Abstract
Nearly a century after Einstein first predicted the existence of gravitational waves, a global network of Earth-based gravitational wave observatories1,2,3,4 is seeking to directly detect this faint radiation using precision laser interferometry. Photon shot noise, due to the quantum nature of light, imposes a fundamental limit on the attometre-level sensitivity of the kilometre-scale Michelson interferometers deployed for this task. Here, we inject squeezed states to improve the performance of one of the detectors of the Laser Interferometer Gravitational-Wave Observatory (LIGO) beyond the quantum noise limit, most notably in the frequency region down to 150 Hz, critically important for several astrophysical sources, with no deterioration of performance observed at any frequency. With the injection of squeezed states, this LIGO detector demonstrated the best broadband sensitivity to gravitational waves ever achieved, with important implications for observing the gravitational-wave Universe with unprecedented sensitivity.
This is a preview of subscription content, access via your institution
Access options
Subscribe to this journal
Receive 12 print issues and online access
$209.00 per year
only $17.42 per issue
Prices may be subject to local taxes which are calculated during checkout



Similar content being viewed by others
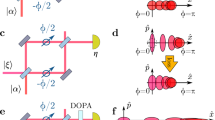
Overcoming detection loss and noise in squeezing-based optical sensing
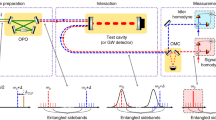
Generation and control of frequency-dependent squeezing via Einstein–Podolsky–Rosen entanglement
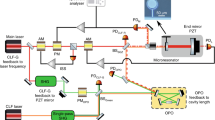
Broadband reduction of quantum radiation pressure noise via squeezed light injection
References
Abramovici, A. et al. LIGO: the Laser Interferometer Gravitational-Wave Observatory. Science 256, 325–333 (1992).
Abbott, B. et al. LIGO: the Laser Interferometer Gravitational-Wave Observatory. Rep. Prog. Phys. 72, 076901 (2009).
Acernese, F. et al. Virgo status. Classical Quant. Grav. 25, 184001 (2008).
Grote, H. et al. The GEO 600 Status. Classical Quant. Grav. 27, 084003 (2010).
Caves, C. M. Quantum-mechanical radiation-pressure fluctuations in an interferometer. Phys. Rev. Lett. 45, 75–79 (1980).
Caves, C. M. Quantum-mechanical noise in an interferometer. Phys. Rev. D 23, 1693–1708 (1981).
Gerry, C. & Knight, P. Introductory Quantum Optics 10–18 (Cambridge Univ. Press, 2005).
Slusher, R. E. et al. Observation of squeezed states generated by four-wave mixing in an optical cavity. Phys. Rev. Lett. 55, 2409–2412 (1985).
Xiao, M., Wu, L. A. & Kimble, H. J. Precision measurement beyond the shot-noise limit. Phys. Rev. Lett. 59, 278–281 (1987).
McKenzie, K. et al. Squeezing in the audio gravitational wave detection band. Phys. Rev. Lett. 93, 161105 (2004).
Vahlbruch, H. et al. Coherent control of vacuum squeezing in the gravitational-wave detection band. Phys. Rev. Lett. 97, 011101 (2006).
Vahlbruch, H., Chelkowski, S., Danzmann, K. & Schnabel, R. Quantum engineering of squeezed states for quantum communication and metrology. New J. Phys. 9, 371 (2007).
Goda, K. et al. A quantum-enhanced prototype gravitational-wave detector. Nature Phys. 4, 472–476 (2008).
Abadie, J. et al. A gravitational wave observatory operating beyond the quantum shot-noise limit. Nature Phys. 7, 962–965 (2011).
Harry, G. M. et al. Advanced LIGO: the next generation of gravitational wave detectors. Classical Quant. Grav. 27, 084006 (2010).
Fricke, T. et al. DC readout experiment in enhanced LIGO. Classical Quant. Grav. 29, 065005 (2012).
Chua, S. et al. Backscatter tolerant squeezed light source for advanced gravitational-wave detectors. Opt. Lett. 36, 4680–4682 (2011).
Stefszky, M. S. et al. Balanced homodyne detection of optical quantum states at audio-band frequencies and below. Classical Quant. Grav. 29, 145015 (2012).
Abadie, J. et al. Search for gravitational waves from low mass compact binary coalescence in LIGO's sixth science run and Virgo's science runs 2 and 3. Phys. Rev. D 85, 082002 (2012).
Evans, M., Barsotti, L. & Fritschel, P. A general approach to optomechanical parametric instabilities. Phys. Lett. A 374, 665–671 (2009).
Takeno, Y. et al. Observation of –9 dB quadrature squeezing with improvement of phase stability in homodyne measurement. Opt. Express 15, 4321–4327 (2007).
Franzen, A., Hage, B., DiGuglielmo, J., Fiurášek, J. & Schnabel, R. Experimental demonstration of continuous variable purification of squeezed states. Phys. Rev. Lett. 97, 150505 (2006).
Yonezawa, H. et al. Quantum-enhanced optical phase tracking. Science 337, 1514–1517 (2012).
Schnabel, R., Mavalvala, N., McClelland, D. E. & Lam, P. K. Quantum metrology for gravitational wave astronomy. Nature Commun. 1, 121 (2010).
McClelland, D. E., Mavalvala, N., Chen, Y. & Schnabel, R. Advanced interferometry, quantum optics and optomechanics in gravitational wave detectors. Laser Photon. Rev. 5, 677–696 (2011).
Kimble, H. J., Levin, Y., Matsko, A. B., Thorne, K. S. & Vyatchanin, S. P. Conversion of conventional gravitational-wave interferometers into quantum nondemolition interferometers by modifying their input and/or output optics. Phys. Rev. D 65, 022002 (2001).
Abbott, B. P. et al. Searches for gravitational waves from known pulsars with science run 5 LIGO data. Astrophys. J. 713, 671–685 (2010).
Hinderer, T., Lackey, B. D., Lang, R. N. & Read, J. S. Tidal deformability of neutron stars with realistic equations of state and their gravitational wave signatures in binary inspiral. Phys. Rev. D 81, 123016 (2010).
Vines, J. & Flanagan, E. E. Post-1-Newtonian tidal effects in the gravitational waveform from binary inspirals. Phys. Rev. D 83, 084051 (2011).
Bauswein, A. & Janka, H. T. Measuring neutron-star properties via gravitational waves from neutron-star mergers. Phys. Rev. Lett. 108, 011101 (2012).
Abbott, B. et al. Observation of a kilogram-scale oscillator near its quantum ground state. New J. Phys. 11, 073032 (2009).
Acknowledgements
The authors acknowledge support from the United States National Science Foundation for the construction and operation of the LIGO Laboratory, and the Science and Technology Facilities Council of the United Kingdom, the Max-Planck-Society and the State of Niedersachsen/Germany for supporting the construction and operation of the GEO600 detector. The authors also acknowledge support for the research, by these agencies and by the Australian Research Council, the International Science Linkages programme of the Commonwealth of Australia, the Council of Scientific and Industrial Research of India, the Istituto Nazionale di Fisica Nucleare of Italy, the Spanish Ministerio de Economía y Competitividad, the Conselleria d'Economia, Hisenda i Innovació of the Govern de les Illes Balears, the Royal Society, the Scottish Funding Council, the Scottish Universities Physics Alliance, The National Aeronautics and Space Administration, the National Research Foundation of Korea, Industry Canada and the Province of Ontario through the Ministry of Economic Development and Innovation, the National Science and Engineering Research Council Canada, the Carnegie Trust, the Leverhulme Trust, the David and Lucile Packard Foundation, the Research Corporation and the Alfred P. Sloan Foundation.
