- OUTLOOK
Unlocking the secrets of scar-free skin healing
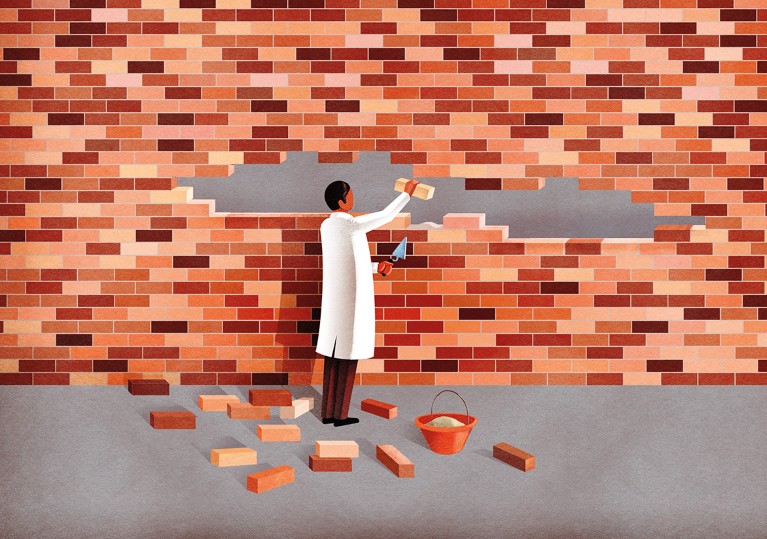
Credit: Marco Melgrati
Access options
Access Nature and 54 other Nature Portfolio journals
Get Nature+, our best-value online-access subscription
$29.99 / 30 days
cancel any time
Subscribe to this journal
Receive 51 print issues and online access
$199.00 per year
only $3.90 per issue
Rent or buy this article
Prices vary by article type
from$1.95
to$39.95
Prices may be subject to local taxes which are calculated during checkout
Nature 563, S86-S88 (2018)
doi: https://doi.org/10.1038/d41586-018-07430-w
This article is part of Nature Outlook: Skin, an editorially independent supplement produced with the financial support of third parties. About this content.
References
Nishiguchi, M. A., Spencer, C. A., Leung, D. H. & Leung, T. H. Cell Rep. 24, 3383–3392 (2018).
Rinkevich, Y. et al. Science 348, aaa2151 (2015).
Ito, M. et al. Nature 447, 316–320 (2007).
Plikus, M. V. et al. Science 355, 748–752 (2017).
Seifert, A. W. et al. Nature 489, 561–565 (2012).
Simkin, J., Gawriluk, T. R., Gensel, J. C. & Seifert, A. W. eLife 6, e24623 (2017).
Holmes, J. H. IV et al. J. Burn Care Res. 39, 694–702 (2018).
Centanni, J. M. et al. Ann. Surg. 253, 672–683 (2011).