« Prev Next »
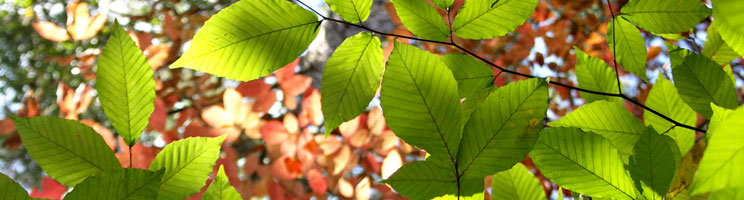
Evidence of climate change is all around us. From melting polar ice caps to home-range shifts of numerous plants and animals, the world is being altered at an alarming pace. The key factor believed to underlie climate change is increased temperature, resulting from increased levels of greenhouse gases in the atmosphere (i.e., the “greenhouse effect”; IPCC 2007; Figure 1). The greenhouse effect is a natural phenomenon permitting life to exist on our planet, however, increased levels of greenhouse gases intensify this effect resulting in increased global temperature. Over the past century, global temperature increased approximately 0.75ºC. At the current rate, global temperature may increase by 1.8–4.0ºC by the end of this century (IPCC 2007).
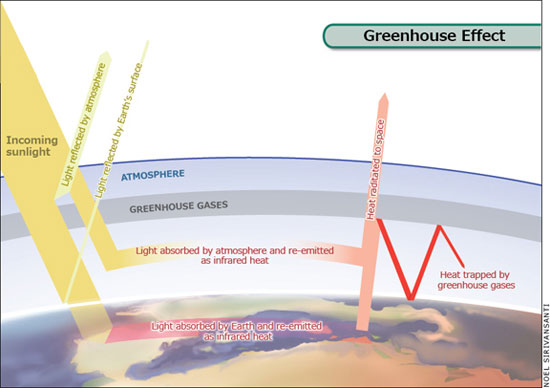
Greenhouse gases are naturally produced (Withgott & Brennan 2009; Bloom 2010). For example, volcanic activity generates large amounts of carbon dioxide (CO2). Limestone (calcium carbonate, CaCO3) and other sedimentary rocks store enormous amounts of carbon. When these rocks are broken down, such as by adverse weather conditions, CO2 is released into the atmosphere. Fires, which are increasing in frequency, also produce large amounts of greenhouse gases (e.g., CO2; methane, CH4).
Anthropogenic activities are also considerably increasing the levels of atmospheric contaminants. In the mid 1800s, with the advent of the industrial revolution, greenhouse gas levels started to increase dramatically (Withgott & Brennan, 2009; Figure 2). Burning of fossil fuels, such as coal, gasoline, and oil, produces both primary pollutants (those directly produced from fossil fuel combustion; e.g., nitrogen dioxide, NO2; carbon monoxide, CO; carbon dioxide, CO2) and secondary pollutants (compounds produced from fossil fuel combustion that undergo secondary chemical reactions to form pollutants; e.g., ozone, O3) (Withgott & Brennan 2009; Bloom 2010). Primary and secondary atmospheric pollutants are compounds that contribute to global climate change.
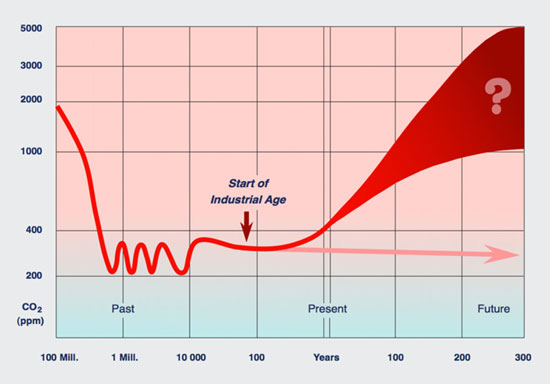
Key Greenhouse Gasses
Carbon Dioxide (CO2)
A direct result of fossil fuel combustion, CO2 is arguably the most important greenhouse gas, on the basis of both the amounts produced and its effects on the climate (Bloom 2010). The majority of CO2 produced by human activities stays in the atmosphere, while some also enters aquatic ecosystems. Carbon dioxide levels are approximately 380 ppm (parts per million), but are expected to reach 535–983 ppm by 2100 (IPCC 2007). (Approximate contribution to global warming: 33%; Hansen & Sato 2001).