Author information
West Virginia University, Morgantown, West Virginia 26505, USA
Authors and Affiliations
LIGO – California Institute of Technology, Pasadena, 91125, California, USA
J. Aasi, J. Abadie, B. P. Abbott, R. Abbott, M. R. Abernathy, R. X. Adhikari, P. Ajith, S. B. Anderson, K. Arai, M. C. Araya, L. Austin, J. C. Barayoga, G. Billingsley, E. Black, J. K. Blackburn, R. Bork, A. F. Brooks, K. Buckland, C. Cepeda, T. Chalermsongsak, D. C. Coyne, B. Daudert, V. Dergachev, S. Doravari, J. C. Driggers, P. Ehrens, R. Engel, T. Etzel, N. Fotopoulos, E. K. Gustafson, J. Harms, J. Heefner, A. W. Heptonstall, K. A. Hodge, A. Ivanov, M. Jacobson, E. James, P. Kalmus, W. Kells, P. J. King, V. Kondrashov, W. Z. Korth, D. Kozak, A. Lazzarini, V. Litvine, M. Mageswaran, K. Mailand, E. Maros, D. Martinov, J. N. Marx, G. McIntyre, S. Meshkov, T. Nash, G. H. Ogin, C. Osthelder, M. Pedraza, M. Phelps, C. Poux, L. R. Price, S. Privitera, E. Quintero, D. H. Reitze, N. A. Robertson, J. G. Rollins, V. Sannibale, L. Santamaría, F. Seifert, Z. Shao, A. Singer, L. Singer, M. R. Smith, N. D. Smith-Lefebvre, R. Taylor, E. Thrane, C. I. Torrie, S. Vass, L. Wallace, A. J. Weinstein, S. E. Whitcomb, P. A. Willems, R. Williams, T. Williams, H. Yamamoto, D. Yeaton-Massey, L. Zhang & J. Zweizig
SUPA, University of Glasgow, Glasgow, G12 8QQ, UK
B. Barr, R. Bassiri, A. S. Bell, C. Bell, N. Beveridge, P. Campsie, K. Craig, A. Cumming, L. Cunningham, G. S. Davies, K. Evans, C. Gill, N. Gordon, A. Grant, G. Hammond, K. Haughian, M. A. Hendry, I. S. Heng, S. Hild, J. Hough, S. H. Huttner, R. Jones, R. Kumar, C. Lawrie, J. Logue, J. Macarthur, I. W. Martin, P. G. Murray, G. Newton, M. Pitkin, N. A. Robertson, S. Rowan, I. Santiago-Prieto, J. Scott, B. Sorazu, K. A. Strain, C. I. Torrie, A. A. van Veggel & G. Woan
LIGO – Livingston Observatory, Livingston, 70754, Louisiana, USA
C. Adams, S. M. Aston, J. Betzwieser, J. Birch, D. O. Bridges, M. Cowart, T. Evans, E. Forsi, V. V. Frolov, M. Fyffe, J. A. Giaime, K. D. Giardina, C. Guido, J. Hanson, K. Holt, T. Huynh-Dinh, W. Katzman, D. L. Kinzel, A. Le Roux, M. Lormand, M. S. Meyer, D. Nolting, B. O'Reilly, H. Overmier, C. Ramet, V. Raymond, R. Riesen, S. Roddy, J. H. Romie, D. Sellers, A. L. Stuver, M. Thomas, K. A. Thorne, G. Traylor, T. Welborn, R. Wooley & I. Yakushin
Albert-Einstein-Institut, Max-Planck-Institut für Gravitationsphysik, Hannover, D-30167, Germany
C. Affeldt, B. Allen, S. Ast, P. Aufmuth, C. Aulbert, J. Bauchrowitz, G. Bergmann, A. Bertolini, O. Bock, C. Bogan, M. Born, J. Breyer, M. Brinkmann, M. Britzger, K. Dahl, M. Damjanic, K. Danzmann, T. Denker, T. Dent, I. Di Palma, K. L. Dooley, T. Eberle, H. Fehrmann, M. Frede, T. T. Fricke, D. Friedrich, E. Goetz, S. Goßler, C. Graef, H. Grote, M. Heurs, M. Hewitson, H. Kaufer, F. Kawazoe, D. Keitel, D. G. Keppel, A. Khalaidovski, V. Kringel, B. Krishnan, G. Kuehn, J. R. Leong, H. Lück, A. P. Lundgren, B. Machenschalk, G. Mazzolo, M. Mehmet, T. Meier, F. Mokler, K. Mossavi, C. M. Mow-Lowry, P. Oppermann, M. Pickenpack, H. J. Pletsch, J. Pöld, M. Prijatelj, C. Röver, A. Rüdiger, F. Salemi, R. Schilling, R. Schnabel, D. Schuette, B. Schulz, M. Shaltev, D. Simakov, J. Slutsky, J. Steinlechner, S. Steinlechner, S. P. Tarabrin, H. Vahlbruch, A. Wanner, M. Was, M. Weinert, P. Wessels, T. Westphal, K. Wette, K. Wiesner, B. Willke, M. Wimmer, L. Winkelmann, W. Winkler & H. Wittel
University of Wisconsin–Milwaukee, Milwaukee, 53201, Wisconsin, USA
B. Allen, E. Amador Ceron, W. G. Anderson, P. R. Brady, S. Caudill, J. D. E. Creighton, M. Favata, S. Giampanis, D. Hammer, M. Huynh, J. Kline, S. Koranda, R. A. Mercer, B. Moe, R. O'Shaughnessy, E. Ochsner, C. Pankow, M. A. Papa, X. Siemens, G. R. Skelton, L. Wade, M. Wade & A. G. Wiseman
Stanford University, Stanford, 94305, California, USA
R. Bassiri, T. Bhadbhade, R. L. Byer, D. E. Clark, N. Kim, C. Kucharczyk, R. Kurdyumov, B. Lantz, A. C. Lin, A. S. Markosyan, M. Roberts & B. Shapiro
LIGO – Hanford Observatory, Richland, 99352, Washington, USA
D. Atkinson, D. Barker, M. A. Barton, J. Batch, J. M. Berliner, B. Bland, F. Clara, D. Cook, J. Garcia, C. Gray, J. Hanks, D. R. Ingram, D. Jones, K. Kawabe, M. Landry, B. Levine, V. Lhuillier, M. Lubinski, R. McCarthy, G. Mendell, D. Moraru, G. Moreno, H. Paris, F. J. Raab, H. Radkins, C. M. Reed, M. Rodruck, K. Ryan, V. Sandberg, R. L. Savage, P. Schwinberg, D. Sigg, E. Steinert, P. Thomas, T. Vo, C. Vorvick, C. Wilkinson & J. Worden
University of Florida, Gainesville, 32611, Florida, USA
D. Amariutei, Y. Bao, G. Ciani, E. Deleeuw, S. S. Eikenberry, D. Feldbaum, P. J. Fulda, J. Gleason, M. T. Hartman, M. C. Heintze, S. Klimenko, Z. Liu, R. M. Martin, G. Mitselmakher, C. L. Mueller, G. Mueller, A. Mytidis, D. Nanda Kumar, V. Necula, R. S. Ottens, D. B. Tanner, V. Tiwari, B. F. Whiting & L. Williams
Louisiana State University, Baton Rouge, 70803, Louisiana, USA
T. D. Abbott, J. Bowers, T. R. Corbitt, R. DeRosa, A. Effler, J. A. Giaime, G. González, W. W. Johnson, K. Kokeyama, G. May & A. Mullavey
University of Birmingham, Birmingham, B15 2TT, UK
B. E. Aylott, C. Bond, D. D. Brown, F. Brückner, L. Carbone, A. Freise, K. Grover, D. Lodhia, I. Mandel, C. M. F. Mingarelli, T. L. Sidery, R. J. E. Smith, A. Vecchio, W. D. Vousden & M. Wang
Leibniz Universität Hannover, Hannover, D-30167, Germany
C. Affeldt, B. Allen, S. Ast, P. Aufmuth, C. Aulbert, J. Bauchrowitz, G. Bergmann, A. Bertolini, O. Bock, C. Bogan, M. Born, J. Breyer, M. Brinkmann, M. Britzger, K. Dahl, M. Damjanic, K. Danzmann, T. Denker, T. Dent, I. Di Palma, K. L. Dooley, T. Eberle, H. Fehrmann, M. Frede, T. T. Fricke, D. Friedrich, E. Goetz, S. Goßler, C. Graef, H. Grote, M. Heurs, M. Hewitson, H. Kaufer, F. Kawazoe, D. Keitel, D. G. Keppel, A. Khalaidovski, V. Kringel, B. Krishnan, G. Kuehn, J. R. Leong, H. Lück, A. P. Lundgren, B. Machenschalk, G. Mazzolo, M. Mehmet, T. Meier, F. Mokler, K. Mossavi, C. M. Mow-Lowry, P. Oppermann, M. Pickenpack, H. J. Pletsch, J. Pöld, M. Prijatelj, C. Röver, A. Rüdiger, F. Salemi, R. Schilling, R. Schnabel, D. Schuette, B. Schulz, M. Shaltev, D. Simakov, J. Slutsky, J. Steinlechner, S. Steinlechner, S. P. Tarabrin, H. Vahlbruch, A. Wanner, M. Was, M. Weinert, P. Wessels, T. Westphal, K. Wette, K. Wiesner, B. Willke, M. Wimmer, L. Winkelmann, W. Winkler & H. Wittel
Albert-Einstein-Institut, Max-Planck-Institut für Gravitationsphysik, Golm, D-14476, Germany
S. Babak, B. Behnke, S. Drasco, S. Grunewald, P. Leaci, G. Manca, M. A. Papa, C. Peralta, E. L. Robinson & B. F. Schutz
Montana State University, Bozeman, 59717, Montana, USA
P. T. Baker & N. Cornish
Carleton College, Northfield, Minnesota, 55057, USA
N. Christensen
LIGO – Massachusetts Institute of Technology, Cambridge, 02139, Massachussetts, USA
L. Barsotti, S. Biscans, T. P. Bodiya, F. Donovan, S. Dwyer, R. Essick, M. Evans, S. Foley, P. Fritschel, S. Gras, T. Isogai, E. Katsavounidis, J. S. Kissel, P. Kwee, M. MacInnis, K. Mason, F. Matichard, N. Mavalvala, R. Mittleman, E. Oelker, S. Sankar, D. H. Shoemaker, R. Vaulin, S. Vitale, S. J. Waldman, R. Weiss, C. C. Wipf, F. Zhang & M. E. Zucker
University of Western Australia, Crawley, 6009, Western Australia, Australia
D. Blair, X. Chen, Q. Chu, D. M. Coward, S. L. Danilishin, J-C. Dumas, Q. Fang, S. Hooper, E. J. Howell, L. Ju, S. Susmithan, S. Verma, L. Wen, S. E. Whitcomb, C. Zhao & X. J. Zhu
Columbia University, New York, 10027, New York, USA
I. Bartos, S. Countryman, M. Factourovich, S. Márka, Z. Márka, L. Matone, D. Murphy, P. Raffai & M. Tse
