Abstract
Extramedullary multiple myeloma (EMM) is an aggressive form of multiple myeloma (MM). This study represents the most comprehensive next-generation sequencing analysis of EMM tumors (N = 14) to date, uncovering key molecular features and describing the tumor microenvironment. We observed the co-occurrence of 1q21 gain/amplification and MAPK pathway mutations in 79% of EMM samples, suggesting that these are crucial mutational events in EMM development. We also demonstrated that patients with mutated KRAS and 1q21 gain/amplification at the time of diagnosis have a significantly higher risk of EMM development (HR = 2.4, p = 0.011) using data from a large CoMMpass dataset. We identified downregulation of CXCR4 and enhanced cell proliferation, along with reduced expression of therapeutic targets (CD38, SLAMF7, GPRC5D, FCRH5), potentially explaining diminished efficacy of immunotherapy. Conversely, we identified significantly upregulated EZH2 and CD70 as potential future therapeutic options. For the first time, we report on the tumor microenvironment of EMM, revealing CD8+ T cells and NK cells as predominant immune effector cells using single-cell sequencing. Finally, this is the first longitudinal study in EMM revealing the molecular changes from the time of diagnosis to EMM relapse.
Similar content being viewed by others
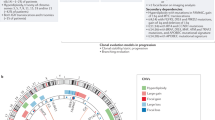
Introduction
Extramedullary multiple myeloma (EMM) is an aggressive form of multiple myeloma (MM), the second most common blood cancer, which is characterized by the clonal proliferation of plasma cells (PCs) within bone marrow (BM) [1]. In EMM, malignant PCs become independent of the BM microenvironment and infiltrate other tissues and organs, creating soft tissue tumors [2]. Therefore, EMM is associated with treatment resistance and short survival [3, 4]. In clinical setting, it is important to distinguish EMM from the less aggressive form, paraskeletal (bone-related) MM, which manifests as the presence of soft tissue lesions adjacent to the bone [1, 5]. The incidence of EMM is reported to be 1.7–4.5% at diagnosis (primary EMM) and up to 43% at relapse (secondary EMM) [1, 6, 7]. Recent clinical trials in heavily pretreated MM patients who are “triple class exposed” (previous proteasome inhibitor [PI], immunomodulatory drug [IMiD], and anti-CD38 monoclonal antibody [mAb]) reported a much higher incidence of EMM than in the past [8, 9]. This observation is probably associated with longer survival of MM patients due to novel treatment options, as well as improved availability of modern imaging techniques, such as PET/CT. Thus, EMM is becoming a clinically relevant issue and one of the “hot topics” in the MM community alongside with circulating tumor PCs, which may be responsible for EMM spread and possess great prognostic value [10,11,12]. The molecular mechanisms mediating EMM development, as well as the composition of the tumor microenvironment, are still poorly understood but crucial for the efficacy of novel immunotherapy.
No detailed genomic or transcriptomic profiling has been carried out for EMM. In addition to a limited number of samples, studies focusing on chromosomal aberrations are usually biased by a preselected set of FISH targets [13, 14], and small somatic mutations are analyzed mostly with targeted panels, excluding the vast majority of human genes [15, 16]. Similarly, almost no transcriptomic data obtained directly from EMM tumor cells exist [17, 18].
In this study, we performed the most comprehensive investigation of EMM cells to date, combining data obtained by FISH, whole exome sequencing (WES), bulk RNA sequencing (RNA-seq), single-cell RNA sequencing (scRNA-seq), and flow cytometry in 14 patients with EMM. Importantly, we obtained genomic and transcriptomic data from eight paired samples of BM aberrant PCs from the time of diagnosis and compare them with EMM cells from the time of relapse which, to the best of our knowledge, represents the first longitudinal study in EMM.
Methods
Patients and data collection
All samples were collected between 2014 and 2022 at the Department of Hematooncology, University Hospital Ostrava: 14 EMM samples from biopsies of soft tissue tumors and 14 BM samples from newly diagnosed MM (NDMM), 8 of them paired samples stored at the biobank from the time of diagnosis and 6 unpaired, and 14 unpaired BM samples from relapsed/refractory MM (RRMM) patients without evidence of EMM. All samples were used for RNA sequencing (n = 42). EMM and paired NDMM samples were also used for WES (n = 22), and some EMM samples were processed for single-cell sequencing (n = 5) (Fig. 1). All EMM samples were processed fresh immediately (max. 1 h) after surgery. Patients were treated in a real-world setting according to institutional guidelines. Biopsy of the soft tissue tumor was performed when clinically indicated to confirm the EMM diagnosis. The clinical characteristics of the EMM patients and treatment summaries are provided in Supplementary Table 1 and 2. The clinical characteristics of the RRMM and unpaired NDMM patients are described in Supplementary Table 3. The study was conducted in accordance with the principles of the Declaration of Helsinki and was approved by institutional ethics committee under number 511/2022. All patients provided written informed consent.
Fluorescence in situ hybridization
For EMM tumor samples, a 2 × 5 × 5 mm slice of fresh tumor was touched several times at one spot against a methanol-cleaned uncoated slide, which was then fixed in 70% ethanol and air-dried. FISH analysis was performed using the following probes: MetaSystems XL RB1/DLEU/LAMP, XL IGH plus, XL P53, XL 1p32/1q21, XL 5p15/9q22/15q22 Hyperdiploidy, XL t(11;14), XL t(4;14), and XL t(14;16). A total of 100 cells were evaluated. FISH analysis of probe hybridization was performed with a 100× objective fluorescence microscope (Olympus BX41) with single and double emission filters. The protocol for samples from NDMM and RRMM samples is described in the Supplementary Methods.
Fluorescence-activated cell sorting
EMM samples were collected in tubes containing normal saline and mechanically disintegrated immediately after surgery. BM aspirates from NDMM and RRMM patients were collected in tubes containing EDTA and processed within 24 h. Filtered cell suspensions from EMM and BM samples were subjected to erythrocyte lysis in NH4Cl-based lysing solution. Cells were stained with multiple fluorescence-labeled mAbs: CD38-FITC/CD45-PB/CD56-PE/CD19-PC7/CD138-APC. Cells were sorted using a BD FACSAria III (BD Biosciences) equipped with 405, 488, 561, and 633 nm excitation lasers. Pathological PCs were gated according to their immunophenotype and sorted into RPMI media containing 10% fetal bovine serum. Purity exceeded 95%.
