Abstract
Sulfonyl fluorides have attracted considerable and growing research interests from various disciplines, which raises a high demand for novel and effective methods to access this class of compounds. Radical flurosulfonylation is recently emerging as a promising approach for the synthesis of sulfonyl fluorides. However, the scope of applicable substrate and reaction types are severely restricted by limited known radical reagents. Here, we introduce a solid state, redox-active type of fluorosulfonyl radical reagents, 1-fluorosulfonyl 2-aryl benzoimidazolium triflate (FABI) salts, which enable the radical fluorosulfonylation of olefins under photoredox conditions. In comparison with the known radical precursor, gaseous FSO2Cl, FABI salts are bench-stable, easy to handle, affording high yields in the radical fluorosulfonylation of olefins with before challenging substrates. The advantage of FABIs is further demonstrated in the development of an alkoxyl-fluorosulfonyl difunctionalization reaction of olefins, which forges a facile access to useful β-alkoxyl sulfonyl fluorides and related compounds, and would thus benefit the related study in the context of chemical biology and drug discovery in the future.
Similar content being viewed by others
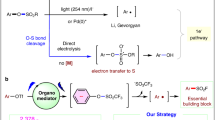
Introduction
Since Sharpless and co-workers introduced sulfur (VI) fluoride exchange (SuFEx) reactions as a new generation of click chemistry1, the popularity of sulfonyl fluorides has grown dramatically over the recent past1,2,3,4, with applications in a wide range of fields, including organic synthesis5,6,7,8,9,10, polymer preparation11,12,13,14, materials science15,16,17, chemical biology etc18,19,20. Particularly, unique and appealing properties were often observed, which has attracted a fast-growing research interest on sulfonyl fluoride being a privileged warhead in chemical biology and drug discovery1,4,18, and successful examples keep emerging in the past years18,19,20,21,22,23,24,25,26,27. Remarkably, enhanced activity was often observed when the SO2F moiety was introduced, resembling, to some extent, the common beneficial effect of trifluoromethyl and fluorine groups in pharmaceuticals18,19,20,28,29,30,31,32,33. For example, Sharpless, and co-workers recently found that fluorosulfonylated Resveratrol showed a potent agent against resistant bacteria, higher than that of the parent compound by over 200-fold (Fig. 1)33. Accordingly, novel and efficient synthetic protocols to broaden the scope of available sulfonyl fluorides are desirable1,2,3,4,19,20,21.
Among the most common methods for the synthesis of sulfonyl fluorides1,2,3,4,34,35,36,37,38,39,40,41,42,43,44,45,46, direct fluorosulfonylation45,46,47,48 undoubtedly represents a concise and effective approach, and could be particularly useful in the late-stage modifications of drugs and biomolecules1,2,3,4. Most of the fluorosulfonylating reagents reported so far belong to the FSO2 + -type of synthons, including the well-known sulfuryl fluoride gas (SO2F2)1 and other solid reagents (such as FDIT, recently reported by Sharpless, Dong et al., which exhibited high reactivity in the fluorosulfonylation of phneols and amines47,48. In contrast, fluorosulfonylation with the corresponding fluorosulfonyl radical (FSO2•) remains less investigated4, likely due to the instability and challenging preparation49. Recently, we used sulfuryl chlorofluoride (FSO2Cl) as a radical precursor and we reported the radical fluorosulfonylation of alkenes50,51, affording an effective method for the preparation of important alkenyl sulfonyl fluorides50,51,52,53,54,55,56. However, when we applied this reagent to the development of other transformations, e.g., the alkoxy-fluorosulfonylation reaction of styrene (Fig. 2a), we failed to obtained any desired product even after extensive optimization. Instead, undesired chloro- and styryl-sulfonyl fluorides were obtained, which were supposed to the products from a radical chain mechanism (Path I, Fig. 2b)50. The weak S-Cl bond in FSO2Cl with a highly reactive chloride renders a fast chloride atom transfer (k estimated >106 M−1s−1)50,57 from FSO2Cl to the radical intermediate Int-A; this rapid radical chain propagation (Path I) makes it difficult to trap this radical with other reagents or by single electron transfer (SET) oxidation to establish a photoredox reaction pathway (Path II). Given this challenging issue and also other limitations with FSO2Cl, such as: chlorination side-reactions and low/no yields with electron-rich substrates (as the chloride in FSO2Cl is highly electrophilic due to the electron-withdrawing effect of the FSO2-group)50, inconvenience in storage and handling due to the gaseous (b.p. 7 °C) and moisture-sensitive nature, the development of a new and convenient FSO2 radical precursor (X ≠ Cl) is highly desirable. Here, we report our efforts toward this goal, and the introduction of a solid-state, bench-stable type of reagents, 1-fluorosulfonyl 2-aryl benzoimidazolium triflate (FABI) salts, which can serve as effective redox-active FSO2 radical precursors and enable the development of radical fluorosulfonylation of olefins via a photoredox catalytic pathway. FABI is compatible with many substrates that were not compatible or low yielding when FSO2Cl is used, such as electron-rich alkenes and triaryl ethylenes. Moreover, a cascade alkoxy-fluorosulfonyl difunctionalization of olefins with FABI is presented, by trapping the postulated cationic intermediate Int-B with alcohols via a photoredox pathway (Fig. 2b, c).