The University of Texas at Brownsville, Brownsville, 78520, Texas, USA
T. D. Creighton, H. Daveloza, M. Díaz, S. R. Morriss, S. Mukherjee, M. E. Normandin, O. Puncken, V. Quetschke, M. Rakhmanov, R. Stone, A. S. Stroeer & C. V. Torres
San Jose State University, San Jose, 95192, California, USA
P. T. Beyersdorf, M. Cordier & K. McAuley
Moscow State University, Moscow, 119992, Russia
I. A. Bilenko, V. B. Braginsky, M. L. Gorodetsky, F. Y. Khalili, V. P. Mitrofanov, L. G. Prokhorov, S. E. Strigin & S. P. Vyatchanin
The Pennsylvania State University, University Park, 16802, Pennsylvania, USA
L. S. Finn, B. J. Owen & H. Zhu
Washington State University, Pullman, 99164, Washington, USA
S. Bose, T. Dayanga, S. Ghosh & S. Steplewski
Caltech–CaRT, Pasadena, 91125, California, USA
Y. Chen, S. Gossan, T. Hong, H. Miao, C. D. Ott, K. S. Thorne, M. Vallisneri & H. Yang
University of Oregon, Eugene, 97403, Oregon, USA
J. E. Brau, R. Frey, E. D. Harstad, R. Quitzow-James, R. M. S. Schofield & D. Talukder
Syracuse University, Syracuse, 13244, New York, USA
S. Ballmer, D. A. Brown, P. Couvares, R. P. Fisher, I. W. Harry, E. A. Huerta, D. B. Kelley, P. Kumar, J. Lough, S. R. P. Mohapatra, A. Nitz, A. Perreca, P. R. Saulson & M. West
Rutherford Appleton Laboratory, HSIC, Chilton, Didcot, Oxon, OX11 0QX, UK
R. J. S. Greenhalgh & J. O'Dell
University of Maryland, College Park, 20742, Maryland, USA
A. Buonanno, C. D. Capano, Y. Pan, P. Shawhan & C. C. Yancey
University of Massachusetts – Amherst, Amherst, 01003, Massachusetts, USA
L. Cadonati, J. A. Clark, D. Hoak, A. L. Lombardi & J. McIver
The University of Mississippi, University, Mississippi, 38677, USA
C. Arceneaux, M. Cavaglià & A. Dietz
NASA/Goddard Space Flight Center, Greenbelt, 20771, Maryland, USA
L. Blackburn, J. B. Camp, N. Gehrels, P. B. Graff & J. B. Kanner
Tsinghua University, Beijing, 100084, China
J. Cao, Z. Du, Y. Liu, Y. Wan & X. Wang
University of Michigan, Ann Arbor, 48109, Michigan, USA
S. Caride, R. Gustafson, G. D. Meadors, K. Riles & J. Sanders
Charles Sturt University, Wagga Wagga, 2678, New South Wales, Australia
P. Charlton
Australian National University, Canberra, Australian Capital Territory, 0200, Australia
B. C. Buchler, J. H. Chow, S. S. Y Chua, R. Inta, P. K. Lam, D. E. McClelland, J. Miller, T. Nguyen, S. M. Scott, D. A. Shaddock, B. J. J Slagmolen, M. Stefszky, A. Stochino, A. Wade & R. L. Ward
The University of Melbourne, Parkville, Victoria, 3010, Australia
C. T. Y. Chung, P. D. Lasky, A. Melatos & L. Sammut
Cardiff University, Cardiff, CF24 3AA, UK
T. Adams, M. Edwards, S. Fairhurst, E. Macdonald, D. M. Macleod, C. Messenger, L. K. Nuttall, F. Ohme, V. Predoi, B. S. Sathyaprakash, B. F. Schutz, P. J. Sutton & J. Veitch
University of Salerno, I- 84084 Fisciano (Salerno), and INFN (Sezione di Napoli), Italy
F. Postiglione
The University of Sheffield, Sheffield, S10 2TN, UK
E. J. Daw, C. Tomlinson & D. J. White
Inter-University Centre for Astronomy and Astrophysics, Pune, 11007, India
S. Dhurandhar, S. Mitra & T. Souradeep
Southern University and A&M College, Baton Rouge, 70813, Louisiana, USA
S. C. McGuire & R. Vincent-Finley
University of Minnesota, Minneapolis, 55455, Minnesota, USA
S. Kandhasamy, A. Kremin, V. Mandic & T. Prestegard
California Institute of Technology, Pasadena, 91125, California, USA
R. W. P. Drever
Northwestern University, Evanston, 60208, Illinois, USA
B. F. Farr, W. Farr, D. Fazi, Y. J. Jang, V. Kalogera, C. Rodriguez, M. S. Shahriar, D. Stevens, M. V. van der Sluys, J. Yablon & H. Yum
The University of Texas at Austin, Austin, 78712, Texas, USA
R. A. Matzner & L. Rodriguez
MTA–Eotvos University, Lendulet A. R. G, Budapest, 1117, Hungary
Z. Frei, G. Gelencser & G. Szeifert
Embry–Riddle Aeronautical University, Prescott, 86301, Arizona, USA
A. M. Gretarsson, B. Hughey, E. Jesse, K. Loew & M. Zanolin
National Astronomical Observatory of Japan, Tokyo, 181-8588, Japan
M-K. Fujimoto, K. Hayama, K. Izumi, S. Kawamura, T. Mori, E. Nishida & A. Nishizawa
University of Adelaide, Adelaide, South, Australia, 5005, Australia
J. Munch, D. J. Ottaway & P. J. Veitch
Universitat de les Illes Balears, Palma de Mallorca, E-07122, Spain
J. Burguet-Castell, S. Gil-Casanova & A. M. Sintes
University of Southampton, Southampton, SO17 1BJ, UK
D. I. Jones
Institute of Applied Physics, Nizhny Novgorod, 603950, Russia
E. A. Khazanov & A. Sergeev
SUPA, University of Strathclyde, Glasgow, G1 1XQ, UK
N. A. Lockerbie & K. V. Tokmakov
Abilene Christian University, Abilene, 79699, Texas, USA
J. L. Willis
Hobart and William Smith Colleges, Geneva, 14456, New York, USA
R. Kasturi & S. Penn
University of Sannio at Benevento, I-82100 Benevento, and INFN (Sezione di Napoli), Italy
P. Addesso, R. DeSalvo, V. Pierro & I. M. Pinto
Louisiana Tech University, Ruston, 71272, Louisiana, USA
T. Reed & N. Zotov
Andrews University, Berrien Springs, 49104, Michigan, USA
T. Z. Summerscales
McNeese State University, Lake Charles, Louisiana, 70609, USA
G. Santostasi
California State University Fullerton, Fullerton, 92831, California, USA
C. Griffo, B. J. Kuper, J. Lee, F. Magaña-Sandoval, C. Padilla & J. R. Smith
Trinity University, San Antonio, 78212, Texas, USA
D. Ugolini
Rochester Institute of Technology, Rochester, 14623, New York, USA
A. D. Castiglia, M. A. Frei, S. R. P. Mohapatra & J. T. Whelan
Southeastern Louisiana University, Hammond, 70402, Louisiana, USA
W. Parkinson & S. Yoshida
Canadian Institute for Theoretical Astrophysics, University of Toronto, Toronto, M5S 3H8, Ontario, Canada
K. Cannon
Pusan National University, Busan, 609-735, Korea
H-S. Cho, Y-M. Kim & C-H. Lee
Hanyang University, Seoul, 133-791, Korea
K. Kim & H. K. Lee
Korea Institute of Science and Technology Information, Daejeon, 305-806, Korea
H. Jang, G. Kang, B. K. Kim & C. Kim
National Institute for Mathematical Sciences, Daejeon, 305-390, Korea
J. J. Oh, S. H. Oh & E. J. Son
Seoul National University, Seoul, 151-742, Korea
H. M. Lee
University of Szeged, 6720 Szeged, Dóm tér, 9, Hungary
L. Á. Gergely
Perimeter Institute for Theoretical Physics, Ontario, N2L 2Y5, Canada
C. Hanna
University of New Hampshire, Durham, New Hampshire, 03824, USA
M. Holtrop
University of Cambridge, Cambridge, CB2 1TN, UK
M. W. Coughlin & J. Gair
American University, Washington, DC 20016, USA
G. M. Harry
Instituto Nacional de Pesquisas Espaciais, 12227-010 – São José dos Campos, SP, Brazil
O. D. Aguiar, M. Constancio Junior & C. A. Costa
University of Washington, Seattle, 98195-4290, Washington, USA
K. Venkateswara
National Tsing Hua University, Hsinchu Taiwan, 300, China
S. Chao, V. Huang, J. Ou & J. Wang
SUPA, University of the West of Scotland, Paisley, PA1 2BE, UK
S. Reid
The George Washington University, Washington, DC 20052, USA
A. Corsi
Raman Research Institute, Bangalore, Karnataka, 560080, India
B. R. Iyer
Universidad Nacional de Cordoba, Cordoba, 5000, Argentina
C. Kozameh
IISER-Kolkata, Mohanpur West, Bengal, 741252, India
R. Nayak
IISER–TVM, CET Campus, Trivandrum Kerala, 695016, India
K. Haris & A. Pai
RRCAT, Indore MP, 452013, India
S. Raja
Indian Institute of Technology, Gandhinagar Ahmedabad Gujarat, 382424, India
A. S. Sengupta
Tata Institute for Fundamental Research, Mumbai, 400005, India
C. S. Unnikrishnan
- J. Aasi
You can also search for this author in PubMed Google Scholar
- J. Abadie
You can also search for this author in PubMed Google Scholar
- B. P. Abbott
You can also search for this author in PubMed Google Scholar
- R. Abbott
You can also search for this author in PubMed Google Scholar
