit, M. The power of visual imagery in drug design. Isopavines as a new class of morphinomimetics and their human opioid receptor binding activity. J. Med. Chem. 46, 34–48 (2003).
Zhang, A., Neumeyer, J. L. & Baldessarini, R. J. Recent progress in development of dopamine receptor subtype-selective agents: potential therapeutics for neurological and psychiatric disorders. Chem. Rev. 107, 274–302 (2007).
Wang, Z.-Q., Feng, C.-G., Zhang, S.-S., Xu, M.-H. & Lin, G.-Q. Rhodium-catalyzed asymmetric conjugate addition of organoboronic acids to nitroalkenes using chiral bicyclo[3.3.0] diene ligands. Angew. Chem. Int. Ed. 49, 5780–5783 (2010).
He, F.-S. et al. Direct asymmetric synthesis of β-bis-aryl-α-amino acid esters via enantioselective copper-catalyzed addition of p-quinone methides. ACS Catal. 6, 652–656 (2016).
Li, W. et al. Enantioselective organocatalytic 1,6-addition of azlactones to para-quinone methides: an access to α,α-disubstituted and β,β-diaryl-α-amino acid esters. Org. Lett. 20, 1142–1145 (2018).
Nishino, S., Miura, M. & Hirano, K. An umpolung-enabled copper-catalysed regioselective hydroamination approach to α-amino acids. Chem. Sci. 12, 11525–11537 (2021).
Röben, C., Souto, J. A., González, Y., Lishchynskyi, A. & Muñiz, K. Enantioselective metal-free diamination of styrenes. Angew. Chem. Int. Ed. 50, 9478–9482 (2011).
Molinaro, C. et al. Catalytic, asymmetric and stereodivergent synthesis of non-symmetric β,β-diaryl-α-amino acids. J. Am. Chem. Soc. 137, 999–1006 (2015).
Gao, W. et al. Nickel-catalyzed asymmetric hydrogenation of β-acylamino nitroolefins: an efficient approach to chiral amines. Chem. Sci. 8, 6419–6422 (2017).
Valdez, S. C. & Leighton, J. L. Tandem asymmetric aza-Darzens/ring-opening reactions: dual functionality from the silane Lewis acid. J. Am. Chem. Soc. 131, 14638–14639 (2009).
Takeda, Y., Ikeda, Y., Kuroda, A., Tanaka, S. & Minakata, S. Pd/NHC-catalyzed enantiospecific and regioselective Suzuki-Miyaura arylation of 2-arylaziridines: synthesis of enantioenriched 2-arylphenethylamine derivatives. J. Am. Chem. Soc. 136, 8544–8547 (2014).
Chai, Z. et al. Synthesis of chiral vicinal diamines by silver(I)-catalyzed enantioselective aminolysis of N-tosylaziridines. Angew. Chem. Int. Ed. 56, 650–654 (2017).
Gómez-Arrayás, R. & Carretero, J. C. Catalytic asymmetric direct Mannich reaction: a powerful tool for the synthesis of α,β-diamino acids. Chem. Soc. Rev. 38, 1940–1948 (2009).
White, D. R., Hutt, J. T. & Wolfe, J. P. Asymmetric Pd-catalyzed alkene carboamination reactions for the synthesis of 2-aminoindane derivatives. J. Am. Chem. Soc. 137, 11246–11249 (2015).
Ozols, K., Onodera, S., Woz´niak, L. & Cramer, N. Cobalt(III)-catalyzed enantioselective intermolecular carboamination by C-H functionalization. Angew. Chem. Int. Ed. 60, 655–659 (2021).
Schultz, D. M. & Wolfe, J. P. Recent developments in Pd-catalyzed alkene aminoarylation reactions for the synthesis of nitrogen heterocycles. Synthesis 44, 351–361 (2012).
Zhang, W., Chen, P. & Liu, G. Enantioselective palladium(II)-catalyzed intramolecular aminoarylation of alkenes by dual N-H and aryl C-H bond cleavage. Angew. Chem. Int. Ed. 56, 5336–5340 (2017).
Jiang, H. & Studer, A. Intermolecular radical carboamination of alkenes. Chem. Soc. Rev. 49, 1790–1811 (2020).
Moon, Y. et al. Visible light induced alkene aminopyridylation using N-aminopyridinium salts as bifunctional reagents. Nat. Commun. 10, 4117 (2019).
Jiang, H., Yu, X., Daniliuc, C. G. & Studer, A. Three-component aminoarylation of electron-rich alkenes by merging photoredox with nickel catalysis. Angew. Chem. Int. Ed. 60, 14399–14404 (2021).
Muñoz-Molina, J. M., Belderrain, T. R. & Pérez, P. J. Copper-catalysed radical reactions of alkenes, alkynes and cyclopropanes with N-F reagents. Org. Biomol. Chem. 18, 8757–8770 (2020).
