« Prev Next »
To what extent do all living cells have the same structure? Do shared features only extend to a bounding membrane and DNA, RNA, and proteins as basic component parts of their molecular biology engines? If so, why is this the case? To answer such questions we first need to know how many types of cell structure there are. The planctomycete bacteria have challenged our assumptions about these fundamental questions.
The Three Domains of Life and the Concepts of Eukaryotes and Prokaryotes
What different kinds of cells do species in different kingdoms of life possess? All the same type or many different types or only a few? All contemporary living cells we know of are bounded by a membrane that surrounds the cell's cytoplasm and a genome of DNA. This applies to members of all three great Domains of life, the Bacteria, the Archaea, and the Eucarya (Woese, Kandler, & Wheelis 1990), first separated from each other on the basis of ribosomal RNA sequences (Woese & Fox 1977). The cells of Bacteria and Archaea, with a simple cell architecture, differ fundamentally in cell structure from those of Eucarya, which have more complex structure, with internal compartments defined by membranes. These two types have been termed prokaryotes (cells with naked DNA without a membrane envelope) and eukaryotes (cells with a membrane-bounded nucleus). Eukaryotes (meaning ‘true nuclei" where "karyote" is derived from the ancient Greek for "kernel") include fungi, protozoa, eukaryotic algae, and of course plants and animals including ourselves. Eukaryotic cells have a nucleus containing chromosomal DNA bounded by membranes. The cell types in Bacteria and Archaea are considered as "prokaryotes." The use of the term prokaryote was first introduced as a result of electron microscope studies showing a shared simple cell structure among bacteria (Stanier & Van Niel 1962). It has been subject to controversy since two distinct Domains of life, Bacteria and Archaea, are grouped as prokaryotes (Pace 2006; Whitman 2009, Martin & Koonin 2006; Cavalier-Smith 2007; Dolan & Margulis 2007; Glansdorff, Xu & Labedan 2008) . Interestingly, "prokaryote" means "before the nucleus," and refers to a cell structure where the chromosomal DNA is not enveloped in any internal membrane but is free in the cell cytoplasm.
It is a useful rule of thumb, for example for a scientist using a light microscope in a hospital microbiology lab, that eukaryotes such as the yeast Candida are larger than prokaryotes by an order of ten. However, cell size does not define eukaryotes, since eukaryotes and prokaryotes overlap in size. The marine alga Ostreococcus manages the internal complexity of chloroplasts, mitochondria, and nucleus in a cell about 1 μm long and 0.7 μm wide, similar to many Bacteria and Archaea (Courties, 1994). In contrast, one of the largest known bacteria — Epulopiscium fishelsoni found in fish intestines — can be up to 600 μm long and 80 μm wide, just visible to the naked eye (Angert, Clements & Pace 1993). The biggest bacterium of all is a giant sulfur-depositing bacterium Thiomargarita namibiensis, typically at least 100 μm wide and up to 750 μm wide (Schulz 1999). Genome sizes of prokaryotes and eukaryotes also overlap: only 2.9 Mb (million base pairs) long in the eukaryotic parasite Encephalitozoon cuniculi but 9.1 Mb in gliding bacterium Myxococcus xanthus.
So What Criteria Can Reliably Distinguish Any Eukaryote from Any Prokaryote?
In prokaryote cell organization there is a nucleoid containing genomic DNA but it is not surrounded by membranes such as what defines the eukaryote nucleus (Martin & Koonin 2006). Eukaryotes such as fungi, protozoa, plants, and animals, and thus we humans, have cells with complex structure (Figure 1) with internal membranes and membrane-bounded organelles. These include the chromosome-containing nucleus surrounded by a nuclear envelope consisting of two membranes. Most eukaryotes also have further membrane-bounded organelles, mitochondria and chloroplasts. Some eukaryotes possess neither mitochondria or chloroplasts; these include parasitic protozoa causing human disease such as Giardia, ancestors of which are thought to have lost their mitochondria but retained remnant mitochondrial genes or even in some cases remnants of mitochondria called mitosomes or hydrogenosomes (Embley 2006). Mitochondria and chloroplasts represent ancient symbiotic events in which bacteria were acquired by early eukaryotes or their ancestors; molecular evidence for their relationships to bacteria is very strong.