- T. D. Abbott
You can also search for this author in PubMed Google Scholar
- M. R. Abernathy
You can also search for this author in PubMed Google Scholar
- C. Adams
You can also search for this author in PubMed Google Scholar
- T. Adams
You can also search for this author in PubMed Google Scholar
- P. Addesso
You can also search for this author in PubMed Google Scholar
- R. X. Adhikari
You can also search for this author in PubMed Google Scholar
- C. Affeldt
You can also search for this author in PubMed Google Scholar
- O. D. Aguiar
You can also search for this author in PubMed Google Scholar
- P. Ajith
You can also search for this author in PubMed Google Scholar
- B. Allen
You can also search for this author in PubMed Google Scholar
- E. Amador Ceron
You can also search for this author in PubMed Google Scholar
- D. Amariutei
You can also search for this author in PubMed Google Scholar
- S. B. Anderson
You can also search for this author in PubMed Google Scholar
- W. G. Anderson
You can also search for this author in PubMed Google Scholar
- K. Arai
You can also search for this author in PubMed Google Scholar
- M. C. Araya
You can also search for this author in PubMed Google Scholar
- C. Arceneaux
You can also search for this author in PubMed Google Scholar
- S. Ast
You can also search for this author in PubMed Google Scholar
- S. M. Aston
You can also search for this author in PubMed Google Scholar
- D. Atkinson
You can also search for this author in PubMed Google Scholar
- P. Aufmuth
You can also search for this author in PubMed Google Scholar
- C. Aulbert
You can also search for this author in PubMed Google Scholar
- L. Austin
You can also search for this author in PubMed Google Scholar
- B. E. Aylott
You can also search for this author in PubMed Google Scholar
- S. Babak
You can also search for this author in PubMed Google Scholar
- P. T. Baker
You can also search for this author in PubMed Google Scholar
- S. Ballmer
You can also search for this author in PubMed Google Scholar
- Y. Bao
You can also search for this author in PubMed Google Scholar
- J. C. Barayoga
You can also search for this author in PubMed Google Scholar
- D. Barker
You can also search for this author in PubMed Google Scholar
- B. Barr
You can also search for this author in PubMed Google Scholar
- L. Barsotti
You can also search for this author in PubMed Google Scholar
- M. A. Barton
You can also search for this author in PubMed Google Scholar
- I. Bartos
You can also search for this author in PubMed Google Scholar
- R. Bassiri
You can also search for this author in PubMed Google Scholar
- J. Batch
You can also search for this author in PubMed Google Scholar
- J. Bauchrowitz
You can also search for this author in PubMed Google Scholar
- B. Behnke
You can also search for this author in PubMed Google Scholar
- A. S. Bell
You can also search for this author in PubMed Google Scholar
- C. Bell
You can also search for this author in PubMed Google Scholar
- G. Bergmann
You can also search for this author in PubMed Google Scholar
- J. M. Berliner
You can also search for this author in PubMed Google Scholar
- A. Bertolini
You can also search for this author in PubMed Google Scholar
- J. Betzwieser
You can also search for this author in PubMed Google Scholar
- N. Beveridge
You can also search for this author in PubMed Google Scholar
- P. T. Beyersdorf
You can also search for this author in PubMed Google Scholar
- T. Bhadbhade
You can also search for this author in PubMed Google Scholar
- I. A. Bilenko
You can also search for this author in PubMed Google Scholar
- G. Billingsley
You can also search for this author in PubMed Google Scholar
- J. Birch
You can also search for this author in PubMed Google Scholar
- S. Biscans
You can also search for this author in PubMed Google Scholar
- E. Black
You can also search for this author in PubMed Google Scholar
- J. K. Blackburn
You can also search for this author in PubMed Google Scholar
- L. Blackburn
You can also search for this author in PubMed Google Scholar
- D. Blair
You can also search for this author in PubMed Google Scholar
- B. Bland
You can also search for this author in PubMed Google Scholar
- O. Bock
You can also search for this author in PubMed Google Scholar
- T. P. Bodiya
You can also search for this author in PubMed Google Scholar
- C. Bogan
You can also search for this author in PubMed Google Scholar
- C. Bond
You can also search for this author in PubMed Google Scholar
- R. Bork
You can also search for this author in PubMed Google Scholar
- M. Born
You can also search for this author in PubMed Google Scholar
- S. Bose
You can also search for this author in PubMed Google Scholar
- J. Bowers
You can also search for this author in PubMed Google Scholar
- P. R. Brady
You can also search for this author in PubMed Google Scholar
- V. B. Braginsky
You can also search for this author in PubMed Google Scholar
- J. E. Brau
You can also search for this author in PubMed Google Scholar
- J. Breyer
You can also search for this author in PubMed Google Scholar
- D. O. Bridges
You can also search for this author in PubMed Google Scholar
- M. Brinkmann
You can also search for this author in PubMed Google Scholar
- M. Britzger
You can also search for this author in PubMed Google Scholar
- A. F. Brooks
You can also search for this author in PubMed Google Scholar
- D. A. Brown
You can also search for this author in PubMed Google Scholar
- D. D. Brown
You can also search for this author in PubMed Google Scholar
- K. Buckland
You can also search for this author in PubMed Google Scholar
- F. Brückner
You can also search for this author in PubMed Google Scholar
- B. C. Buchler
You can also search for this author in PubMed Google Scholar
- A. Buonanno
You can also search for this author in PubMed Google Scholar
- J. Burguet-Castell
You can also search for this author in PubMed Google Scholar
- R. L. Byer
You can also search for this author in PubMed Google Scholar
- L. Cadonati
You can also search for this author in PubMed Google Scholar
- J. B. Camp
You can also search for this author in PubMed Google Scholar
- P. Campsie
You can also search for this author in PubMed Google Scholar
- K. Cannon
You can also search for this author in PubMed Google Scholar
- J. Cao
You can also search for this author in PubMed Google Scholar
- C. D. Capano
You can also search for this author in PubMed Google Scholar
- L. Carbone
You can also search for this author in PubMed Google Scholar
- S. Caride
You can also search for this author in PubMed Google Scholar
- A. D. Castiglia
You can also search for this author in PubMed Google Scholar
- S. Caudill
You can also search for this author in PubMed Google Scholar
- M. Cavaglià
You can also search for this author in PubMed Google Scholar
- C. Cepeda
You can also search for this author in PubMed Google Scholar
- T. Chalermsongsak
You can also search for this author in PubMed Google Scholar
- S. Chao
You can also search for this author in PubMed Google Scholar
- P. Charlton
You can also search for this author in PubMed Google Scholar
- X. Chen
You can also search for this author in PubMed Google Scholar
- Y. Chen
You can also search for this author in PubMed Google Scholar
- H-S. Cho
You can also search for this author in PubMed Google Scholar
- J. H. Chow
You can also search for this author in PubMed Google Scholar
- N. Christensen
You can also search for this author in PubMed Google Scholar
- Q. Chu
You can also search for this author in PubMed Google Scholar
- S. S. Y Chua