DNA/RNA isolation and quality assessment
Sorted aberrant PCs were subjected to DNA and RNA isolation using the AllPrep DNA/RNA Micro Kit (Qiagen, Germantown, MD) following the manufacturer’s protocol. The concentration of isolated DNA and RNA was determined on a Qubit 2.0 fluorometer (Life Technologies, ThermoFisher Scientific, Waltham, MA, USA). RNA quality was assessed on an Agilent 2200 Tapestation (Agilent Technologies, Santa Clara, CA, USA) using High Sensitivity RNA ScreenTape.
RNA sequencing
The total RNA (5 ng) from 42 samples was used for library preparation using SMARTer Stranded Total RNA-Seq Kit v2 - Pico Input Mammalian (Takara Bio, San Jose, CA, USA). Briefly, RNA was converted to cDNA, followed by the addition of Illumina adaptors and barcodes by five cycles of PCR. Next, libraries were submitted to depletion of ribosomal cDNA using ZapR v2 enzyme and R-probes v2. Finally, libraries were amplified by 14 cycles of PCR. Libraries were pooled in equimolar ratios and the final library pool sequenced using the Illumina platform (Illumina Inc., San Diego, USA) at Macrogen Europe (the Netherlands). Sequencing resulted in an average number of 16.4 (63%), 8.0 (31%) and 1.5 (6%) million readsmapped to exonic, intronic and intergenic regions, respectively. The RNA-seq data analysis is described in detail in the Supplementary Methods.
Whole exome sequencing
Genomic DNA from 22 samples was used for WES. For each patient, T-lymphocytes were sequenced as the normal reference. DNA (72–200 ng) from 11 patients was used for library preparation using the Twist Comprehensive (Core + RefSeq) Human Exome kit (Twist Biosciences, USA) following the manufacturer’s protocol with enzymatic fragmentation performed by Macrogen Europe (the Netherlands). Samples from three patients in the above-mentioned cohort underwent library preparation using SureSelect Human All Exon V6 (Agilent Technologies, USA), but the data were analyzed in the same way as all samples (i.e., using BED files from Twist Comprehensive [Core+RefSeq] Human Exome kit). Sequencing was performed on an Illumina platform aiming at 200× raw coverage (150 bp pair-end reads) by Macrogen Europe (the Netherlands). Sequencing resulted in an average target coverage of 94× (range 57–133×) for tumor samples and 67× (range 50–82×) for normal samples. The WES data analysis is described in detail in the Supplementary Methods.
Single-cell analyses of EMM tumors
Single-cell RNAseq was performed using cell suspensions from five EMM tumors (5000–10,000 cells per sample) that were subjected to Chromium Next GEM Single Cell 3′ RNA reagent kit v3.1 (cat. num. 1000128, single indexing) (10x Genomics, USA) following manufacturer’s protocol. Sequencing was performed by Macrogen Europe (the Netherlands) on an Illumina sequencer. Sequencing resulted in an estimated average of 6054 cells, 34888 mean reads per cell, and 3575 median genes per cell per patient. The scRNA data analysis and flow cytometry analysis is described in detail in the Supplementary Methods.
Survival analysis
Survival analysis of Multiple Myeloma Research Foundation CoMMpass study (NCT01454297) (“CoMMpass”, N = 699, IA20) is described in detail in the Supplementary methods. We used p = 0.05 as a threshold for significance in all analyses. We performed all computations and visualization using R(v4.0.3) and survival(v3.2.11), survminer(v0.4.9), lubridate(v1.7.10), readxl(v1.3.1), and tidyverse(v1.3.1) packages.
Results
Clinical characteristics of EMM relapse
A total 14 of RRMM patients (10 men and 4 women) underwent biopsy of the soft tissue tumor, which confirmed the diagnosis of EMM (Fig. 2). Median age at the time of EMM relapse was 59 years, median time from diagnosis was 22.2 months, and median overall survival from the time of EMM relapse was only 3.6 months (Fig. 1). Median number of previous lines of therapy was 3 (range 1–6), and the majority of patients received a combination of PI, IMiDs, corticosteroids, and alkylators. Six of the 14 patients had daratumumab before EMM relapse (Supplementary Table 2). Patients with EMM relapse presented with a significant drop in serum electrophoresis defined M-protein levels compared to non-EMM relapse (median 5.8 g/L vs. 14.4 g/L; p = 0.011) at the time of relapse, but there was no difference in free light chain levels. BM PC infiltration was significantly lower in patients with EMM relapse assessed by cytology (2.8% vs. 14.4%; p = 0.023) or flow cytometry (0.5% vs. 8.3%; p = 0.05; Supplementary Fig. 1). This minimal BM infiltration of malignant PCs translated into normal blood counts in practically all patients experiencing EMM relapse (median white blood cell count 5.1 × 109 /L; median absolute neutrophil count 2.4 × 109/L; median level of hemoglobin 12.0 g/dL; median platelet count 168 × 109/L).
The left column represents whole body maximum intensity projections (MIPs), the medial and right columns fused hybrid multi-planar reconstructions (MPRs) in the coronal and sagittal plane. A 59-year-old male with multiple metabolically active EM foci (EMM13) had a histologically evaluated lesion in the right cubital fossa (green arrow, SUVmax 17.4). B 75-year-old female with active foci in the skeleton and lymph nodes (EMM14) had a histologically evaluated extramedullary lesion growing near the wall of the right maxillary sinus (green arrow, SUVmax 5.1). C 62-year-old female with multiple active foci with liver involvement (EMM08) (green arrow, SUVmax 44.4).