a The development of radical alkoxy-fluorosulfonylation of styrene with alcohol as a nuleophile. b Radical fluorosulfonylation of alkenes via radical chain pathway (Path I) versus photoredox pathway (Path II). c This work: photoredox catalytic radical fluorosulfonylation of olefins enabled by redox-active radical reagent development.
Results
Reaction optimization
We commenced our study with the screening of suitable FSO2 radical precursors in the form of imidazolium salts under photoredox conditions (Table 1). In the beginning, we tried a sample imidazolium salt, 2a47,58, but it was found that 2a was unable to generate the FSO2 radicals under this photoredox condition, delivering no any detectable formation of the desired product (entry 1). This is unexpected, as the excited fac-Ir(ppy)3 should be reducing enough (−1.73 V vs SCE) to reduce 2a (−1.03 V vs SCE) via single electron transfer (SET). We guess the extrusion of FSO2 radicals after accepting one electron from excited fac-Ir(ppy)3 requires a good driving force of re-aromatization (for details, see the mechanistic discussion later). Therefore, we tested the imidazolium salt 2b and 2c58, with a 2-substitued or a fused phenyl group, respectively. Encouragingly, we could observe a trace amount of 3aa (entry 2 and 3) Then, we combined the effects, and synthesized two 1-fluorosulfonyl 2-aryl benzoimidazolium triflate (FABI) salts: 2d and 2e. To our delight, 2d afforded a substantial improvement in the reaction efficiency (entry 4), and the yield of the desired product 3aa can be further improved to above 90% by using 2e as the precursor, together with a high E/Z ratio (94%, entry 5). In this case, the desired product can be isolated in 90% yield. For more details about the reaction optimizations, please see the Supplementary Table 1 and 2. In addition, control experiments indicated that both the photocatalyst and light are crucial to the reaction (entry 7 and 8).
Substrate scope
Having the optimized reaction conditions in hand, we moved on to investigate the reaction scope. As shown in Fig. 3, this protocol could readily accommodate a variety of styrenes (3aa-3ap) and showed a good tolerance of various functional groups, including halides (F, Cl, Br, 3ae-3ai), ester (3an), and nitrile (3ao), etc. Notably, 4-methoxystyrene is compatible with the current conditions with FABI 2e, the desired sulfonyl fluoride 3ad can be obtained in 66% yield. In sharp contrast, the previous method with FSO2Cl as the FSO2 radical precursor afforded a messy reaction, and no desired product was obtained50. Aliphatic alkenes were less favored by this system (3aq-3ar), in line with the photoredox mechanism and the higher difficulty in oxidizing simple alkyl radicals than benzylic radicals59. Nevertheless, to our great pleasure, this FSO2 radical reagent (2e) is well compatible with electron-rich olefins, allowing for a facile access to β-O- or N-substituted vinyl sulfonyl fluorides (3as-3bb). As shown in Fig. 3, alkyl vinyl ethers (3as-3av), phenyl vinyl ether (3aw), vinyl acetates (3ax), vinyl thioether (3ay), and N-vinyl amides (3az-3bb), were all well tolerated, which further demonstrated the usefulness and advantages of FABI reagents over FSO2Cl.