You can also search for this author in PubMed Google Scholar
- C. T. Y. Chung
You can also search for this author in PubMed Google Scholar
- G. Ciani
You can also search for this author in PubMed Google Scholar
- F. Clara
You can also search for this author in PubMed Google Scholar
- D. E. Clark
You can also search for this author in PubMed Google Scholar
- J. A. Clark
You can also search for this author in PubMed Google Scholar
- M. Constancio Junior
You can also search for this author in PubMed Google Scholar
- D. Cook
You can also search for this author in PubMed Google Scholar
- T. R. Corbitt
You can also search for this author in PubMed Google Scholar
- M. Cordier
You can also search for this author in PubMed Google Scholar
- N. Cornish
You can also search for this author in PubMed Google Scholar
- A. Corsi
You can also search for this author in PubMed Google Scholar
- C. A. Costa
You can also search for this author in PubMed Google Scholar
- M. W. Coughlin
You can also search for this author in PubMed Google Scholar
- S. Countryman
You can also search for this author in PubMed Google Scholar
- P. Couvares
You can also search for this author in PubMed Google Scholar
- D. M. Coward
You can also search for this author in PubMed Google Scholar
- M. Cowart
You can also search for this author in PubMed Google Scholar
- D. C. Coyne
You can also search for this author in PubMed Google Scholar
- K. Craig
You can also search for this author in PubMed Google Scholar
- J. D. E. Creighton
You can also search for this author in PubMed Google Scholar
- T. D. Creighton
You can also search for this author in PubMed Google Scholar
- A. Cumming
You can also search for this author in PubMed Google Scholar
- L. Cunningham
You can also search for this author in PubMed Google Scholar
- K. Dahl
You can also search for this author in PubMed Google Scholar
- M. Damjanic
You can also search for this author in PubMed Google Scholar
- S. L. Danilishin
You can also search for this author in PubMed Google Scholar
- K. Danzmann
You can also search for this author in PubMed Google Scholar
- B. Daudert
You can also search for this author in PubMed Google Scholar
- H. Daveloza
You can also search for this author in PubMed Google Scholar
- G. S. Davies
You can also search for this author in PubMed Google Scholar
- E. J. Daw
You can also search for this author in PubMed Google Scholar
- T. Dayanga
You can also search for this author in PubMed Google Scholar
- E. Deleeuw
You can also search for this author in PubMed Google Scholar
- T. Denker
You can also search for this author in PubMed Google Scholar
- T. Dent
You can also search for this author in PubMed Google Scholar
- V. Dergachev
You can also search for this author in PubMed Google Scholar
- R. DeRosa
You can also search for this author in PubMed Google Scholar
- R. DeSalvo
You can also search for this author in PubMed Google Scholar
- S. Dhurandhar
You can also search for this author in PubMed Google Scholar
- I. Di Palma
You can also search for this author in PubMed Google Scholar
- M. Díaz
You can also search for this author in PubMed Google Scholar
- A. Dietz
You can also search for this author in PubMed Google Scholar
- F. Donovan
You can also search for this author in PubMed Google Scholar
- K. L. Dooley
You can also search for this author in PubMed Google Scholar
- S. Doravari
You can also search for this author in PubMed Google Scholar
- S. Drasco
You can also search for this author in PubMed Google Scholar
- R. W. P. Drever
You can also search for this author in PubMed Google Scholar
- J. C. Driggers
You can also search for this author in PubMed Google Scholar
- Z. Du
You can also search for this author in PubMed Google Scholar
- J-C. Dumas
You can also search for this author in PubMed Google Scholar
- S. Dwyer
You can also search for this author in PubMed Google Scholar
- T. Eberle
You can also search for this author in PubMed Google Scholar
- M. Edwards
You can also search for this author in PubMed Google Scholar
- A. Effler
You can also search for this author in PubMed Google Scholar
- P. Ehrens
You can also search for this author in PubMed Google Scholar
- S. S. Eikenberry
You can also search for this author in PubMed Google Scholar
- R. Engel
You can also search for this author in PubMed Google Scholar
- R. Essick
You can also search for this author in PubMed Google Scholar
- T. Etzel
You can also search for this author in PubMed Google Scholar
- K. Evans
You can also search for this author in PubMed Google Scholar
- M. Evans
You can also search for this author in PubMed Google Scholar
- T. Evans
You can also search for this author in PubMed Google Scholar
- M. Factourovich
You can also search for this author in PubMed Google Scholar
- S. Fairhurst
You can also search for this author in PubMed Google Scholar
- Q. Fang
You can also search for this author in PubMed Google Scholar
- B. F. Farr
You can also search for this author in PubMed Google Scholar
- W. Farr
You can also search for this author in PubMed Google Scholar
- M. Favata
You can also search for this author in PubMed Google Scholar
- D. Fazi
You can also search for this author in PubMed Google Scholar
- H. Fehrmann
You can also search for this author in PubMed Google Scholar
- D. Feldbaum
You can also search for this author in PubMed Google Scholar
- L. S. Finn
You can also search for this author in PubMed Google Scholar
- R. P. Fisher
You can also search for this author in PubMed Google Scholar
- S. Foley
You can also search for this author in PubMed Google Scholar
- E. Forsi
You can also search for this author in PubMed Google Scholar
- N. Fotopoulos
You can also search for this author in PubMed Google Scholar
- M. Frede
You can also search for this author in PubMed Google Scholar
- M. A. Frei
You can also search for this author in PubMed Google Scholar
- Z. Frei
You can also search for this author in PubMed Google Scholar
- A. Freise
You can also search for this author in PubMed Google Scholar
- R. Frey
You can also search for this author in PubMed Google Scholar
- T. T. Fricke
You can also search for this author in PubMed Google Scholar
- D. Friedrich
You can also search for this author in PubMed Google Scholar
- P. Fritschel
You can also search for this author in PubMed Google Scholar
- V. V. Frolov
You can also search for this author in PubMed Google Scholar
- M-K. Fujimoto
You can also search for this author in PubMed Google Scholar
- P. J. Fulda
You can also search for this author in PubMed Google Scholar
- M. Fyffe
You can also search for this author in PubMed Google Scholar
- J. Gair
You can also search for this author in PubMed Google Scholar
- J. Garcia
You can also search for this author in PubMed Google Scholar
- N. Gehrels
You can also search for this author in PubMed Google Scholar
- G. Gelencser
You can also search for this author in PubMed Google Scholar
- L. Á. Gergely
You can also search for this author in PubMed Google Scholar
- S. Ghosh
You can also search for this author in PubMed Google Scholar
- J. A. Giaime
You can also search for this author in PubMed Google Scholar
- S. Giampanis
You can also search for this author in PubMed Google Scholar
- K. D. Giardina
You can also search for this author in PubMed Google Scholar
- S. Gil-Casanova
You can also search for this author in PubMed Google Scholar
- C. Gill
You can also search for this author in PubMed Google Scholar
- J. Gleason
You can also search for this author in PubMed Google Scholar
- E. Goetz
You can also search for this author in PubMed Google Scholar