1q21 gain/amp is the most frequent chromosomal aberration in EMM
Out of 14 EMM samples, we detected (by FISH and/or WES) 1q21 gain or amplification (≥4 copies) spanning the CKS1B gene in 12/14 samples (86%; 5 gains and 7 amplifications) (Fig. 3A). Del(13q) including RB1 and del(17p) including TP53 were detected in 8 (57%) and 6 (43%) cases, respectively. Surprisingly, high-risk t(4;14) was present in only 2 patients (14%). We verified this finding with RNA-seq data revealing elevated FGFR3 expression and/or presence of FGFR3—IGH fusion transcripts. Detailed copy number analysis utilizing WES data further uncovered amplifications of several oncogenes, deletions of tumor suppressor genes (TSGs) [19] and deletion of CD38 in 4 out of 14 EMM samples, (Supplementary Fig. 2).
A CAs are represented by two triangles: the top-left triangle depicts aberrations detected by FISH, and the bottom-right triangle denotes CAs detected by WES. The absence of a triangle indicates unavailable data. For two patients, FISH EMM samples were only available from BM and are marked as such. B Oncoplot produced by Maftools [61] displays the most frequently mutated pathways. Columns represent samples, and rows represent mutated genes or indicate the entire pathway (in bold text and dark grey boxes). Different types of mutations are shown in different colors. The total number of mutations is visualized at the top as a bar plot. Paired BM NDMM and EMM samples are grouped together.
Members of MAPK pathway are mutated in virtually all EMM samples
Next, we identified at least one mutated gene in the MAPK signaling pathway in almost all EMM samples (13/14, 93%; Fig. 3B), with KRAS as the most frequently mutated gene in our cohort (10/14, 71%; Fig. 3B, Supplementary Fig. 3). Mutations in NF1 and NRAS were identified in 2 (14%) samples, and BRAF and HRAS were mutated in 1 (7%) sample. Mutations in the MAPK pathway were always clonal with the cancer cell fraction bearing them >0.8 (Supplementary Table 4), suggesting their emergence prior to extramedullary tumor formation. We also discovered that 57% of EMM samples had mutations in genes associated with genome integrity, with TP53 being the most frequently mutated TSG (5/14; 36%). The last group of genes frequently mutated in EMM were those belonging to the receptor tyrosine kinase (RTK) signaling pathway upstream of the MAPK pathway [20]. Further, we used RNA-seq data to demonstrate that at least 80% of the above-mentioned mutations were transcribed into mRNA and are presumably translated into final protein products (Supplementary Table 4). Notably, all EMM samples with mutated TP53 transcribed the mutated allele, with variant allele frequency (VAF) ≥ 0.9 as a sign of biallelic inactivation (Supplementary Fig. 2). All mutations in K/N/HRAS had VAF of at least 0.3 in the RNA-seq data. Of note, melphalan signature was detected only in 3/9 (33%) of patients that underwent ASCT (Supplementary Fig. 4).
Combination of 1q21 gain/amp and mutated KRAS in NDMM patients predicts higher risk of EMM development
Due to the high frequency of 1q21 gain/amp and KRAS mutations in EMM, we hypothesized that NDMM patients carrying the combination of these two abnormalities are at higher risk of EMM development. We observed this combination already at the time of MM diagnosis in 3/8 (38%) paired NDMM samples (Fig. 3). Therefore, we used data collected in the CoMMpass study (N = 699; MM patients with all required data available) that supported our hypothesis as only the combination of 1q21 gain/amp with KRAS mutations (7.6% of patients), but not any of them alone (29.8% and 17.7% with only 1q21 gain/amp or KRAS respectively), resulted in a significantly higher risk of soft tissue plasmacytoma development in univariate analysis (HR = 2.7; p = 0.002) and multivariate Cox analysis (HR = 2.4; p = 0.011) with ISS, LDH level, del(13q), and del(17p) (Supplementary Table 5). In addition, we observed virtually identical results using the Fine-Gray model for survival analysis with competing risks (soft tissue plasmacytoma development vs. death, HR = 2.4; p = 0.011). No interaction was detected between KRAS mutations or 1q21 gain/amp with del(13q) or del(17p).
Transcriptomic profiling of EMM cells suggests higher proliferation and decreased homing to BM
To further explore the biological features of EMM, we used RNA-sequencing to compare 14 EMM samples with 14 NDMM samples from which 8 samples were paired. In total, we identified 799 significantly deregulated protein coding genes (absolute log2 fold change > 1, Benjamini–Hochberg adjusted p-value < 0.05) among which 290 were downregulated and 509 upregulated (Fig. 4A). Pathway enrichment analysis revealed marked upregulation of pathways connected to cell proliferation and downregulation of pathways connected to immune response in comparison with NDMM (Fig. 4B) as well as in unrelated RRMM samples (Supplementary Fig. 5). Among the most significantly upregulated genes, we identified common biomarkers in human cancers: STC2, CACNA1C, and GAGE2A [21,22,23]. The most downregulated genes were FCGR2B, which was previously connected to PC persistence in BM and higher susceptibility to apoptosis [24], LYZ, and ITPRIP. In addition, we observed decreased expression of deubiquitinase gene OTUD1, which we recently found associated with worse prognosis in MM patients [25]. Importantly, we detected lower expression of CXCR4, which encodes a key molecule for PCs homing to the BM [2], and integrin gene ITGA6, the downregulation of which was recently connected to progression from MM to plasma cell leukemia [26]. Similarly, expression of CKS1B was increased probably due to presence of 1q21 gains. Notably, expression of interleukin 6 (IL6), a potent growth factor for PCs [18], was upregulated, suggesting autocrine regulation of EMM tumor growth. Furthermore, we observed higher production of light chains (IGL) compared to heavy chains in almost all samples (Fig. 4C). Congruent with our previous research, we detected significantly higher expression of important epigenetic mediators in EMM compared to MM: a histone methyltransferase (EZH2) and two out of three human DNA methyltransferases (DNMT1 and DNMT3B) [27]. Furthermore, we detected 33 downregulated and 62 upregulated lncRNAs. Among these, we identified upregulation of several lncRNAs previously connected to tumor progression, including EPIC1 [28], CASC9 [29], and ZFPM2-AS1 [30].