Would you expect some features of eukaryote molecular biology to be characteristic of this type of cell? Yes, for example transcription and translation are not coupled in eukaryotes but are separated; protein-coding genes come as exons interrupted by introns which must be spliced out; DNA is compacted via proteins called histones; and there is a complex endomembrane system. That system includes membrane vesicle-dependent movement of materials into and out of the cell-endocytosis and exocytosis. Cell division in eukaryotes is a visibly complex multi-stage process called mitosis involving microtubules composed of cytoskeletal proteins.
Planctomycetes: Distinctive Bacteria with Compartmented Cells
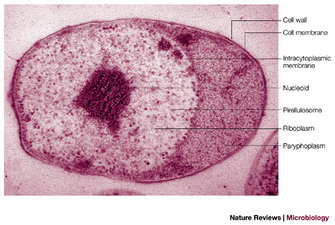



Membranes of the nuclear body envelope are formed during budding (Lee, Webb & Fuerst 2009). In the anammox bacteria mentioned above, there is also an additional but this time single-membrane-bounded organelle, the anammoxosome (), containing at least one enzyme important for their unique anammox ammonia-oxidizing physiology in the absence of oxygen (van Niftrik et al 2004). Another interesting feature of planctomycete cells is their condensed nucleoid, a mass of folded chromosomal DNA fibrils visible in sectioned cells via electron microscopy, contrasting with the nucleoids of other bacteria which tend to be spread throughout the cytoplasm. In the case of Gemmata obscuriglobus this has been linked to the high resistance of cells in this species to UV and gamma radiation (Lieber et al 2009). The planctomycetes are thus Bacteria that form a significant exception to simple prokaryote structure. Are there possibly other exceptions to be discovered? Perhaps; Ignicoccus hospitalis, an Archaean living at 90°C may pose similar problems for the prokaryote idea (Kuper, U. et al 2010).
Planctomycetes Are Important for Theories of the Origin of Eukaryotes and Their Nuclei
Why are planctomycetes important for understanding evolution of eukaryotes? There are two major types of hypothesis about how eukaryotes and their nuclei may have originated (Poole & Penny 2007). One of these involves fusion between cells from domains Archaea and Bacteria: one of the cells involved in the fusion becomes the eukaryote nucleus (Martin & Muller 1998; Martin 2005; Martin et al. 2001). There are several different versions of this hypothesis (Martin & Koonin 2006). The other type of hypothesis involves "autogenous" formation of internal membranes from only one non-eukaryote cell type via an infolding of cytoplasmic membrane to form an endomembrane system and eventually to form a nucleus (Cavalier-Smith 2002; Cavalier-Smith 2009). Nutrient acquisition may have selected for this (de Duve 2007).
The existence of planctomycetes with their membrane compartments supports an autogenous (self generating) model for formation of membrane-bounded nuclei (Glansdorff, Xu & Labedan 2008; Fuerst 2005). We don't yet know to what extent compartments of planctomycetes can be explained by molecular mechanisms similar to those of eukaryotes. Even if it turns out that there are no direct links between planctomycetes and eukaryotes (i.e. if convergent evolution can explain their similarities), the planctomycete cell plan implies that fusion models for origins of eukaryote-like cell compartments may not be needed to explain how eukaryotes acquired their nucleus.
Planctomycetes May Yield New Insights into the Eukaryote-Like Cell Biology of a Bacterium
Planctomycete cell structure differs from that of all other known prokaryotes. We are not sure yet whether the cell plan of a planctomycete like Gemmata resembles that of eukaryotes at a deeper molecular level. Such a eukaryote-like cell plan occurring in a member of the Bacteria suggests that it may be worth looking for such deeper similarities.