- G. González
You can also search for this author in PubMed Google Scholar
- N. Gordon
You can also search for this author in PubMed Google Scholar
- M. L. Gorodetsky
You can also search for this author in PubMed Google Scholar
- S. Gossan
You can also search for this author in PubMed Google Scholar
- S. Goßler
You can also search for this author in PubMed Google Scholar
- C. Graef
You can also search for this author in PubMed Google Scholar
- P. B. Graff
You can also search for this author in PubMed Google Scholar
- A. Grant
You can also search for this author in PubMed Google Scholar
- S. Gras
You can also search for this author in PubMed Google Scholar
- C. Gray
You can also search for this author in PubMed Google Scholar
- R. J. S. Greenhalgh
You can also search for this author in PubMed Google Scholar
- A. M. Gretarsson
You can also search for this author in PubMed Google Scholar
- C. Griffo
You can also search for this author in PubMed Google Scholar
- H. Grote
You can also search for this author in PubMed Google Scholar
- K. Grover
You can also search for this author in PubMed Google Scholar
- S. Grunewald
You can also search for this author in PubMed Google Scholar
- C. Guido
You can also search for this author in PubMed Google Scholar
- E. K. Gustafson
You can also search for this author in PubMed Google Scholar
- R. Gustafson
You can also search for this author in PubMed Google Scholar
- D. Hammer
You can also search for this author in PubMed Google Scholar
- G. Hammond
You can also search for this author in PubMed Google Scholar
- J. Hanks
You can also search for this author in PubMed Google Scholar
- C. Hanna
You can also search for this author in PubMed Google Scholar
- J. Hanson
You can also search for this author in PubMed Google Scholar
- K. Haris
You can also search for this author in PubMed Google Scholar
- J. Harms
You can also search for this author in PubMed Google Scholar
- G. M. Harry
You can also search for this author in PubMed Google Scholar
- I. W. Harry
You can also search for this author in PubMed Google Scholar
- E. D. Harstad
You can also search for this author in PubMed Google Scholar
- M. T. Hartman
You can also search for this author in PubMed Google Scholar
- K. Haughian
You can also search for this author in PubMed Google Scholar
- K. Hayama
You can also search for this author in PubMed Google Scholar
- J. Heefner
You can also search for this author in PubMed Google Scholar
- M. C. Heintze
You can also search for this author in PubMed Google Scholar
- M. A. Hendry
You can also search for this author in PubMed Google Scholar
- I. S. Heng
You can also search for this author in PubMed Google Scholar
- A. W. Heptonstall
You can also search for this author in PubMed Google Scholar
- M. Heurs
You can also search for this author in PubMed Google Scholar
- M. Hewitson
You can also search for this author in PubMed Google Scholar
- S. Hild
You can also search for this author in PubMed Google Scholar
- D. Hoak
You can also search for this author in PubMed Google Scholar
- K. A. Hodge
You can also search for this author in PubMed Google Scholar
- K. Holt
You can also search for this author in PubMed Google Scholar
- M. Holtrop
You can also search for this author in PubMed Google Scholar
- T. Hong
You can also search for this author in PubMed Google Scholar
- S. Hooper
You can also search for this author in PubMed Google Scholar
- J. Hough
You can also search for this author in PubMed Google Scholar
- E. J. Howell
You can also search for this author in PubMed Google Scholar
- V. Huang
You can also search for this author in PubMed Google Scholar
- E. A. Huerta
You can also search for this author in PubMed Google Scholar
- B. Hughey
You can also search for this author in PubMed Google Scholar
- S. H. Huttner
You can also search for this author in PubMed Google Scholar
- M. Huynh
You can also search for this author in PubMed Google Scholar
- T. Huynh-Dinh
You can also search for this author in PubMed Google Scholar
- D. R. Ingram
You can also search for this author in PubMed Google Scholar
- R. Inta
You can also search for this author in PubMed Google Scholar
- T. Isogai
You can also search for this author in PubMed Google Scholar
- A. Ivanov
You can also search for this author in PubMed Google Scholar
- B. R. Iyer
You can also search for this author in PubMed Google Scholar
- K. Izumi
You can also search for this author in PubMed Google Scholar
- M. Jacobson
You can also search for this author in PubMed Google Scholar
- E. James
You can also search for this author in PubMed Google Scholar
- H. Jang
You can also search for this author in PubMed Google Scholar
- Y. J. Jang
You can also search for this author in PubMed Google Scholar
- E. Jesse
You can also search for this author in PubMed Google Scholar
- W. W. Johnson
You can also search for this author in PubMed Google Scholar
- D. Jones
You can also search for this author in PubMed Google Scholar
- D. I. Jones
You can also search for this author in PubMed Google Scholar
- R. Jones
You can also search for this author in PubMed Google Scholar
- L. Ju
You can also search for this author in PubMed Google Scholar
- P. Kalmus
You can also search for this author in PubMed Google Scholar
- V. Kalogera
You can also search for this author in PubMed Google Scholar
- S. Kandhasamy
You can also search for this author in PubMed Google Scholar
- G. Kang
You can also search for this author in PubMed Google Scholar
- J. B. Kanner
You can also search for this author in PubMed Google Scholar
- R. Kasturi
You can also search for this author in PubMed Google Scholar
- E. Katsavounidis
You can also search for this author in PubMed Google Scholar
- W. Katzman
You can also search for this author in PubMed Google Scholar
- H. Kaufer
You can also search for this author in PubMed Google Scholar
- K. Kawabe
You can also search for this author in PubMed Google Scholar
- S. Kawamura
You can also search for this author in PubMed Google Scholar
- F. Kawazoe
You can also search for this author in PubMed Google Scholar
- D. Keitel
You can also search for this author in PubMed Google Scholar
- D. B. Kelley
You can also search for this author in PubMed Google Scholar
- W. Kells
You can also search for this author in PubMed Google Scholar
- D. G. Keppel
You can also search for this author in PubMed Google Scholar
- A. Khalaidovski
You can also search for this author in PubMed Google Scholar
- F. Y. Khalili
You can also search for this author in PubMed Google Scholar
- E. A. Khazanov
You can also search for this author in PubMed Google Scholar
- B. K. Kim
You can also search for this author in PubMed Google Scholar
- C. Kim
You can also search for this author in PubMed Google Scholar
- K. Kim
You can also search for this author in PubMed Google Scholar
- N. Kim
You can also search for this author in PubMed Google Scholar
- Y-M. Kim
You can also search for this author in PubMed Google Scholar
- P. J. King
You can also search for this author in PubMed Google Scholar
- D. L. Kinzel
You can also search for this author in PubMed Google Scholar
- J. S. Kissel
You can also search for this author in PubMed Google Scholar
- S. Klimenko
You can also search for this author in PubMed Google Scholar
- J. Kline
You can also search for this author in PubMed Google Scholar
- K. Kokeyama
You can also search for this author in PubMed Google Scholar
- V. Kondrashov
You can also search for this author in PubMed Google Scholar
- S. Koranda
You can also search for this author in PubMed Google Scholar