A Volcano plot showing the top three down- and upregulated genes, along with several other significantly deregulated genes between EMM (N = 14) and NDMM (N = 14) samples. B The top five up- and downregulated hallmark pathways detected by GSEA based on the aforementioned comparison. C Preferential expression of the immunoglobulin light chain (IGL) over the heavy chain (IGH) in EMM samples.
Therapeutically relevant targets are downregulated in EMM cells
As the efficacy of anti-myeloma therapy, including novel immunotherapy, is suboptimal in EMM [8, 27, 31,32,33], we focused on the expression of immunotherapeutic targets, which could predict treatment efficacy. To compare the expression of key molecular targets for MM treatment, we used the same RNA-seq data as in the previous experiments (Fig. 4). We also performed differential expression analysis using only paired samples (N = 8) to better capture the longitudinal changes. In addition, we included 14 unrelated RRMM samples for comparison with advanced MM disease without EMM. We observed significantly decreased expression of important molecular targets that are currently in use for MM treatment (CD38, SLAMF7, and GPRC5D) and a strong trend of lower expression for FCRH5 (Fig. 5). Notably, no deregulation was observed for BCMA. Intriguingly, our data show high expression of EZH2 and CD70, which are promising targets in other malignancies [34,35,36]. In addition, we observed decreased expression of MHC-I molecules HLA-B and HLA-C in EMM cells, a phenomenon previously associated with a worse response to immunotherapy in other types of cancer [37]. However, this might not be clinically relevant as these genes remained between the top expressed genes (Supplementary Fig. 6).
Boxplots based on the normalized expression level of select therapeutically relevant targets, HLA-B/C, and potentially new targets EZH2 and CD70. Significance between NDMM and EMM samples is stated for both unpaired (14 vs. 14 samples) and paired settings (8 samples; depicted in color). Count normalization (used for visualization) and significance were obtained using DESeq2. RRMM samples (N = 14) represent unrelated advanced MM patients without EMM. A grey dot with a black cross represents a sample with very low expression of CD38 from Supplementary Fig. 8.
CD8+ T and NK cells are predominant immune effector cells in the EMM tumor microenvironment
To better understand the biology and composition of EMM tumors, we used, for the first time, scRNA-seq to sequence soft tissue tumors in five EMM patients. We achieved a median of 3916 cells per sample. The majority of cells were identified as aberrant PCs (median 90.5%) and the rest were mainly CD8 + T cells, NK cells, monocytes, and CD4+ T cells (median 7%, 1.8%, 0.5%, and 0.2%, respectively; Fig. 6). Importantly, we observed CD8+ T and NK cells as the most abundant immune cells with comparable proportions in flow cytometry (Supplementary Fig. 7). In addition, we observed high heterogeneity of EMM cells (Fig. 6), which could be further demonstrated on typical PC molecules; for example, the EMM cells of one patient virtually lost expression of CD38 and tumor cells from another patient had very low CD138 expression. Importantly, these data are congruent with bulk RNA-seq and flow cytometry data (Supplementary Fig. 8).
Discussion
The molecular mechanisms mediating EMM development and resistance are still poorly understood. In contrast to MM, virtually no NGS data describe EMM tumors. The need for such data has become even more evident as the incidence of EMM has dramatically increased along with the prolonged survival of MM patients. Indeed, recent clinical trials report EMM in approximately 40% of RRMM patients [8, 9]. In this study, we present the largest and most comprehensive NGS study of EMM tumor cells to date, describing the key molecular characteristics of EMM, including the expression levels of important therapeutic targets (Fig. 7). We also provide the first insights into the composition of the EMM tumor microenvironment using scRNA-seq data.
EMM cells have a higher frequency of 1q21/gain and mutations in the MAPK pathway, mainly in KRAS. Arrows indicate a higher level of proliferation and free light chains and expression of EZH2 and lower expression of CXCR4, HLA B and C, and many important therapeutic targets compared to MM. Finally, the EMM tumor microenvironment is mainly composed of CD8+ and NK cells.
Over a decade ago, Billecke et al. reported t(4;14), del(13q), and del(17p) as the most frequent cytogenetic abnormalities, found in 37%, 35%, and 32% of 19 EMM patients, respectively [13]. However, the inclusion of 1q21 in the FISH panel revealed an even higher frequency of this abnormality (13/18, 72%) [14]. Nevertheless, only around 15% of NDMM patients with 1q21 gain/amp progress to EMM [38, 39]. Importantly, analysis using genetic mutation panels identified RAS/BRAF mutations in 73% (8/11) [15] and MAPK pathway mutations in 100% of EMM samples (6/6) [16]. In our study, we detected by FISH and/or WES 1q21 gain/amp in 12/14 EMM samples and identified at least one mutated gene in the MAPK signaling pathway in almost all EMM samples (13/14, 93%), with KRAS as the most frequently mutated gene (10/14, 71%).
Based on our and previously published data we hypothesize that EMM development is mostly driven by the interplay between 1q21 gain/amp and mutations in the MAPK pathway, as we detected this combination in 11/14 patients (79%). In particular, KRAS was mutated at a markedly higher frequency (71%) than previously reported in NDMM (21.8%) [40] or RRMM (19%) [41]. Furthermore, these mutations could often be detected already at the time of diagnosis. Therefore, we tested our hypothesis on patients from a CoMMpass dataset (N = 699) and showed that patients with mutated KRAS and 1q21 gain/amp have a significantly higher risk of EMM development (HR = 2.4, p = 0.011). This may be particularly important in the near future when such information could easily be available with the implementation of diagnostic gene panels in routine clinical practice [42, 43] and may influence the choice of patient-tailored therapy. Despite the exact molecular mechanisms still remaining elusive, we speculate that both mutations in KRAS and gain/amp of 1q21 can synergistically over-activate the MAPK pathway through constitutively active RAS signaling [44] and over-expression of CKS1B [45], respectively, resulting in increased cell proliferation, dissemination, and resistance [46]. In fact, CKS1B was the only previously suggested myeloma driver on 1q21 [47] that was significantly upregulated in our analysis.
