- OUTLOOK
Decoding the neuroscience of consciousness
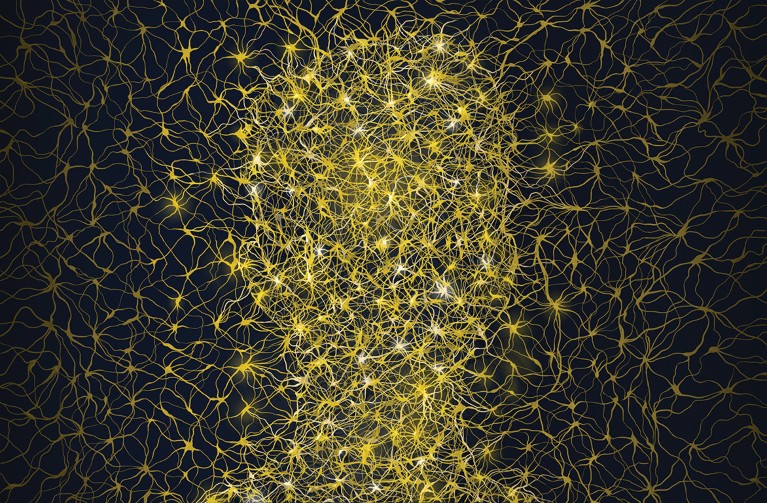
Credit: Sam Falconer
Access options
Access Nature and 54 other Nature Portfolio journals
Get Nature+, our best-value online-access subscription
$29.99 / 30 days
cancel any time
Subscribe to this journal
Receive 51 print issues and online access
$199.00 per year
only $3.90 per issue
Rent or buy this article
Prices vary by article type
from$1.95
to$39.95
Prices may be subject to local taxes which are calculated during checkout
Nature 571, S2-S5 (2019)
doi: https://doi.org/10.1038/d41586-019-02207-1
This article is part of Nature Outlook: The brain, an editorially independent supplement produced with the financial support of third parties. About this content.
References
Owen, A. M. et al. Science 313, 1402 (2006).
Monti, M. M. et al. N. Engl. J. Med. 362, 579–589 (2010).
Casali, A. G. et al. Sci. Transl. Med. 5, 198ra105 (2013).
Siclari, F. et al. Nature Neurosci. 20, 872–878 (2017).
Demertzi, A. et al. Sci. Adv. 5, eaat7603 (2019).
Monti, M. M., Schnakers, C., Korb, A. S., Bystritsky, A. & Vespa, P. M. Brain Stimul. 9, 940–941 (2016).
Taschereau-Dumouchel, V. et al. Proc. Natl Acad. Sci. USA 115, 3470–3475 (2018).
Michel, M. et al. Front. Psychol. 9, 2134 (2018).
Michel, M. et al. Nature Hum. Behav. 3, 104–107 (2019).