- W. Z. Korth
You can also search for this author in PubMed Google Scholar
- D. Kozak
You can also search for this author in PubMed Google Scholar
- C. Kozameh
You can also search for this author in PubMed Google Scholar
- A. Kremin
You can also search for this author in PubMed Google Scholar
- V. Kringel
You can also search for this author in PubMed Google Scholar
- B. Krishnan
You can also search for this author in PubMed Google Scholar
- C. Kucharczyk
You can also search for this author in PubMed Google Scholar
- G. Kuehn
You can also search for this author in PubMed Google Scholar
- P. Kumar
You can also search for this author in PubMed Google Scholar
- R. Kumar
You can also search for this author in PubMed Google Scholar
- B. J. Kuper
You can also search for this author in PubMed Google Scholar
- R. Kurdyumov
You can also search for this author in PubMed Google Scholar
- P. Kwee
You can also search for this author in PubMed Google Scholar
- P. K. Lam
You can also search for this author in PubMed Google Scholar
- M. Landry
You can also search for this author in PubMed Google Scholar
- B. Lantz
You can also search for this author in PubMed Google Scholar
- P. D. Lasky
You can also search for this author in PubMed Google Scholar
- C. Lawrie
You can also search for this author in PubMed Google Scholar
- A. Lazzarini
You can also search for this author in PubMed Google Scholar
- A. Le Roux
You can also search for this author in PubMed Google Scholar
- P. Leaci
You can also search for this author in PubMed Google Scholar
- C-H. Lee
You can also search for this author in PubMed Google Scholar
- H. K. Lee
You can also search for this author in PubMed Google Scholar
- H. M. Lee
You can also search for this author in PubMed Google Scholar
- J. Lee
You can also search for this author in PubMed Google Scholar
- J. R. Leong
You can also search for this author in PubMed Google Scholar
- B. Levine
You can also search for this author in PubMed Google Scholar
- V. Lhuillier
You can also search for this author in PubMed Google Scholar
- A. C. Lin
You can also search for this author in PubMed Google Scholar
- V. Litvine
You can also search for this author in PubMed Google Scholar
- Y. Liu
You can also search for this author in PubMed Google Scholar
- Z. Liu
You can also search for this author in PubMed Google Scholar
- N. A. Lockerbie
You can also search for this author in PubMed Google Scholar
- D. Lodhia
You can also search for this author in PubMed Google Scholar
- K. Loew
You can also search for this author in PubMed Google Scholar
- J. Logue
You can also search for this author in PubMed Google Scholar
- A. L. Lombardi
You can also search for this author in PubMed Google Scholar
- M. Lormand
You can also search for this author in PubMed Google Scholar
- J. Lough
You can also search for this author in PubMed Google Scholar
- M. Lubinski
You can also search for this author in PubMed Google Scholar
- H. Lück
You can also search for this author in PubMed Google Scholar
- A. P. Lundgren
You can also search for this author in PubMed Google Scholar
- J. Macarthur
You can also search for this author in PubMed Google Scholar
- E. Macdonald
You can also search for this author in PubMed Google Scholar
- B. Machenschalk
You can also search for this author in PubMed Google Scholar
- M. MacInnis
You can also search for this author in PubMed Google Scholar
- D. M. Macleod
You can also search for this author in PubMed Google Scholar
- F. Magaña-Sandoval
You can also search for this author in PubMed Google Scholar
- M. Mageswaran
You can also search for this author in PubMed Google Scholar
- K. Mailand
You can also search for this author in PubMed Google Scholar
- G. Manca
You can also search for this author in PubMed Google Scholar
- I. Mandel
You can also search for this author in PubMed Google Scholar
- V. Mandic
You can also search for this author in PubMed Google Scholar
- S. Márka
You can also search for this author in PubMed Google Scholar
- Z. Márka
You can also search for this author in PubMed Google Scholar
- A. S. Markosyan
You can also search for this author in PubMed Google Scholar
- E. Maros
You can also search for this author in PubMed Google Scholar
- I. W. Martin
You can also search for this author in PubMed Google Scholar
- R. M. Martin
You can also search for this author in PubMed Google Scholar
- D. Martinov
You can also search for this author in PubMed Google Scholar
- J. N. Marx
You can also search for this author in PubMed Google Scholar
- K. Mason
You can also search for this author in PubMed Google Scholar
- F. Matichard
You can also search for this author in PubMed Google Scholar
- L. Matone
You can also search for this author in PubMed Google Scholar
- R. A. Matzner
You can also search for this author in PubMed Google Scholar
- N. Mavalvala
You can also search for this author in PubMed Google Scholar
- G. May
You can also search for this author in PubMed Google Scholar
- G. Mazzolo
You can also search for this author in PubMed Google Scholar
- K. McAuley
You can also search for this author in PubMed Google Scholar
- R. McCarthy
You can also search for this author in PubMed Google Scholar
- D. E. McClelland
You can also search for this author in PubMed Google Scholar
- S. C. McGuire
You can also search for this author in PubMed Google Scholar
- G. McIntyre
You can also search for this author in PubMed Google Scholar
- J. McIver
You can also search for this author in PubMed Google Scholar
- G. D. Meadors
You can also search for this author in PubMed Google Scholar
- M. Mehmet
You can also search for this author in PubMed Google Scholar
- T. Meier
You can also search for this author in PubMed Google Scholar
- A. Melatos
You can also search for this author in PubMed Google Scholar
- G. Mendell
You can also search for this author in PubMed Google Scholar
- R. A. Mercer
You can also search for this author in PubMed Google Scholar
- S. Meshkov
You can also search for this author in PubMed Google Scholar
- C. Messenger
You can also search for this author in PubMed Google Scholar
- M. S. Meyer
You can also search for this author in PubMed Google Scholar
- H. Miao
You can also search for this author in PubMed Google Scholar
- J. Miller
You can also search for this author in PubMed Google Scholar
- C. M. F. Mingarelli
You can also search for this author in PubMed Google Scholar
- S. Mitra
You can also search for this author in PubMed Google Scholar
- V. P. Mitrofanov
You can also search for this author in PubMed Google Scholar
- G. Mitselmakher
You can also search for this author in PubMed Google Scholar
- R. Mittleman
You can also search for this author in PubMed Google Scholar
- B. Moe
You can also search for this author in PubMed Google Scholar
- F. Mokler
You can also search for this author in PubMed Google Scholar
- S. R. P. Mohapatra
You can also search for this author in PubMed Google Scholar
- D. Moraru
You can also search for this author in PubMed Google Scholar
- G. Moreno
You can also search for this author in PubMed Google Scholar
- T. Mori
You can also search for this author in PubMed Google Scholar
- S. R. Morriss
You can also search for this author in PubMed Google Scholar
- K. Mossavi
You can also search for this author in PubMed Google Scholar
- C. M. Mow-Lowry
You can also search for this author in PubMed Google Scholar
- C. L. Mueller
You can also search for this author in PubMed Google Scholar
- G. Mueller
You can also search for this author in PubMed Google Scholar
- S. Mukherjee