Methane (CH4)
Ozone (O3)
Tropospheric ozone, as opposed to stratospheric ozone (i.e., the “ozone layer”), is a secondary pollutant. One of the main components of photochemical smog, it is generated through complex chemical reactions that involve sunlight, nitrogen oxides (NOx), carbon monoxide (CO), and volatile organic compounds (VOCs) (Bloom 2010). All of these compounds are produced through by fossil fuel combustion. Tropospheric ozone levels may increase by 40–60% by 2100 (IPCC, 2007). (Approximate contribution to global warming: 13%; Hansen & Sato 2001).
Chlorofluorocarbons (CFCs)
Molecules of carbon atoms bound to chlorine and fluorine atoms. These compounds are used in diverse ways such as refrigerants, spray can propellants, cleaners, and in the production of Styrofoam (Bloom 2010). These compounds break down the ozone layer; chlorine atoms can break down multiple ozone molecules (Withgott & Brennan 2009). While tremendous progress has been made to reduce the production of these compounds, they have a lifespan in the atmosphere of 20–100 years, and the potential to increase global warming 1000-times more than a similar mass of CO2 molecules (IPCC 2007; Bloom 2010). (Approximate contribution to global warming: 7%; Hansen & Sato, 2001).
Nitrous Oxide (N2O)
Most nitrous oxide is produced by microorganisms undergoing anaerobic respiration (denitrification). Large fertilizer additions, a common agricultural practice, increase these emissions (Bloom, 2010). Nitrous oxide levels are approximately 0.32 ppm, but future levels are predicted to be 0.36–0.46 ppm by 2100 (IPCC 2007). (Approximate contribution to global warming: 6%; Hoffman et al. 2006).
Effects of Greenhouse Gases Are Not Just from Global Warming
As plants are primary producers, and CO2 is converted into sugars through photosynthesis, CO2 is essential for plant growth. It would seem intuitive, then, that increased CO2 levels would benefit both the plants and the animals that utilize them. But how do animals respond to plants grown under increased CO2 levels? In general, increased CO2 levels decrease leaf nitrogen levels, a vital nutrient for herbivorous animals (Ehleringer et al. 2005). Similarly, plants frequently increase the production of secondary compounds (antifeedants, toxins) under elevated CO2 atmospheres (Ehleringer et al. 2005). As a result, herbivores may increase feeding rates on enriched plants to compensate for decreased nitrogen availability, but still exhibit decreased growth rates and higher mortality (Percy et al. 2002).
In comparison, the effects of tropospheric O3 may be even more detrimental to ecosystem functioning. Ozone, a highly unstable greenhouse gas, damages organisms at the cellular level (Menzel 1984). When ozone breaks down, free radicals are produced; free radicals directly damage both lipids and proteins (Halliwell & Gutteridge 1999). In plants, O3 directly interferes with photosynthesis; plants become weakened and susceptible to herbivory, plant pathogens, and diseases. It is not yet possible to generalize how animal populations will be influenced by elevated tropospheric O3 levels; both positive and negative effects have been reported (Percy et al. 2002).
Scientists have contemplated the long-term effects of increased atmospheric pollutants on animal populations. It is believed that atmospheric contaminants will alter animal populations by: 1. increasing extinction rates, and/or 2. reducing genetic diversity (Mondor et al. 2005). As organisms are faced with challenges at an unprecedented scale and rate, species extinctions may increase and become widespread as these organisms are unable to cope with these challenges. Others argue that most populations have sufficient genetic variation to survive almost any environmental changes. Species may persist, but with reduced genetic variance, they may become more susceptible to other environmental challenges (e.g., diseases).
Examples
Ocean Life
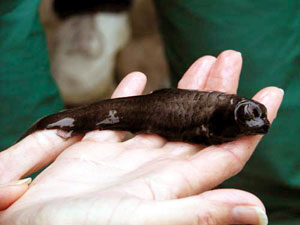
Insect Behavior
As greenhouse gases alter plant developmental trajectories, insects colonizing those plants may also differ behaviorally and physiologically. Research conducted at the Aspen Free-Air CO2 Enrichment (Aspen FACE) site on insects that colonize plants growing under increased CO2 and/or O3 levels shows some striking results. Poplar aphids (Chaitophorus stevensis) colonizing Trembling aspen (Populus tremuloides) trees grown under high CO2 conditions are behaviorally different than those colonizing trees grown under elevated O3 (Mondor et al. 2004; Figure 4). When exposed to alarm pheromone, aphids in high CO2 environments disperse at a lower rate, while those growing in high O3 environments disperse at a higher rate, from the plant than those living at ambient CO2 and O3 levels (Mondor et al. 2004). It is unknown whether this effect results from altered host plant quality or is a result of altered production/reception of alarm pheromone. This experiment does suggest, however, that behavioral responses to pheromones (such as are often used for pest management) may be altered under future atmospheric conditions.