Liu, Z. & Liu, Z.-Q. An intermolecular azidoheteroarylation of simple alkenes via free radical multicomponent cascade reactions. Org. Lett. 19, 5649–5652 (2017).
Hari, D. P., Hering, T. & König, B. The photoredox-catalyzed Meerwein addition reaction: intermolecular amino-arylation of alkenes. Angew. Chem. Int. Ed. 53, 725–728 (2014).
Fumagalli, G., Boyd, S. & Greaney, M. F. Oxyarylation and aminoarylation of styrenes using photoredox catalysis. Org. Lett. 15, 4398–4401 (2013).
Brenzovich, W. E. et al. Gold-catalyzed intramolecular aminoarylation of alkenes: C-C bond formation through bimolecular reductive elimination. Angew. Chem. Int. Ed. 49, 5519–5522 (2010).
Sahoo, B., Hopkinson, M. N. & Glorius, F. Combining gold and photoredox catalysis: visible light-mediated oxy- and aminoarylation of alkenes. J. Am. Chem. Soc. 135, 5505–5508 (2013).
Tathe, A. G. et al. Gold-catalyzed 1,2-aminoarylation of alkenes with external amines. ACS Catal. 11, 4576–4582 (2021).
Lee, S. & Rovis, T. Rh(III)-catalyzed three-component syn-carboamination of alkenes using arylboronic acids and dioxazolones. ACS Catal. 11, 8585–8590 (2021).
Liu, Z. et al. Catalytic intermolecular carboamination of unactivated alkenes via directed aminopalladation. J. Am. Chem. Soc. 139, 11261–11270 (2017).
Hajra, S., Maji, B. & Mal, D. A catalytic and enantioselective synthesis of trans-2-amino-1-aryltetralins. Adv. Synth. Catal. 351, 859–864 (2009).
Akhtar, S. M. S., Bar, S. & Hajra, S. Asymmetric aminoarylation for the synthesis of trans-3-amino-4-aryltetrahydroquinolines: an access to aza-analogue of dihydrexidine. Tetrahedron 103, 132257 (2021).
Kwon, Y. & Wang, Q. Recent advances in 1,2-amino(hetero)arylation of alkenes. Chem. Asian J. 17, e202200215 (2022).
Ye, B. & Cramer, N. Chiral cyclopentadienyl ligands as stereocontrolling element in asymmetric C-H functionalization. Science 338, 504–506 (2012).
Bizet, V., Borrajo-Calleja, G., Besnard, C. & Mazet, C. Direct access to furoindolines by palladium-catalyzed intermolecular carboamination. ACS Catal. 6, 7183–7187 (2016).
Wang, D. et al. Asymmetric copper-catalyzed intermolecular aminoarylation of styrenes: efficient access to optical 2,2-diarylethylamines. J. Am. Chem. Soc. 139, 6811–6814 (2017).
Zheng, D. & Studer, A. Asymmetric synthesis of heterocyclic γ-amino-acid and diamine derivatives by three-component radical cascade reactions. Angew. Chem. Int. Ed. 58, 15803–15807 (2019).
Monos, T. M., McAtee, R. C. & Stephenson, C. R. J. Arylsulfonylacetamides as bifunctional reagents for alkene aminoarylation. Science 361, 1369–1373 (2018).
Allen, A. R. et al. Mechanism of visible light-mediated alkene aminoarylation with arylsulfonylacetamides. ACS Catal. 12, 8511–8526 (2022).
Noten, E., Ng, C., Wolesensky, R. & Stephenson, C. R. J. A general alkene aminoarylation enabled by N-centered radical reactivity of sulfinamides. Preprint at https://chemrxiv.org/engage/chemrxiv/article-details/6347680bbb6d8b9cf1568f62 (2022).
Allen, A. R., Noten, E. A. & Stephenson, C. R. J. Aryl transfer strategies mediated by photoinduced electron transfer. Chem. Rev. 122, 2695–2751 (2022).
Hervieu, C. et al. Asymmetric, visible light-mediated radical sulfinyl-Smiles rearrangement to access all-carbon quaternary stereocentres. Nat. Chem. 13, 327–334 (2021).
Desai, M. C., Lefkowitz, S. L., Bryce, D. K. & McLean, S. Articulating a pharmacophore driven synthetic strategy: discovery of a potent substance P antagonist. Bioorg. Med. Chem. Lett. 4, 1865–1868 (1994).
Saito, B. & Fu, G. C. Enantioselective alkyl-alkyl Suzuki cross-couplings of unactivated homobenzylic halides. J. Am. Chem. Soc. 130, 6694–6695 (2008).