The reactions were performed on 0.1 or 0.2 mmol scales, with 2 equiv. of 2e in 1,4-dioxane at room temperature for 12 h. aYields on 1.0 mmol scale. bThe yields in parentheses are the reactions with FSO2Cl50. cWith 3.0 eq. of 2e. dWith 1.0 eq. of K3PO4. eWith 1.0 eq. of K2CO3. *Yields on 1.0 mmol scale.
The direct radical fluorosulfonylation of cyclic, di- and tri-substituted olefins enables the preparation of multi-substituted vinyl sulfonyl fluorides. As shown in Fig. 4, the current protocol with FABI 2e as the FSO2 radical reagent was found very effective for the diaryl and triaryl olefins (4ad, 4ae, and 4ag-4an), delivering the corresponding products in much higher yield than that of reactions with FSO2Cl. For example, indene and 1,2-dihydronaphthalene readily underwent the functionalization to give 5ab and 5ac in 91% and 86% yield with FABI 2e, respectively, while the yields were 68% and 63% when using FSO2Cl as the radical precursor50. In the reactions of stilbene and 1,1-diphenylethylene, 2e also exhibited good reactivity (Fig. 4, 5ae and 5af). The superiority of this reagent was further manifested in the direct fluorosulfonylation of triarylethylenes (4ag-4an), and the desired sulfonyl fluoride products (5ag-5an) can be obtained in good to high yields (41–92%). In contrast, the previous method with FSO2Cl gave 5ag in a quite poor yield (18%)50. The low E/Z ratios in some cases probably resulted from the E/Z isomerization of the starting olefins, suggested by the tracking experiment with 4aj and 4ak (Fig. 4, b), in which both starting olefin 4aj & 4ak was found rapidly isomerized into Z/E 1:1 ratio in 10 min. Further, more examples of triarylethylenes also afforded the products (5ay-5ba, in the Supplementary Methods) in ~1:1 Z/E ratios. Moreover, as shown in Fig. 4c, biorelevant molecules, such as cinnamic alcohol, menthol, ciprofibrate, thymol, galactose, abietic acid, chromene, tyrosine, estrone, and febuxosate-derived alkenes, can all be readily modified with this reagent, affording the corresponding sulfonyl fluorides (5ao-5ax) with a good functional group compatibility and high structural diversity.
a Scope of cyclic, di- and tri-substituted olefins. b Tracking the E/Z isomerization of substrate 4aj and 4ak. c Late-stage modification of natural products. aAll reactions were performed on 0.1 or 0.2 mmol scales . bIn parentheses are yields with FSO2Cl. cWith 1.0 eq. of K2CO3. dWith 2.0 eq. of 2e. *Reactions on 1.0 mmol scale.
To our delight, this reagent (2e) could finally allow the development of the alkoxy-fluorosulfonyl difunctionalization reaction of olefins with alcohols as a nucleophile. As shown in Fig. 5, in the presence of EtOH as nucleophile, this alkoxy-fluorosulfonyl difunctionalization protocol could readily accommodate a variety of styrenes (7a-7l) and electron-rich olefins (7m-7p). Reactions with other alcohols including methanol, isopropanol also proceeded well (7q, 7r). Further, formic acid (7 s) and acetic acid (7t) were also suitable nucleophiles, affording the corresponding ester product in 72% and 55% yield, respectively. It is worth mentioning that many β-hydoxy or alkoxyl sulfonic acids, sulfonamides, and related compounds were found showing various biological activity60,61,62, while the corresponding β-alkoxyl sulfonyl fluorides could server as precursors to access these molecules via SuFEx reactions1,2,3,4.