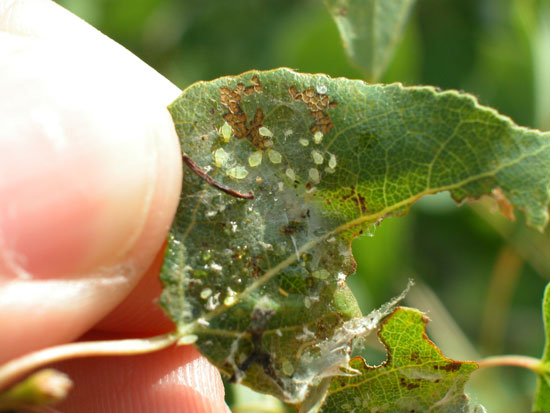
Bird Foraging
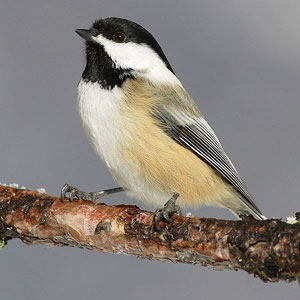
Summary
Animal populations face rapid and long-lasting challenges as a result of atmospheric changes. Some of these challenges will result from atmospherically induced increases in global temperature. Greenhouse gases such as CO2 and tropospheric O3, however, have already been documented, and will continue to alter animal population dynamics through altered ecosystem functioning. Organisms may express both behavioral and physiological changes to atmospheric composition, resulting in large-scale and long-term responses of animal populations ranging from reduced genetic variation to increased rates of extinction.
References and Recommended Reading
Bloom, A. J. Global Climate Change: Convergence of Disciplines. 1st ed. Sunderland, MA: Sinauer & Associates, 2010.
Brewer, P. G. & Peltzer, E. T. Limits to marine life. Science 324, 347-348 (2009).
Ehleringer, J. R., Cerling, T. E. et al. eds. A History of Atmospheric CO2 and its Effects on Plants, Animals, and Ecosystems. New York, NY: Springer, 2005.
Halliwell, B. & Gutteridge, J. M. C. Free Radicals in Biology and Medicine, 3rd ed. New York, NY: Oxford University Press 1999.
Hansen, J. E. & Sato, M. Trends of measured climate forcing agents. Proceedings of the National Academy of Sciences of the United States of America 98, 14778-14783 (2001).
Hoffman, D. J., Butler, J. H. et al., The role of carbon dioxide in climate forcing from 1979 to 2004: introduction of the Annual Greenhouse Gas Index. Tellus Series B - Chemical and Physical Meterology 58, 614-619 (2006).
Intergovernmental Panel on Climate Change (IPCC). Climate Change 2007: Synthesis Report. Geneva: IPCC Secretariat, 2007.
Menzel, D. B. Ozone: An overview of its toxicity in man and animals. Journal of Toxicology and Environmental Health, Part A: Current Issues 13, 181-204 (1984).
Mondor, E. B., Tremblay, M. N. et al., Divergent pheromone-mediated insect behaviour under global atmospheric change. Global Change Biology 10, 1820-1824 (2004).
Mondor, E. B., Tremblay, M. N. et al., Altered genotypic and phenotypic frequencies of aphid populations under enriched CO2 and O3 atmospheres. Global Change Biology 11, 1990-1996 (2005).
Muller, M., McWilliams, S. R. et al., Tri-trophic direct and indirect effects of plant defenses: Black-capped chickadees choose to eat gypsy moth caterpillars based on host leaf chemistry. Oikos 114, 507-517 (2006).
Percy, K. E., Awmack, C. S. et al., Altered performance of forest pests under atmospheres enriched by CO2 and O3. Nature 420, 403-407 (2002).
Withgott, J. H., & Brennan, S. Essential Environment: The Science Behind the Stories, 3rd ed. San Francisco, CA: Pearson, 2009.