Zhang, B., Wang, H., Lin, G.-Q. & Xu, M.-H. Ruthenium(II)-catalyzed asymmetric transfer hydrogenation using unsymmetrical vicinal diamine-based ligands: dramatic substituent effect on catalyst efficiency. Eur. J. Org. Chem. 2011, 4205–4211 (2011).
Gehlen, M. H. The centenary of the Stern-Volmer equation of fluorescence quenching: from the single line plot to the SV quenching map. J. Photochem. Photobiol. C Photochem. Rev. 42, 100338 (2019).
Cismesia, M. A. & Yoon, T. P. Characterizing chain processes in visible light photoredox catalysis. Chem. Sci. 6, 5426–5434 (2015).
Neveselý, T., Wienhold, M., Molloy, J. J. & Gilmour, R. Advances in the E → Z isomerization of alkenes using small molecule photocatalysts. Chem. Rev. 122, 2650–2694 (2022).
Okada, K. & Sekiguchi, S. Aromatic nucleophilic substitution. 9. Kinetics of the formation and decomposition of anionic σ complexes in the Smiles rearrangements of N-acetyl-β-aminoethyl 2-X-4-nitro-1-phenyl or N-acetyl-β-aminoethyl 5-nitro-2-pyridyl ethers in aqueous dimethyl sulfoxide. J. Org. Chem. 43, 441–447 (1978).
Herron, J. T. & Huie, R. E. Rate constants at 298 K for the reactions SO + SO + M → (SO)2 + M AND SO + (SO)2 → SO2 + S2O. Chem. Phys. Lett. 76, 322–324 (1980).
Acknowledgements
We thank A. Linden and O. Blacque for the X-ray diffraction analysis of 1s′ (CCDC no. 2270374), 2.3 (CCDC no. 2212927) and 2.11 (CCDC no. 2212932). This work was supported by the Swiss National Science Foundation (SNF es 200021_ 184986). This publication was created as part of NCCR Catalysis, a National Centre of Competence in Research funded by the Swiss National Science Foundation. We also thank the Comunidad de Madrid Research Talent Attraction Program (2018-T1/IND-10054 and 2022-5A/IND-24227 to E.M.). Correspondence and requests for materials should be addressed to C.N.
Author information
Authors and Affiliations
Contributions
C.H., M.S.K., Y.H. and S.C.-G. performed the experiments. E.M. performed DFT calculations. C.H., M.S.K., E.M. and C.N. analysed the data and co-wrote the manuscript. E.M. and C.N. conceptualized and supervised the project.
Corresponding authors
Ethics declarations
Competing interests
The authors declare no competing interests.
Peer review
Peer review information
Nature Chemistry thanks Robert Stockman and the other, anonymous, reviewer(s) for their contribution to the peer review of this work.
Additional information
Publisher’s note Springer Nature remains neutral with regard to jurisdictional claims in published maps and institutional affiliations.
Supplementary information
Supplementary Information
General information, reaction optimization data, experimental procedures, additional experiments, compound characterization including spectroscopic and analytical data for all new compounds, X-ray crystallographic data, NMR and HPLC spectra, computational details, Supplementary Figs. 1–30, Tables 1–3 and references.
Supplementary Data 1
Crystallographic data for compounds 1s′; CCDC reference, 2270374.
Supplementary Data 2
Crystallographic data for compounds 2.3; CCDC reference, 2212927.
Supplementary Data 3
Crystallographic data for compounds 2.11; CCDC reference, 2212932.
Supplementary Data 4
Coordinates of DFT calculations.
Rights and permissions
Open Access This article is licensed under a Creative Commons Attribution 4.0 International License, which permits use, sharing, adaptation, distribution and reproduction in any medium or format, as long as you give appropriate credit to the original author(s) and the source, provide a link to the Creative Commons license, and indicate if changes were made. The images or other third party material in this article are included in the article’s Creative Commons license, unless indicated otherwise in a credit line to the material. If material is not included in the article’s Creative Commons license and your intended use is not permitted by statutory regulation or exceeds the permitted use, you will need to obtain permission directly from the copyright holder. To view a copy of this license, visit http://creativecommons.org/licenses/by/4.0/.
About this article
Cite this article
Hervieu, C., Kirillova, M.S., Hu, Y. et al. Chiral arylsulfinylamides as reagents for visible light-mediated asymmetric alkene aminoarylations. Nat. Chem. 16, 607–614 (2024). https://doi.org/10.1038/s41557-023-01414-8
Received:
Accepted:
Published:
Issue Date:
DOI: https://doi.org/10.1038/s41557-023-01414-8
This article is cited by
-
Photoredox catalytic alkylarylation of alkynes with arylsulfonylacetate as bifunctional reagent
Science China Chemistry (2024)