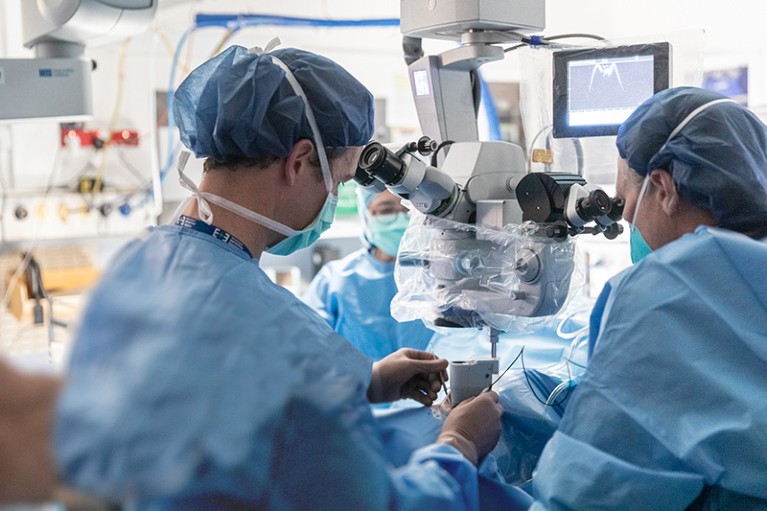
Tom Edwards (left), from the Centre for Eye Research Australia, administers a viral-vector-based gene therapy.Credit: Mathew Lynn/CERA
In a landmark 1972 paper1, physician Theodore Friedmann and biochemist Richard Roblin foresaw a future in which DNA could be manipulated to help treat human genetic diseases. But they cautioned that it should remain off-limits until the field gained a firmer grasp of genetic processes in cells, their relationship to disease and the potential side effects of treatments.
Over the following years, scientists began to remove these obstacles, and in 1990 achieved a major breakthrough when a four-year-old girl with a form of severe combined immunodeficiency disease was successfully treated in a clinical trial2. This ushered in a decade of high hopes and bold promises.
Nature Index 2022 Biomedical sciences
But the afterglow of that early achievement proved short-lived, as a labyrinth of technical challenges emerged. A common gene-therapy approach involves delivering a healthy gene to cells that have only defective copies in their genetic libraries. Once inside, the therapeutic gene instructs the cells to manufacture functional proteins instead of faulty ones. One difficulty has been making sure a treatment gene zeroes in on the correct cells in the right tissue, and is shuttled into millions of these cells rather than just a few, all without disrupting the working order of neighbouring genes. Another impediment has been ensuring that the inserted gene produces enough protein to get the job done, says John Rasko, a clinical haematologist who heads the Department of Cell and Molecular Therapies at the Royal Prince Alfred Hospital in Sydney, Australia. “We had the tools that could deliver genes into cells, but we just couldn’t do it efficiently enough to get a therapeutic benefit,” he says.
And the therapeutic gene also needed to ride in a delivery vehicle that could slip quietly into cells without triggering a harmful, and sometimes fatal, reaction from the toughest gatekeeper of them all: the immune system.
Despite these challenges, scientists made further progress, but in 1999, an 18-year-old trial participant died of liver failure shortly after receiving a dose of an experimental gene therapy for ornithine transcarbamylase (OTC) deficiency, a rare metabolic disorder that prevents the body from breaking down ammonia. The tragedy triggered a shockwave through the field and forced it to reassess. “Absolutely there was a ‘dark age’ in gene therapy” after this point, says Rasko. However, more promising advances are finally beginning to emerge.
Viruses as delivery drivers
Through the highs and lows, viruses have remained the couriers of choice for delivering therapeutic genes to hard-to-reach cells. In nature, viruses are cunning cellular hijackers that can ignite devastating disease outbreaks and pandemics. Their ability to penetrate cells and hoodwink the host into replicating their genes also makes them the perfect tool for gene therapy. With millions of years of evolution behind them, viruses have a formidable edge over other gene-therapy vectors, such as synthetic nanoparticles, says Julie Crudele, who investigates viral-vector gene therapies for muscular dystrophies at the University of Washington in Seattle. “They’re already designed to do this,” she says. “We just hijack what they do naturally.”
Over the past decade, efforts to vanquish the spectre of failures past have started to pay off, heralding an era of optimism, particularly in the treatment of genetic eye disorders. There are almost 300 clinical trials worldwide investigating viral-vector-based gene therapies. Roughly half of these use modified adeno-associated viruses (AAVs) — small, well-studied viruses that do not cause disease in humans. These viruses are an ideal match for gene therapy as they can target a wide range of tissues and trigger only a mild immune response in most cases, making them safe, versatile and efficient therapeutic tools.