To gain some mechanistic insight into the reaction, the radical scavenger 2,2,6,6-tetramethyl-1-piperidinoxyl (TEMPO, 2.0 equiv.) was added to the reaction mixture of 1a and 2e under standard conditions. The reaction was found completely inhibited, and no fluorosulfonylation product 3aa was observed (Fig. 6 and Eq. 1). To further examine the involvement of FSO2 radical in the reaction, a radical-clock experiment was conducted with cyclopropylstyrene (8), a well-known radical probe63,64, and the cyclization product 9 can be isolated in 21% yield. This is in accord with a redox mechanism, and suggested that fluorosulfonyl radical addition to the double bond, followed by a subsequent radical ring-opening of the three-membered cycle and radical cyclization, should be involved (Fig. 6 and Eq. 2)50,63,64. On the other hand, as demonstrated in Fig. 5, carbocationic species can be trapped by alcohols. For comparison, we also performed the reaction with FSO2Cl, in which no formation of 7a was observed under the same reaction conditions (Fig. 6b). In contrast, with FABI 2e as the radical precursor, the desired ether product 7a can be isolated in high yield, which further manifested the superiority of the newly developed FABI agents.
a Mechanistic study: (1) the reaction was performed under standard conditions with TEMPO (2.0 equiv.); (2) the reaction was performed under standard conditions with 8 as a radical probe. b Trapping experiments was performed with styrene (0.1 mmol), radical precursor (2.0–3.0 equiv.), EtOH (10.0 equiv.), fac-Ir(ppy)3 (1 mol%), NaOAc (0.1 mmol), and 1,4-dioxane (1 mL) under the irradiation of 6 W blue LEDs. c Mechanistic proposal for this photoredox radical fluorosulfonylation with FABI 2e. TEMPO = 2,2,6,6-tetramethyl-1-piperidinoxyl.
According to these results, a possible reaction mechanism for this radical fluorosulfonylation reaction using FABI 2e as the radical precursor is proposed in Fig. 6c. First, under the irradiation of blue LEDs, the photocatalyst (IrIII) is excited (E1/2IV/III* = −1.73 V vs SCE)65 and then undergoes a single electron transfer (SET) to the redox-active radical precursor 2e (E1/2red = −1.07 V vs SCE). Upon the acceptance of one electron, 2e′ would undergo a homolytic fission of the N-S bond, and give the desired FSO2 radicals. Subsequently, the addition of FSO2• to styrene furnishes the key radical intermediate Int-1. Oxidation of Int-1 by IrIV affords the cationic species Int-2, which can be deprotonated to give 3aa, while trapping Int-2 with alcohols (R’OH) could afford the difunctionalization product 7. It is worth mentioning that when FSO2Cl was used as FSO2• precursor, it was found unable to establish this redox cascade difunctionalization reaction as shown in Fig. 6B, probably due to the fast radical chain mechanism (Fig. 2B, path I) preventing the SET oxidation of the radical intermediate Int-150,51. Further, considering the other reagents 2a-c have similar redox potentials (E1/2red = −1.03−1.09 V vs SCE, see the Supplementary Information) as 2e, the presence of both a benzo-moiety and 2-aryl group in the reagents (FABI 2d and 2e) could probably facilitate the extrusion of the desired FSO2 radicals by enhancing the driving force of re-aromatization in the step from 2e′ to 2e″.
Discussion
In summary, 1-fluorosulfonyl benzoimidazolium triflate (FABI) salts have been demonstrated as an effective redox-active fluorosulfonyl radical precursor, featuring its solid state, bench-stable characters, convenience to handle, and good tolerance of functional groups. This radical fluorosulfonylation method allows for a facile access to various vinyl sulfonyl fluorides from olefinic substrates, with remarkable good compatibility to electron-rich substrates and triaryl ethylenes, in comparison with the methods established with the known FSO2 radical precursor. In particular, FABI could allow the radical fluorosulfonylation proceding through a photoredox pathway and thus make it possible to develop the alkoxy-fluorosulfonylation reaction of olefins via trapping the carbocationic intermediates. We expect that the FABI reagents66 serving as a convenient and effective radical precursor would bring about the design and development of many radical fluorosulfonylation reactions, and further benefit the related study in the context of chemical biology and drug discovery in the future.