An interesting recent development suggesting that planctomycetes and their cells may possess deeper resemblances with eukaryotes is the discovery of an endocytosis-like process for protein uptake by cells of Gemmata obscuriglobus involving membrane infolding and vesicle formation (Lonhienne et al 2010). Like the membrane-bounded nuclear body in this species, such a process is unknown in other Bacteria or Archaea. This is consistent with the nutrient-acquisition-driven model for endomembrane evolution referred to above as a possible first step on the road to eukaryotes. Could planctomycetes have a whole series of further characteristics previously known only in eukaryotes, including eukaryote-homologous genes and proteins? Cell biologists and microbiologists don't know yet, but are excited by this prospect which may lead to new insights into the evolutionary relationships between simpler and more complex kinds of cells.
Summary
Cell biologists and microbiologists have in the past thought that only two major types of structural cell organization exist, prokaryotic — where the chromosomal DNA is naked and exposed within the cell cytoplasm — and eukaryotic — where chromosomal DNA is surrounded by a membranous envelope. All members of domains Bacteria and Archaea have been thought to possess prokaryotic organization. The planctomycete bacteria however complicate this simple scheme, since their cells are compartmentalized by internal membranes including those surrounding the chromosomal DNA. Are they a third type of cell organization that originated independently of other known types, or are they key to understanding how eukaryotes and their complex internal structure evolved? In either case they are significant for understanding the evolution of cells and the diverse ways in which cells can potentially evolve.
References and Recommended Reading
Angert, E. R., Clements, K. D. & Pace, N. R. The largest bacterium. Nature 362, 239–241 (1993) doi:10.1038/362239a0.
Cavalier-Smith, T. Concept of a bacterium still valid in prokaryote debate. Nature 446, 257 (2007) doi:10.1038/446257c.
Cavalier-Smith, T. The phagotrophic origin of eukaryotes and phylogenetic classification of Protozoa. Int J Syst Evol Microbiol 52, 297–354 (2002).
Cavalier-Smith, T. Predation and eukaryote cell origins: a coevolutionary perspective. Int J Biochem Cell Biol 41, 307–322 (2009) doi:10.1016/j.biocel.2008.10.002.
Courties, C. et al. Smallest eukaryotic organism. Nature 370, 255 (1994).
de Duve, C. The origin of eukaryotes: a reappraisal. Nat Rev Microbiol 8, 395–403 (2007) doi:10.1038/nrg2071.
Dolan, M. F. & Margulis, L. Advances in biology reveal truth about prokaryotes. Nature 445, 21 (2007) doi:10.1038/445021b.
Embley, T. M. Multiple secondary origins of the anaerobic lifestyle in eukaryotes. Philos Trans R Soc Lond B Biol Sci 361, 1055–1067 (2006) doi:10.1098/rstb.2006.1844.
Fuerst, J. A. Intracellular compartmentation in planctomycetes. Annu Rev Microbiol 59, 299–328 (2005) doi:10.1146/annurev.micro.59.030804.121258.
Fuerst, J. A. & Webb, R. I. Membrane-bounded nucleoid in the eubacterium Gemmata obscuriglobus. Proc Natl Acad Sci U S A 88, 8184–8188 (1991).
Fuerst, J. A. The planctomycetes: emerging models for microbial ecology, evolution and cell biology. Microbiology 141 ( Pt 7), 1493–1506 (1995).
Glansdorff, N., Xu, Y. & Labedan, B. The last universal common ancestor: emergence, constitution and genetic legacy of an elusive forerunner. Biol Direct 3, 29 (2008) doi:10.1186/1745-6150-3-29.
Kartal, B., Kuenen, J. G. & van Loosdrecht, M. C. Engineering. Sewage treatment with anammox. Science 328, 702–703 (2010) doi:10.1126/science.1185941.
Kuenen, J. G. Anammox bacteria: from discovery to application. Nat Rev Microbiol 6, 320–326 (2008) doi:10.1038/nrmicro1857.
Kuper, U. et al. Energized outer membrane and spatial separation of metabolic processes in the hyperthermophilic Archaeon Ignicoccus hospitalis. Proc Natl Acad Sci USA 107, 3152–3156 (2010) doi:10.1073/pnas.0911711107.
Lindsay, M. R. et al. Cell compartmentalisation in planctomycetes: novel types of structural organisation for the bacterial cell. Arch Microbiol 175, 413–429 (2001).