Unveiling clearer vision
One of the recent jewels in the gene-therapy crown has been in inherited retinal diseases, which cause vision loss and blindness in more than two million people worldwide. “There’s been a huge explosion in gene therapy and the eye,” says Christine Kay, a surgeon at Vitreoretinal Associates in Gainesville, Florida. Eyes are immune-privileged, which means they limit local immune and inflammatory responses to preserve vision. This makes them an ideal gene-therapy target because they are shielded from the destructive force of immune-induced inflammation and can sneak in without setting off alarm bells (see ‘Viral vectors’).
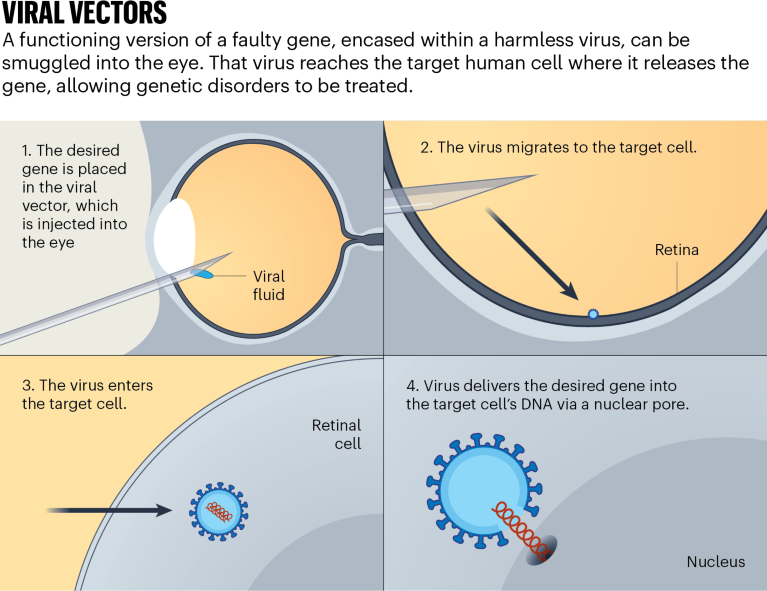
In 2017, voretigene neparvovec-rzyl (marketed as Luxturna) was the first gene replacement therapy to be approved by the US Food and Drug Administration (FDA). It was one of the first treatments for an inherited retinal disease. Developed by Spark Therapeutics, a biotechnology start-up in Philadelphia, Pennsylvania, the gene therapy is a one-shot treatment for people with a harmful mutation in the gene RPE65, which causes severe vision loss. The drug relies on an AAV vector to deliver a working copy of the gene directly into retinal cells, where it gives instructions for making a protein that converts light into electrical signals. The treatment has also been approved in the European Union and in Australia. “That’s been a boon to the field,” says Thomas Edwards, who leads the retinal gene-therapy research group at the Centre for Eye Research Australia in Melbourne.
Investors and big pharma are catching on. In the two years before 2020, seven pharmaceutical giants — including Novartis, Roche and Bayer — acquired small biotech companies developing viral-vector-based gene therapies at values near or above US$1 billion. Globally, the gene-therapy market’s revenue is projected to jump from $5.33 billion to $19.88 billion by 2027. Positive results from clinical trials in recent years have given patients and researchers a much-needed confidence boost, says Guangping Gao, a gene-therapy researcher specializing in viral vectors at the University of Massachusetts Chan Medical School in Worcester. “That confidence eventually translates into more investment.”
The Luxturna milestone laid the groundwork for tackling more-common eye disorders underpinned by multiple genes, says Edwards. “Inherited retinal diseases have sort of been the starting point, because they lend themselves to a gene-therapy approach,” he says. “We’re now seeing the technology being applied to more common diseases, which are more genetically complicated.”
One of those diseases is age-related macular degeneration, a leading cause of vision loss globally. GT005, an AAV-based gene therapy developed by London-based Gyroscope Therapeutics, is designed to target dry age-related macular degeneration. GT005’s genetic payload aims to restore balance by boosting the production of complement factor I (CFI), a protein that usually regulates the complement system — a facet of the body’s immune arsenal that drives age-related macular degeneration when it becomes overactive. Turning up the production of CFI reduces the inflammation associated with the overactive complement system, reducing the number of vision-destroying blood vessels in the eye. Phase I/II trials are under way in the United States, Europe and Australia. If proven to be safe and effective, the AAV-based gene therapy could offer hope to patients with the once untreatable disease, says Edwards, who is leading the Australian trials.
The eyes and beyond
Progress is also being made outside the eye, although challenges remain ahead. In 2019, the FDA approved onasemnogene abeparvovec (marketed as Zolgensma), an AAV-based gene therapy for spinal muscular atrophy. Developed by Novartis Gene Therapies, the treatment — which costs $2 million per dose — replaces the disease-causing gene with a healthy version in patients younger than two years old. More than 2,300 patients have been treated with Zolgensma to date. But in August, two children in Russia and Kazakhstan died of acute liver failure about one month after receiving a dose, despite being treated with medication to reduce the risk of side effects. In a statement released after the deaths, Novartis said that acute liver failure “is a known side effect” of Zolgensma, but added that it will update its labelling to reflect that fatalities had been reported. It also said it firmly believed in the drug’s “overall favourable risk/ benefit profile”.