Methods
General procedure
The fac-Ir(ppy)3 (1 mol%) and FABI 2e (2.0 or 3.0 equivalents) were weighed into an oven-dried Schlenk tube, followed by the addition of anhydrous 1,4-dioxane (4.0 mL, 0.025 M) and olefin substrate (0.1 mmol) under argon. The reaction mixture was allowed to stir at room temperature under irradiation with blue LEDs for 12 h. Purification by column chromatography or preparative thin-layer chromatography on silica gel gave the desired pure product. Full experimental details and characterization of new compounds can be found in the Supplementary Methods and Supplementary Figs. 11–238.
Data availability
The authors declare that all data generated in this study are provided in the article and the Supplementary Information file, and are also available from the corresponding author upon request.
References
Dong, J., Krasnova, L., Finn, M. G. & Sharpless, K. B. Sulfur(VI) fluoride exchange (SuFEx): another good reaction for click chemistry. Angew. Chem. Int. Ed. 53, 9430–9448 (2014).
Barrow, A. S. et al. The growing applications of SuFEx click chemistry. Chem. Soc. Rev. 48, 4731–4758 (2019).
Xu, L. & Dong, J. Click chemistry: evolving on the fringe. Chin. J. Chem. 38, 414–419 (2020).
Lee, C. et al. The emerging applications of sulfur(VI) fluorides in catalysis. ACS Catal. 11, 6578–6589 (2021).
Lou, T. S.-B. & Willis, M. C. Sulfonyl fluorides as targets and substrates in the development of new synthetic methods. Nat. Rev. Chem. 6, 146–162 (2022).
Ungureanu, A., Levens, A., Candish, L. & Lupton, D. W. N-Heterocyclic carbene catalyzed synthesis of δ-sultones via α,β-unsaturated sulfonyl azolium intermediates. Angew. Chem. Int. Ed. 54, 11780–11784 (2015).
Meng, G. et al. Modular click chemistry libraries for functional screens using a diazotizing reagent. Nature 574, 86–89 (2019).
Smedley, C. J. et al. Bifluoride ion mediated SuFEx trifluoromethylation of sulfonyl fluorides and iminosulfur oxydifluorides. Angew. Chem. Int. Ed. 58, 4552–4556 (2019).
Wei, M. et al. A broad-spectrum catalytic amidation of sulfonyl fluorides and fluorosulfates. Angew. Chem. Int. Ed. 60, 7397–7404 (2021).
Smedley, C. J. et al. Accelerated SuFEx click chemistry for modular synthesis. Angew. Chem. Int. Ed. 61, e202112375 (2022).
Dong, J., Sharpless, K. B., Kwisnek, L., Oakdale, J. S. & Fokin, V. V. SuFEx-based synthesis of polysulfates. Angew. Chem. Int. Ed. 53, 9466–9470 (2014).
Gao, B. et al. Bifluoride-catalysed sulfur(VI) fluoride exchange reaction for the synthesis of polysulfates and polysulfonates. Nat. Chem. 9, 1083–1088 (2017).
Wang, H. et al. SuFEx-based polysulfonate formation from ethenesulfonyl fluoride–amine ddducts. Angew. Chem. Int. Ed. 56, 11203–11208 (2017).
Cao, Z. et al. Preparation of aryl polysulfonates via a highly efficient SuFEx click reaction, their controllable degradation and functionalized behavior. Polym. Chem. 11, 3120–3124 (2020).
Hmissa, T. et al. Autocatalytic synthesis of bifluoride ionic liquids by SuFEx click chemistry. Angew. Chem. Int. Ed. 57, 16005–16009 (2018).
Durie, K. et al. SuFEx postpolymerization modification kinetics and reactivity in polymer brushes. Macromolecules 51, 297–305 (2018).
Yang, C., Flynn, J. P. & Niu, J. Facile synthesis of sequence-regulated synthetic polymers using orthogonal SuFEx and CuAAC click reactions. Angew. Chem. Int. Ed. 57, 16194–16199 (2018).
Narayanan, A. & Jones, L. H. Sulfonyl fluorides as privileged warheads in chemical biology. Chem. Sci. 6, 2650–2659 (2015).
Jones, L. H. & Kelly, J. W. Structure-based design and analysis of SuFEx chemical probes. RSC Med. Chem. 11, 10–17 (2020).