Lonhienne, T. G. et al. Endocytosis-like protein uptake in the bacterium Gemmata obscuriglobus. Proc Natl Acad Sci USA (2010) doi:10.1073/pnas.1001085107.
Lee, K. C., Webb, R. I. & Fuerst, J. A. The cell cycle of the planctomycete Gemmata obscuriglobus with respect to cell compartmentalization. BMC Cell Biol 10, 4 (2009) doi:10.1186/1471-2121-10-4.
Lieber, A., Leis, A., Kushmaro, A., Minsky, A. & Medalia, O. Chromatin organization and radio resistance in the bacterium Gemmata obscuriglobus. J Bacteriol, 191 1439–1445 (2009) doi:10.1128/jb.01513-08.
Lindsay, M. R., Webb, R. I. & Fuerst, J. A. Pirellulosomes: A new type of membrane-bounded cell compartment in planctomycete bacteria of the genus Pirellula. Microbiol-Uk 143, 739–748 (1997).
Martin, W. Archaebacteria (Archaea) and the origin of the eukaryotic nucleus. Curr Opin Microbiol 8, 630–637 (2005) doi:10.1016/j.mib.2005.10.004.
Martin, W. et al. An overview of endosymbiotic models for the origins of eukaryotes, their ATP-producing organelles (mitochondria and hydrogenosomes), and their heterotrophic lifestyle. Biol Chem 382, 1521–1539 (2001) doi:10.1515/bc.2001.187.
Martin, W. & Koonin, E. V. A positive definition of prokaryotes. Nature 442, 868 (2006) doi:10.1038/442868c.
Martin, W. & Koonin, E. V. Introns and the origin of nucleus-cytosol compartmentalization. Nature 440, 41–45 (2006) doi:10.1038/nature04531.
Martin, W. & Muller, M. The hydrogen hypothesis for the first eukaryote. Nature 392, 37–41 (1998) doi:10.1038/32096.
Pace, N. R. Time for a change. Nature 441, 289 (2006) doi:10.1038/441289a.
Pace, N. R. Problems with "procaryote". J Bacteriol 191, 2008–2010; discussion 2011 (2009) doi:10.1128/jb.01224-08
Papineau, D. et. al Composition and structure of microbial communities from stromatolites of Hamelin Pool in Shark Bay, Western Australia. Appl Environ Microbiol 71, 4822–4832 (2005) doi:10.1128/aem.71.8.4822-4832.2005
Poole, A. M. & Penny, D. Evaluating hypotheses for the origin of eukaryotes. Bioessays 29, 74–84 (2007) doi:10.1002/bies.20516.
Schlesner, H. The development of media suitable for the microorganisms morphologically resembling Planctomyces spp., Pirellula spp., and other Planctomycetales from various aquatic habitats using dilute media. Systematic and Applied Microbiology 17, 135–145 (1994).
Schulz, H. N. et al. Dense populations of a giant sulfur bacterium in Namibian shelf sediments. Science 284, 493–495 (1999).
Stanier, R. Y. & Van Niel, C. B. The concept of a bacterium. Arch Mikrobiol 42, 17–35 (1962).
Strous, M. et al. Missing lithotroph identified as new planctomycete. Nature 400, 446–449 (1999) doi:10.1038/22749.
van Niftrik, L. A. et al. The anammoxosome: an intracytoplasmic compartment in anammox bacteria. FEMS Microbiol Lett 233, 7–13 (2004).
Whitman, W. B. The modern concept of the procaryote. J Bacteriol 191, 2000–2005; discussion 2006–2007 (2009) doi:10.1128/jb.00962-08.
Woese, C. R., Kandler, O. & Wheelis, M. L. Towards a natural system of organisms: proposal for the domains Archaea, Bacteria, and Eucarya. Proc Natl Acad Sci U S A 87, 4576–4579 (1990).
Woese, C. R. & Fox, G. E. Phylogenetic structure of the prokaryotic domain: the primary kingdoms. Proc Natl Acad Sci U S A 74, 5088–5090 (1977).