The effect of any routinely used anti-myeloma drugs on the increased incidence of EMM development remains unclear. Higher incidence of secondary EMM in the era of novel drugs is mainly explained by prolonged survival and improved imaging techniques [1]. No evidence of increased risk of EMM was observed for lenalidomide-bortezomib combinations [48], nevertheless this question has been raised dominantly after the introduction of CD38 targeted therapy. Despite limited numbers we detected relatively high occurrence of CD38 deletion in 4/14 of EMM samples in total and in 2/7 of EMM samples pretreated with anti-CD38 mAbs. Regarding patients who underwent ASCT (N = 9), we observed relatively low contribution of HD melphalan on overall mutational burden.
Though genomic changes have the potential to provide insights into the mutational events presumably leading to EMM development, especially when paired NDMM samples are available, they fail to offer sufficient understanding of the actual phenotype of EMM cells. Unfortunately, our current knowledge of gene expression in EMM is limited to a gene expression profiling experiment involving six patients conducted approximately 20 years ago [17], four single-cell EMM samples obtained from ascites and pleural effusions [18], and a recent brief communication by our group focusing primarily on CD38 expression in five paired EMM samples [27]. Therefore, this study represents the largest transcriptomic analysis of soft tissue EMM samples to date.
We revealed expression pattern towards higher proliferation rate of EMM cells, as previously demonstrated by a high proliferation index determined during histological examination [49]. The increased proliferation is likely stimulated in an autocrine manner through the production of IL6, which is not typically produced by PCs or MM cells [18]. Consistent with a case report describing tumor cells in a patient with skin-related EMM, we observed decreased expression of CXCR4, the protein product of which is responsible for the normal homing of PCs to BM [50].
Intriguingly, based on experiments with mouse models, Roccaro et al. suggested that higher expression of CXCR4 mediates EMM development through the acquisition of an epithelial-to-mesenchymal (ETM) phenotype [51]. As our human samples likely do not represent the ETM phenotype responsible for disease spread, but rather cells from well-established solid-like tumors, we cannot exclude the possibility of higher CXCR4 production in the early stages of EMM development.
Previously published data and current results show upregulation of chromatin modifiers EZH2, DNMT1 and DNMT3B in the EMM samples [27]. This suggests that changes in gene expression during the EMM stage could be regulated by epigenetic mechanisms. Importantly, inhibition of EZH2 is currently used in the treatment for other malignancies, including follicular lymphoma; and in MM it can lead to re-expression of CD38 [34, 52].
Immunotherapy is the most promising therapeutic modality for MM patients. Three naked mAbs are currently FDA approved: daratumumab and isatuximab targeting CD38, and elotozumab targeting SLAMF7 [53,54,55]. There are also two FDA-approved CAR-T products, both targeting BCMA: cilta-cel and ide-cel [56, 57]. Finally, there are also two FDA-approved bispecific antibodies (bsAbs) targeting BCMA (teclistamab and elranatamab) and one targeting GPRC5D (talquetamab) [8, 33, 58]. Many others are under clinical development, such as cevostamab targeting FCRH5 [59]. The efficacy of these agents is dependent on the patient´s immune effector cells, as well as on the expression level of the targets on the surface of malignant PCs.
Our data revealed decreased expression of CD38, SLAMF7, GPRC5D, and FCRH5 in EMM cells but no changes in expression for BCMA. We also observed lower expression of MHC-I genes on EMM cells (HLA-B, HLA-C), which may be associated with reduced efficacy of cancer immunotherapies due to decreased recognition of malignant cells by effector T cells [37, 60]. Many recent studies reported lower efficacy of modern immunotherapy in patients with EMM relapse for daratumumab, teclistamab, talquetamab, and others [8, 27, 33]. On the other hand, we observed elevated CD70 expression in EMM. This could potentially represent a way to activate the immune system by blocking the CD27-CD70 axis [36]. This concept has already been tested in trials for solid tumors using CAR-T agents.
For further elucidation of immunotherapy efficacy, it is crucial to study the tumor microenvironment of EMM, which has not been described to date. Previously, Ryu et al. reported the presence of several immune populations from ascites (n = 2) and pleural effusion (n = 1) samples from patients with EMM [18]. However, these samples do not represent true soft tissue EMM tumors. Therefore, we provide the first insight into EMM tumors at single-cell resolution, showing that the majority of the tumor mass is composed of aberrant PCs, and approximately 10% of the tumor is then formed by immune cells, mainly CD8+ T cells and NK cells. These immune cell subsets might be vital for adequate cell killing by novel immunotherapeutic agents. Nevertheless, low proportion of immune cells in EMM tumors prevents detailed characterization of these populations and represent a limitation in our scRNA data. An example of the most effective therapy for EMM to date seems to be the combination of teclistamab and talquetamab targeting two different antigens [9].
Despite the limited number of EMM samples, our study stands as the most comprehensive and largest NGS study of EMM to date, given the difficulty to obtain bioptable EMM samples. Our findings suggest that the development of EMM from MM cannot be attributed to a single mutation event, but rather a combination of several genetic, and possibly epigenetic, changes. Importantly, these changes are usually not present at diagnosis, but acquired later during the disease, as demonstrated by our unprecedented longitudinal data. Furthermore, we describe significant alterations in the expression profile of EMM cells compared to MM cells in the BM, including clinically important targets and CXCR4. Additionally, our study provides the first insights into the EMM tumor microenvironment.
Data availability
The datasets generated during the current study are available in the European Genome-phenome Archive (EGA) repository under the accession numbers: EGAD50000000051- EGAD50000000053.
References
Rosiñol L, Beksac M, Zamagni E, Van de Donk NWCJ, Anderson KC, Badros A, et al. Expert review on soft-tissue plasmacytomas in multiple myeloma: definition, disease assessment and treatment considerations. Br J Haematol. 2021;194:496–507.
Bhutani M, Foureau DM, Atrash S, Voorhees PM, Usmani SZ. Extramedullary multiple myeloma. Leukemia. 2020;34:1–20.