Grygorenko, O. O., Volochnyuk, D. M. & Vashchenko, B. V. Emerging building blocks for medicinal chemistry: recent synthetic advances. Eur. J. Org. Chem. 47, 6478–6510 (2021).
Brouwer, A. J. et al. Peptido sulfonyl fluorides as new powerful proteasome inhibitors. J. Med. Chem. 55, 10995–11003 (2012).
Dubiella, C. et al. Selective inhibition of the immunoproteasome by ligand-induced crosslinking of the active site. Angew. Chem. Int. Ed. 53, 11969–11973 (2014).
Chen, W. et al. Arylfluorosulfates inactivate intracellular lipid binding protein(s) through chemoselective SuFEx reaction with a binding site Tyr Residue. J. Am. Chem. Soc. 138, 7353–7364 (2016).
Zhao, Q. et al. Broad-spectrum kinase profiling in live cells with lysine-targeted sulfonyl fluoride probes. J. Am. Chem. Soc. 139, 680–685 (2017).
Guardiola, S. et al. Targeted covalent inhibition of prolyl oligopeptidase (POP): discovery of sulfonylfluoride peptidomimetics. Cell Chem. Biol. 25, 1031–1037 (2018).
Uematsu, S., Tabuchi, Y., Ito, Y. & Taki, M. Combinatorially screened peptide as targeted covalent binder: alteration of bait-conjugated peptide to reactive modifier. Bioconjug. Chem. 29, 1866–1871 (2018).
Artschwager, R. et al. Potent and highly selective inhibitors of the proteasome trypsin-like site by incorporation of basic side chain containing amino acid-derived sulfonyl fluorides. J. Med. Chem. 61, 5395–5411 (2018).
Liu, Z. et al. SuFEx click chemistry enabled late-stage drug functionalization. J. Am. Chem. Soc. 140, 2919–2925 (2018).
Martín-Gago, P. & Olsen, C. A. Arylfluorosulfate-based electrophiles for covalent protein labeling: a new addition to the arsenal. Angew. Chem. Int. Ed. 58, 957–966 (2019).
Mortenson, D. E. et al. “Inverse drug discovery” strategy to identify proteins that are targeted by latent electrophiles as exemplified by aryl fluorosulfates. J. Am. Chem. Soc. 140, 200–210 (2018).
Li, Q. et al. Developing covalent protein drugs via proximity-enabled reactive therapeutics. Cell 182, 85–97 (2020).
Zheng, Q. et al. Sulfur [18F]fluoride exchange click chemistry enabled ultrafast late-stage radiosynthesis. J. Am. Chem. Soc. 143, 3753–3763 (2021).
Zhang, J. et al. Identification of simple arylfluorosulfates as potent agents against resistant bacteria. Proc. Nat. Acad. Sci. usa 118, e2103513118 (2021).
Davies, A. T., Curto, J. M., Bagley, S. W. & Willis, M. C. One-pot palladium-catalyzed synthesis of sulfonyl fluorides from aryl bromides. Chem. Sci. 8, 1233–1237 (2017).
Laudadio, G. et al. Sulfonyl fluoride synthesis through electrochemical oxidative coupling of thiols and potassium fluoride. J. Am. Chem. Soc. 141, 11832–11836 (2019).
Liu, S., Huang, Y., Xu, X.-H. & Qing, F.-L. Fluorosulfonylation of arenediazonium tetrafluoroborates with Na2S2O5 and N-fluorobenzenesulfonimide. J. Fluor. Chem. 240, 109653 (2020).
Xu, T. et al. Decarboxylative Thiolation of redox-active esters to thioesters by merging photoredox and copper catalysis. Org. Lett. 22, 3692–3696 (2020).
Zhong, T. et al. Photoredox-catalyzed aminofluorosulfonylation of unactivated olefins. Chem. Sci. 12, 9359–9365 (2021).
Magre, M. & Cornella, J. Redox-neutral organometallic elementary steps at bismuth: catalytic synthesis of aryl sulfonyl fluorides. J. Am. Chem. Soc. 143, 21497–21502 (2021).
Moku, B., Fang, W.-Y., Leng, J., Kantchev, E. A. B. & Qin, H.-L. Rh(I)–diene-catalyzed addition of (hetero)aryl functionality to 1,3-dienylsulfonyl fluorides achieving exclusive regioselectivity and high enantioselectivity: generality and mechanism. ACS Catal. 9, 10477–10488 (2019).