Spinal muscular atrophy isn’t the only neuromuscular disease on the gene-therapy hit-list. With five trials in the United States alone, Duchenne muscular dystrophy is also being targeted for treatment. In 2019, Solid Biosciences, a life-sciences company in Charlestown, Massachusetts, paused its phase I and II trials for SGT-001 gene therapy after several participants experienced adverse side effects, including liver damage and low platelet counts. But after improving the manufacturing process, the company reported in March that three patients receiving high doses showed improvements in their motor and pulmonary function without the harmful side effects seen previously.
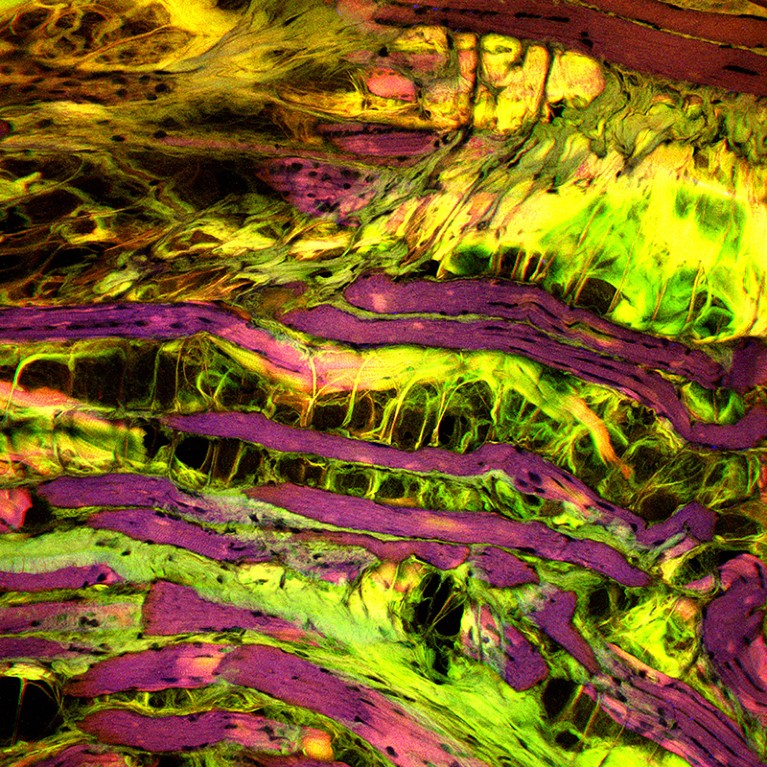
Micrograph images of a section through muscle tissue affected by muscular dystrophy.Credit: Patrick Landmann/SPL
Treating muscular diseases with AAV-based gene therapy is a fine balance; massive doses are needed to make a therapeutic impact, which increases the chance of adverse reactions, says Crudele. “You truly have to hit every single cell, which means that you have to give these really huge doses.”
Some researchers are tackling this problem by tweaking AAV vectors to deliver working genes to cells more efficiently, reducing the need for large doses. In 2021, researchers genetically altered the AAV vectors themselves3. When they injected each vector into mice with Duchenne muscular dystrophy, they found that one of the modified vectors reached muscle tissue ten times more efficiently than the standard ones, remaining effective even at low doses.
The end of the dark ages?
Haemophilia — an inherited blood-clotting disorder that affects more than one million people worldwide — has been edging its way to the top of the gene-therapy queue for decades. It is usually treated with regular injections to replace the missing clotting protein. But high costs prevent patients in developing countries, where roughly 80% of haemophilia cases are found, from accessing the treatment. “The majority will die young because of the fact that they can’t access treatment,” says Amit Nathwani, who specializes in gene therapies for haemophilia and other blood disorders at University College London. “For these individuals, gene therapy will be a game-changer.” There are currently at least 12 clinical trials exploring AAV-based gene therapies for haemophilia A and B.
Nathwani and his team at biotech firm Freeline Therapeutics in Stevenage, UK, are leading trials in the United States and Europe on a haemophilia B gene therapy called FLT180a (verbrinacogene setparvovec). The treatment delivers a functional version of the affected gene inside a synthetic AAV vector. In a 2022 paper4, 90% of trial participants’ clotting protein levels remained high two years after receiving a dose, meaning that they no longer needed regular protein injections. “If you had asked 10 or 12 years ago if we would ever achieve a normal range in haemophilia B patients, I would have said no,” says Nathwani. “We’ve been able to push the frontier.”
With emerging treatments such as FLT180a in the pipeline, the future is beginning to look better for people with debilitating genetic diseases, from blood disorders to eye conditions. And although gene therapy still has a way to go before it becomes a routine treatment, the wave of approvals and clinical trials signal that the dark age of gene therapy could finally be over, says Rasko. “It’s here to stay.”