Varettoni M, Corso A, Pica G, Mangiacavalli S, Pascutto C, Lazzarino M. Incidence, presenting features and outcome of extramedullary disease in multiple myeloma: a longitudinal study on 1003 consecutive patients. Ann Oncol. 2010;21:325–30.
Gagelmann N, Eikema D-J, Iacobelli S, Koster L, Nahi H, Stoppa A-M, et al. Impact of extramedullary disease in patients with newly diagnosed multiple myeloma undergoing autologous stem cell transplantation: a study from the Chronic Malignancies Working Party of the EBMT. Haematologica. 2018;103:890–7.
Pour L, Sevcikova S, Greslikova H, Kupska R, Majkova P, Zahradova L, et al. Soft-tissue extramedullary multiple myeloma prognosis is significantly worse in comparison to bone-related extramedullary relapse. Haematologica. 2014;99:360–4.
Bladé J, Beksac M, Caers J, Jurczyszyn A, von Lilienfeld-Toal M, Moreau P, et al. Extramedullary disease in multiple myeloma: a systematic literature review. Blood Cancer J. 2022;12:1–10.
Sevcikova S, Minarik J, Stork M, Jelinek T, Pour L, Hajek R. Extramedullary disease in multiple myeloma - controversies and future directions. Blood Rev. 2019;36:32–39.
Chari A, Minnema MC, Berdeja JG, Oriol A, van de Donk NWCJ, Rodríguez-Otero P, et al. Talquetamab, a T-cell-redirecting GPRC5D bispecific antibody for multiple myeloma. N Engl J Med. 2022;387:2232–44.
Cohen YC, Morillo D, Gatt ME, Sebag M, Kim K, Min C-K, et al. First results from the RedirecTT-1 study with teclistamab (tec) + talquetamab (tal) simultaneously targeting BCMA and GPRC5D in patients (pts) with relapsed/refractory multiple myeloma (RRMM). J Clin Oncol. 2023;41:8002–8002.
Garcés J-J, Simicek M, Vicari M, Brozova L, Burgos L, Bezdekova R, et al. Transcriptional profiling of circulating tumor cells in multiple myeloma: a new model to understand disease dissemination. Leukemia. 2020;34:589–603.
Termini R, Žihala D, Terpos E, Perez-Montaña A, Jelínek T, Raab M, et al. Circulating tumor and immune cells for minimally invasive risk stratification of smoldering multiple myeloma. Clin Cancer Res J Am Assoc Cancer Res. 2022;28:4771–81.
Jelinek T, Bezdekova R, Zihala D, Sevcikova T, Anilkumar Sithara A, Pospisilova L, et al. More than 2% of circulating tumor plasma cells defines plasma cell leukemia–like multiple myeloma. J Clin Oncol. 2023;41:1383–92.
Billecke L, Murga Penas EM, May AM, Engelhardt M, Nagler A, Leiba M, et al. Cytogenetics of extramedullary manifestations in multiple myeloma. Br J Haematol. 2013;161:87–94.
Besse L, Sedlarikova L, Greslikova H, Kupska R, Almasi M, Penka M, et al. Cytogenetics in multiple myeloma patients progressing into extramedullary disease. Eur J Haematol. 2016;97:93–100.
de Haart SJ, Willems SM, Mutis T, Koudijs MJ, van Blokland MT, Lokhorst HM, et al. Comparison of intramedullary myeloma and corresponding extramedullary soft tissue plasmacytomas using genetic mutational panel analyses. Blood Cancer J. 2016;6:e426.
Liu Y, Jelloul F, Zhang Y, Bhavsar T, Ho C, Rao M, et al. Genetic basis of extramedullary plasmablastic transformation of multiple myeloma. Am J Surg Pathol. 2020;44:838–48.
Hedvat CV, Comenzo RL, Teruya-Feldstein J, Olshen AB, Ely SA, Osman K, et al. Insights into extramedullary tumour cell growth revealed by expression profiling of human plasmacytomas and multiple myeloma. Br J Haematol. 2003;122:728–44.
Ryu D, Kim SJ, Hong Y, Jo A, Kim N, Kim H-J, et al. Alterations in the transcriptional programs of myeloma cells and the microenvironment during extramedullary progression affect proliferation and immune evasion. Clin Cancer Res. 2020;26:935–44.
Bailey MH, Tokheim C, Porta-Pardo E, Sengupta S, Bertrand D, Weerasinghe A, et al. Comprehensive characterization of cancer driver genes and mutations. Cell. 2018;173:371–.e18.
McKay MM, Morrison DK. Integrating signals from RTKs to ERK/MAPK. Oncogene. 2007;26:3113–21.
Qie S, Sang N. Stanniocalcin 2 (STC2): a universal tumour biomarker and a potential therapeutical target. J Exp Clin Cancer Res. 2022;41:161.
Chang X, Dong Y. CACNA1C is a prognostic predictor for patients with ovarian cancer. J Ovarian Res. 2021;14:88.
Patel AJ, Tan T-M, Richter AG, Naidu B, Blackburn JM, Middleton GW. A highly predictive autoantibody-based biomarker panel for prognosis in early-stage NSCLC with potential therapeutic implications. Br J Cancer. 2022;126:238–46.
Xiang Z, Cutler AJ, Brownlie RJ, Fairfax K, Lawlor KE, Severinson E, et al. FcγRIIb controls bone marrow plasma cell persistence and apoptosis. Nat Immunol. 2007;8:419–29.
Vdovin A, Jelinek T, Zihala D, Sevcikova T, Durech M, Sahinbegovic H, et al. The deubiquitinase OTUD1 regulates immunoglobulin production and proteasome inhibitor sensitivity in multiple myeloma. Nat Commun. 2022;13:6820.
Song S, Zhang J, Su Q, Zhang W, Jiang Y, Fan G, et al. Downregulation of ITGA6 confers to the invasion of multiple myeloma and promotes progression to plasma cell leukaemia. Br J Cancer. 2021;124:1843–53.