Xu, R., Xu, T., Yang, M., Cao, T. & Liao, S. A rapid access to aliphatic sulfonyl fluorides. Nat. Commun. 10, 3752 (2019).
Smedley, C. J. et al. Diversity Oriented Clicking (DOC): divergent synthesis of sufexable pharmacophores from 2-substituted-alkynyl-1-sulfonyl fluoride (SASF) Hubs. Angew. Chem. Int. Ed. 59, 12460–12469 (2020).
Andrews, J. A., Pantaine, L. R. E., Palmer, C. F., Poole, D. L. & Willis, M. C. Sulfinates from amines: a radical approach to alkyl sulfonyl derivatives via donor–acceptor activation of pyridinium salts. Org. Lett. 23, 8488–8493 (2021).
Frye, N. L., Daniliuc, C. G. & Studer, A. Radical 1-fluorosulfonyl-2-alkynylation of unactivated alkenes. Angew. Chem. Int. Ed. 61, e202115593 (2022).
Kwon, J. & Kim, B. M. Synthesis of arenesulfonyl fluorides via sulfuryl fluoride incorporation from arynes. Org. Lett. 21, 428–433 (2019).
Lee, C., Ball, N. D. & Sammis, G. M. One-pot fluorosulfurylation of Grignard reagents using sulfuryl fluoride. Chem. Commun. 55, 14753–14756 (2019).
Guo, T. et al. A new portal to SuFEx click chemistry: a stable fluorosulfuryl imidazolium salt emerging as an “F−SO2+” donor of unprecedented reactivity, selectivity, and scope. Angew. Chem. Int. Ed. 57, 2605–2610 (2018).
Zhou, H. et al. Introduction of a crystalline, shelf-stable reagent for the synthesis of sulfur(VI) fluorides. Org. Lett. 20, 812–815 (2018).
Zeng, X., Beckers, H. & Willner, H. Thermally persistent fluorosulfonyl nitrene and unexpected formation of the fluorosulfonyl radical. J. Am. Chem. Soc. 135, 2096–2099 (2013).
Nie, X. et al. Radical fluorosulfonylation: accessing alkenyl sulfonyl fluorides from alkenes. Angew. Chem. Int. Ed. 60, 3956–3960 (2021).
Nie, X. et al. Introducing a new class of sulfonyl fluoride hubs via radical chloro-fluorosulfonylation of alkynes. Angew. Chem. Int. Ed. 60, 22035–22042 (2021).
Qin, H.-L., Zheng, Q., Bare, G. A. L., Wu, P. & Sharpless, K. B. A heck–matsuda process for the synthesis of β-arylethenesulfonyl fluorides: selectively addressable bis-electrophiles for SuFEx click chemistry. Angew. Chem. Int. Ed. 55, 14155–14158 (2016).
Chinthakindi, P. K. et al. A synthesis of “dual warhead” β-aryl ethenesulfonyl fluorides and one-pot reaction to β-Sultams. Org. Lett. 19, 480–483 (2017).
Zha, G.-F. et al. Palladium-catalyzed fluorosulfonylvinylation of organic iodides. Angew. Chem. Int. Ed. 56, 4849–4852 (2017).
Chen, X.-Y., Wu, Y., Zhou, J., Wang, P. & Yu, J.-Q. Synthesis of β-arylethenesulfonyl fluoride via Pd-catalyzed nondirected C–H alkenylation. Org. Lett. 21, 1426–1429 (2019).
Lou, T. S.-B., Bagley, S. W. & Willis, M. C. Cyclic alkenylsulfonyl fluorides: palladium-catalyzed synthesis and functionalization of compact multifunctional reagents. Angew. Chem. Int. Ed. 58, 18859–18863 (2019).
Cao, L., Weidner, K. & Renaud, P. Metal-free, radical addition to alkenes via desulfitative chlorine atom transfer. Adv. Synth. Catal. 353, 3467–3472 (2011).