Jelinek T, Sevcikova T, Zihala D, Popkova T, Kapustova V, Broskevicova L, et al. Limited efficacy of daratumumab in multiple myeloma with extramedullary disease. Leukemia. 2022;36:288–91.
Wang Z, Yang B, Zhang M, Guo W, Wu Z, Wang Y, et al. lncRNA Epigenetic Landscape Analysis Identifies EPIC1 as an Oncogenic lncRNA that Interacts with MYC and Promotes Cell-Cycle Progression in Cancer. Cancer Cell. 2018;33:706–.e9.
Yang Y, Chen D, Liu H, Yang K. Increased expression of lncRNA CASC9 promotes tumor progression by suppressing autophagy-mediated cell apoptosis via the AKT/mTOR pathway in oral squamous cell carcinoma. Cell Death Dis. 2019;10:1–16.
Kong F, Deng X, Kong X, Du Y, Li L, Zhu H, et al. ZFPM2-AS1, a novel lncRNA, attenuates the p53 pathway and promotes gastric carcinogenesis by stabilizing MIF. Oncogene. 2018;37:5982–96.
Danhof S, Rasche L, Mottok A, Steinmüller T, Zhou X, Schreder M, et al. Elotuzumab for the treatment of extramedullary myeloma: a retrospective analysis of clinical efficacy and SLAMF7 expression patterns. Ann Hematol. 2021;100:1537–46.
Lonial S, Lee HC, Badros A, Trudel S, Nooka AK, Chari A, et al. Longer term outcomes with single-agent belantamab mafodotin in patients with relapsed or refractory multiple myeloma: 13-month follow-up from the pivotal DREAMM-2 study. Cancer. 2021;127:4198–212.
Moreau P, Garfall AL, van de Donk NWCJ, Nahi H, San-Miguel JF, Oriol A, et al. Teclistamab in Relapsed or Refractory Multiple Myeloma. N Engl J Med. 2022;387:495–505.
Duan R, Du W, Guo W. EZH2: a novel target for cancer treatment. J Hematol Oncol. 2020;13:104.
Jacobs J, Deschoolmeester V, Zwaenepoel K, Rolfo C, Silence K, Rottey S, et al. CD70: an emerging target in cancer immunotherapy. Pharmacol Ther. 2015;155:1–10.
Flieswasser T, Van den Eynde A, Van Audenaerde J, De Waele J, Lardon F, Riether C, et al. The CD70-CD27 axis in oncology: the new kids on the block. J Exp Clin Cancer Res. 2022;41:12.
Taylor BC, Balko JM. Mechanisms of MHC-I downregulation and role in immunotherapy response. Front. Immunol. 2022;13. https://www.frontiersin.org/articles/10.3389/fimmu.2022.844866. Aaccessed 2 Jul 2023.
Stork M, Sevcikova S, Minarik J, Krhovska P, Radocha J, Pospisilova L, et al. Identification of patients at high risk of secondary extramedullary multiple myeloma development. Br J Haematol. 2022;196:954–62.
Zanwar S, Ho M, Lin Y, Kapoor P, Binder M, Buadi FK, et al. Natural history, predictors of development of extramedullary disease, and treatment outcomes for patients with extramedullary multiple myeloma. Am J Hematol; n/a. https://doi.org/10.1002/ajh.27023.
Walker BA, Mavrommatis K, Wardell CP, Ashby TC, Bauer M, Davies FE, et al. Identification of novel mutational drivers reveals oncogene dependencies in multiple myeloma. Blood. 2018;132:587–97.
Ansari-Pour N, Samur M, Flynt E, Gooding S, Towfic F, Stong N, et al. Whole-genome analysis identifies novel drivers and high-risk double-hit events in relapsed/refractory myeloma. Blood. 2023;141:620–33.
Bolli N, Li Y, Sathiaseelan V, Raine K, Jones D, Ganly P, et al. A DNA target-enrichment approach to detect mutations, copy number changes and immunoglobulin translocations in multiple myeloma. Blood Cancer J. 2016;6:e467–e467.
Sudha P, Ahsan A, Ashby C, Kausar T, Khera A, Kazeroun MH, et al. Myeloma Genome Project Panel is a comprehensive targeted genomics panel for molecular profiling of patients with multiple myeloma. Clin Cancer Res. 2022;28:2854–64.
Huang L, Guo Z, Wang F, Fu L. KRAS mutation: from undruggable to druggable in cancer. Signal Transduct Target Ther. 2021;6:1–20.
Shi L, Wang S, Zangari M, Xu H, Cao TM, Xu C, et al. Over-expression of CKS1B activates both MEK/ERK and JAK/STAT3 signaling pathways and promotes myeloma cell drug-resistance. Oncotarget. 2010;1:22–33.
Burotto M, Chiou VL, Lee J-M, Kohn EC, The MAPK. pathway across different malignancies: a new perspective. Cancer. 2014;120:3446–56.
Boyle EM, Blaney P, Stoeckle JH, Wang Y, Ghamlouch H, Gagler D, et al. Multiomic mapping of acquired chromosome 1 copy number and structural variants to identify therapeutic vulnerabilities in multiple myeloma. Clin Cancer Res. 2023:CCR-22-3209.
Varga C, Xie W, Laubach J, Ghobrial IM, O’Donnell EK, Weinstock M, et al. Development of extramedullary myeloma in the era of novel agents: no evidence of increased risk with lenalidomide–bortezomib combinations. Br J Haematol. 2015;169:843–50.
Rasche L, Bernard C, Topp MS, Kapp M, Duell J, Wesemeier C, et al. Features of extramedullary myeloma relapse: high proliferation, minimal marrow involvement, adverse cytogenetics: a retrospective single-center study of 24 cases. Ann Hematol. 2012;91:1031–7.
Marchica V, Accardi F, Storti P, Mancini C, Martella E, Dalla Palma B, et al. Cutaneous localization in multiple myeloma in the context of bortezomib-based treatment: how do myeloma cells escape from the bone marrow to the skin? Int J Hematol. 2017;105:104–8.
Roccaro AM, Mishima Y, Sacco A, Moschetta M, Tai Y-T, Shi J, et al. CXCR4 regulates extra-medullary myeloma through epithelial-mesenchymal-transition-like transcriptional activation. Cell Rep. 2015;12:622–35.
Chemlal D, Varlet E, Machura A, Ovejero S, Requirand G, Robert N, et al. EZH2 targeting induces CD38 upregulation and response to anti-CD38 immunotherapies in multiple myeloma. Leukemia. 2023;37:1925–8.
Dimopoulos MA, Oriol A, Nahi H, San-Miguel J, Bahlis NJ, Usmani SZ, et al. Daratumumab, lenalidomide, and dexamethasone for multiple myeloma. N Engl J Med. 2016;375:1319–31.
Moreno L, Perez C, Zabaleta A, Manrique I, Alignani D, Ajona D, et al. The mechanism of action of the anti-CD38 monoclonal antibody isatuximab in multiple myeloma. Clin Cancer Res. 2019;25:3176–87.
Lonial S, Dimopoulos M, Palumbo A, White D, Grosicki S, Spicka I, et al. Elotuzumab therapy for relapsed or refractory multiple myeloma. N Engl J Med. 2015;373:621–31.
Berdeja JG, Madduri D, Usmani SZ, Jakubowiak A, Agha M, Cohen AD, et al. Ciltacabtagene autoleucel, a B-cell maturation antigen-directed chimeric antigen receptor T-cell therapy in patients with relapsed or refractory multiple myeloma (CARTITUDE-1): a phase 1b/2 open-label study. Lancet. 2021;398:314–24.
Munshi NC, Anderson LD, Shah N, Madduri D, Berdeja J, Lonial S, et al. Idecabtagene vicleucel in relapsed and refractory multiple myeloma. N Engl J Med. 2021;384:705–16.
Lesokhin AM, Tomasson MH, Arnulf B, Bahlis NJ, Miles Prince H, Niesvizky R, et al. Elranatamab in relapsed or refractory multiple myeloma: phase 2 MagnetisMM-3 trial results. Nat Med. 2023. https://doi.org/10.1038/s41591-023-02528-9.
Lesokhin AM, Richter J, Trudel S, Cohen AD, Spencer A, Forsberg PA, et al. Enduring responses after 1-year, fixed-duration cevostamab therapy in patients with relapsed/refractory multiple myeloma: early experience from a phase i study. Blood. 2022;140:4415–7.
Garrido F, Aptsiauri N, Doorduijn EM, Garcia Lora AM, van Hall T. The urgent need to recover MHC class I in cancers for effective immunotherapy. Curr Opin Immunol. 2016;39:44–51.
Mayakonda A, Lin D-C, Assenov Y, Plass C, Koeffler HP. Maftools: efficient and comprehensive analysis of somatic variants in cancer. Genome Res. 2018;28:1747–56.
Acknowledgements
This work was supported by the European Regional Development Fund—Project ENOCH (No. CZ.02.1.01/0.0/0.0/16_019/0000868) and Cell Coolab Ostrava—Research and Development Center for Cell Therapy in Hematology and Oncology (No. CZ.02.1.01/0.0/0.0/17_049/0008440); European Union project LERCO (No. CZ.10.03.01/00/22_003/0000003) via the Operational Programme Just Transition; SALVAGE project CZ.02.01.01/00/22_008/0004644, supported by OP JAK, with co-financing from the EU and the State Budget; the Czech Health Research Council (NU23-03-00374); institutional support (MH CZ— DRO—FNOs/2022, MH CZ—DRO— FNOs/2023) from the Ministry of Health of the Czech Republic; the student’s grant system of the University of Ostrava (SGS10/LF/2022, SGS04/PrF/2023, SGS05/LF/2023, SGS11/LF/2023); Grant Agency of the Czech Republic (GACR 22-26981S) and the Ministry of Education, Youth and Sports of the Czech Republic through the e-INFRA CZ (ID:90254) and project “Podpora vědy a výzkumu v Moravskoslezském kraji 2021 a 2022.” Data for survival analysis with 1q21 amp/KRAS were obtained through Multiple Myeloma Research Foundation Personalized Medicine Initiatives (https://research.themmrf.org and www.themmrf.org).
Funding
Open access publishing supported by the National Technical Library in Prague.
Author information
Authors and Affiliations
Contributions
TJ, DZ, TS, and RH conceived and designed the study. TJ, DZ, TS, AAS, VK, OV, MS, SN and RH wrote the manuscript. VK, OV, and JV analyzed flow cytometry data. TS, VK, and HS prepared all sequencing libraries. DZ, AAS, DB,SN and GS designed and performed all bioinformatic analyses. LM, LB, TP, HP, VZ, PH, MH, ZCH, MH, and RH provided patients, study material, and clinical or preclinical data. All authors interpreted and discussed the results. All authors approved the final version of the manuscript prior to submission.
Corresponding author
Ethics declarations
Competing interests
The authors declare no competing interests.
Additional information
Publisher’s note Springer Nature remains neutral with regard to jurisdictional claims in published maps and institutional affiliations.
Supplementary information
Rights and permissions
Open Access This article is licensed under a Creative Commons Attribution 4.0 International License, which permits use, sharing, adaptation, distribution and reproduction in any medium or format, as long as you give appropriate credit to the original author(s) and the source, provide a link to the Creative Commons licence, and indicate if changes were made. The images or other third party material in this article are included in the article’s Creative Commons licence, unless indicated otherwise in a credit line to the material. If material is not included in the article’s Creative Commons licence and your intended use is not permitted by statutory regulation or exceeds the permitted use, you will need to obtain permission directly from the copyright holder. To view a copy of this licence, visit http://creativecommons.org/licenses/by/4.0/.
About this article
Cite this article
Jelinek, T., Zihala, D., Sevcikova, T. et al. Beyond the marrow: insights from comprehensive next-generation sequencing of extramedullary multiple myeloma tumors. Leukemia (2024). https://doi.org/10.1038/s41375-024-02206-w
Received:
Revised:
Accepted:
Published:
DOI: https://doi.org/10.1038/s41375-024-02206-w