Dong, J.; Yang Q.; Guo T.; Zhan X. & Meng G. Fluorosulfonyl-containing Compound, Intermediate Thereof, Preparation Method Therefor And Use Thereof, WO2019101132 A1 (2019).
Wayner, D. D. M. & Houmam, A. Redox properties of free radicals. Acta Chem. Scand. 52, 377–384 (1998).
Werder, M., Hauser, H. & Carreira, E. M. Synthesis and in vitro evaluation of inhibitors of intestinal cholesterol absorption. J. Med. Chem. 48, 6035–6053 (2005).
Ashley, G. W., Henise, J., Reid, R. & Santi, D. V. Hydrogel drug delivery system with predictable and tunable drug release and degradation rates. Proc. Natl Acad. Sci. USA 110, 2318 (2013).
Santi, D. V., Schneider, E. L. & Ashley, G. W. Macromolecular prodrug that provides the irinotecan (CPT-11) active-metabolite SN-38 with ultralong half-life, low cmax, and low glucuronide formation. J. Med. Chem. 57, 2303–2314 (2014).
Feng, Z., Min, Q.-Q., Zhao, H.-Y., Gu, J.-W. & Zhang, X. A general synthesis of fluoroalkylated alkenes by palladium-catalyzed heck-type reaction of fluoroalkyl bromides. Angew. Chem. Int. Ed. 54, 1270–1274 (2015).
Feng, F.-F., Ma, J.-A. & Cahard, D. Radical 1,5-chloropentafluorosulfanylation of unactivated vinylcyclopropanes and transformation into α-SF5 ketones. J. Org. Chem. 86, 13808–13816 (2021).
Kelly, C. B. et al. Preparation of visible-light-activated metal complexes and their use in photoredox/nickel dual catalysis. Nat. Protoc. 1, 472–492 (2017).
Liao, S., Wang, P. & Zhang, H. Fluorosulfonyl radical reagent, preparation method and application. CN 113248444 A (2021).
Acknowledgements
We gratefully acknowledge the Recruitment Program of Global Experts (S.L.), Natural Science Foundation of China (No. 21602028, S.L.), Beijing National Laboratory for Molecular Sciences (BNLMS201913, S.L.) and Fuzhou University for the financial support.
Author information
Authors and Affiliations
Contributions
P.W. developed the reactions, and performed the reaction scope investigation, mechanistic study. H.Z. and X.N. participated in the synthesis of substrates, T.X. helped the study of the reaction mechanism. S.L. conceived this concept and prepared this manuscript with feedback from P.W.
Corresponding author
Ethics declarations
Competing interests
The authors declare no competing interests.
Peer review
Peer review information
Nature Communications thanks Glenn Sammis and the anonymous reviewer(s) for their contribution to the peer review of this work.
Additional information
Publisher’s note Springer Nature remains neutral with regard to jurisdictional claims in published maps and institutional affiliations.
Supplementary information
Rights and permissions
Open Access This article is licensed under a Creative Commons Attribution 4.0 International License, which permits use, sharing, adaptation, distribution and reproduction in any medium or format, as long as you give appropriate credit to the original author(s) and the source, provide a link to the Creative Commons license, and indicate if changes were made. The images or other third party material in this article are included in the article’s Creative Commons license, unless indicated otherwise in a credit line to the material. If material is not included in the article’s Creative Commons license and your intended use is not permitted by statutory regulation or exceeds the permitted use, you will need to obtain permission directly from the copyright holder. To view a copy of this license, visit http://creativecommons.org/licenses/by/4.0/.
About this article
Cite this article
Wang, P., Zhang, H., Nie, X. et al. Photoredox catalytic radical fluorosulfonylation of olefins enabled by a bench-stable redox-active fluorosulfonyl radical precursor. Nat Commun 13, 3370 (2022). https://doi.org/10.1038/s41467-022-31089-7
Received:
Accepted:
Published:
DOI: https://doi.org/10.1038/s41467-022-31089-7
This article is cited by
-
Electroreductive hydroxy fluorosulfonylation of alkenes
Nature Communications (2023)
Comments
By submitting a comment you agree to abide by our Terms and Community Guidelines. If you find something abusive or that does not comply with our terms or guidelines please flag it as inappropriate.