You can also search for this author in PubMed Google Scholar
- A. Mullavey
You can also search for this author in PubMed Google Scholar
- J. Munch
You can also search for this author in PubMed Google Scholar
- D. Murphy
You can also search for this author in PubMed Google Scholar
- P. G. Murray
You can also search for this author in PubMed Google Scholar
- A. Mytidis
You can also search for this author in PubMed Google Scholar
- D. Nanda Kumar
You can also search for this author in PubMed Google Scholar
- T. Nash
You can also search for this author in PubMed Google Scholar
- R. Nayak
You can also search for this author in PubMed Google Scholar
- V. Necula
You can also search for this author in PubMed Google Scholar
- G. Newton
You can also search for this author in PubMed Google Scholar
- T. Nguyen
You can also search for this author in PubMed Google Scholar
- E. Nishida
You can also search for this author in PubMed Google Scholar
- A. Nishizawa
You can also search for this author in PubMed Google Scholar
- A. Nitz
You can also search for this author in PubMed Google Scholar
- D. Nolting
You can also search for this author in PubMed Google Scholar
- M. E. Normandin
You can also search for this author in PubMed Google Scholar
- L. K. Nuttall
You can also search for this author in PubMed Google Scholar
- J. O'Dell
You can also search for this author in PubMed Google Scholar
- B. O'Reilly
You can also search for this author in PubMed Google Scholar
- R. O'Shaughnessy
You can also search for this author in PubMed Google Scholar
- E. Ochsner
You can also search for this author in PubMed Google Scholar
- E. Oelker
You can also search for this author in PubMed Google Scholar
- G. H. Ogin
You can also search for this author in PubMed Google Scholar
- J. J. Oh
You can also search for this author in PubMed Google Scholar
- S. H. Oh
You can also search for this author in PubMed Google Scholar
- F. Ohme
You can also search for this author in PubMed Google Scholar
- P. Oppermann
You can also search for this author in PubMed Google Scholar
- C. Osthelder
You can also search for this author in PubMed Google Scholar
- C. D. Ott
You can also search for this author in PubMed Google Scholar
- D. J. Ottaway
You can also search for this author in PubMed Google Scholar
- R. S. Ottens
You can also search for this author in PubMed Google Scholar
- J. Ou
You can also search for this author in PubMed Google Scholar
- H. Overmier
You can also search for this author in PubMed Google Scholar
- B. J. Owen
You can also search for this author in PubMed Google Scholar
- C. Padilla
You can also search for this author in PubMed Google Scholar
- A. Pai
You can also search for this author in PubMed Google Scholar
- Y. Pan
You can also search for this author in PubMed Google Scholar
- C. Pankow
You can also search for this author in PubMed Google Scholar
- M. A. Papa
You can also search for this author in PubMed Google Scholar
- H. Paris
You can also search for this author in PubMed Google Scholar
- W. Parkinson
You can also search for this author in PubMed Google Scholar
- M. Pedraza
You can also search for this author in PubMed Google Scholar
- S. Penn
You can also search for this author in PubMed Google Scholar
- C. Peralta
You can also search for this author in PubMed Google Scholar
- A. Perreca
You can also search for this author in PubMed Google Scholar
- M. Phelps
You can also search for this author in PubMed Google Scholar
- M. Pickenpack
You can also search for this author in PubMed Google Scholar
- V. Pierro
You can also search for this author in PubMed Google Scholar
- I. M. Pinto
You can also search for this author in PubMed Google Scholar
- M. Pitkin
You can also search for this author in PubMed Google Scholar
- H. J. Pletsch
You can also search for this author in PubMed Google Scholar
- J. Pöld
You can also search for this author in PubMed Google Scholar
- F. Postiglione
You can also search for this author in PubMed Google Scholar
- C. Poux
You can also search for this author in PubMed Google Scholar
- V. Predoi
You can also search for this author in PubMed Google Scholar
- T. Prestegard
You can also search for this author in PubMed Google Scholar
- L. R. Price
You can also search for this author in PubMed Google Scholar
- M. Prijatelj
You can also search for this author in PubMed Google Scholar
- S. Privitera
You can also search for this author in PubMed Google Scholar
- L. G. Prokhorov
You can also search for this author in PubMed Google Scholar
- O. Puncken
You can also search for this author in PubMed Google Scholar
- V. Quetschke
You can also search for this author in PubMed Google Scholar
- E. Quintero
You can also search for this author in PubMed Google Scholar
- R. Quitzow-James
You can also search for this author in PubMed Google Scholar
- F. J. Raab
You can also search for this author in PubMed Google Scholar
- H. Radkins
You can also search for this author in PubMed Google Scholar
- P. Raffai
You can also search for this author in PubMed Google Scholar
- S. Raja
You can also search for this author in PubMed Google Scholar
- M. Rakhmanov
You can also search for this author in PubMed Google Scholar
- C. Ramet
You can also search for this author in PubMed Google Scholar
- V. Raymond
You can also search for this author in PubMed Google Scholar
- C. M. Reed
You can also search for this author in PubMed Google Scholar
- T. Reed
You can also search for this author in PubMed Google Scholar
- S. Reid
You can also search for this author in PubMed Google Scholar
- D. H. Reitze
You can also search for this author in PubMed Google Scholar
- R. Riesen
You can also search for this author in PubMed Google Scholar
- K. Riles
You can also search for this author in PubMed Google Scholar
- M. Roberts
You can also search for this author in PubMed Google Scholar
- N. A. Robertson
You can also search for this author in PubMed Google Scholar
- E. L. Robinson
You can also search for this author in PubMed Google Scholar
- S. Roddy
You can also search for this author in PubMed Google Scholar
- C. Rodriguez
You can also search for this author in PubMed Google Scholar
- L. Rodriguez
You can also search for this author in PubMed Google Scholar
- M. Rodruck
You can also search for this author in PubMed Google Scholar
- J. G. Rollins
You can also search for this author in PubMed Google Scholar
- J. H. Romie
You can also search for this author in PubMed Google Scholar
- C. Röver
You can also search for this author in PubMed Google Scholar
- S. Rowan
You can also search for this author in PubMed Google Scholar
- A. Rüdiger
You can also search for this author in PubMed Google Scholar
- K. Ryan
You can also search for this author in PubMed Google Scholar
- F. Salemi
You can also search for this author in PubMed Google Scholar
- L. Sammut
You can also search for this author in PubMed Google Scholar
- V. Sandberg
You can also search for this author in PubMed Google Scholar
- J. Sanders
You can also search for this author in PubMed Google Scholar
- S. Sankar
You can also search for this author in PubMed Google Scholar
- V. Sannibale
You can also search for this author in PubMed Google Scholar
- L. Santamaría
You can also search for this author in PubMed Google Scholar
- I. Santiago-Prieto
You can also search for this author in PubMed Google Scholar
- G. Santostasi
You can also search for this author in PubMed Google Scholar
- B. S. Sathyaprakash
You can also search for this author in PubMed Google Scholar
- P. R. Saulson
You can also search for this author in PubMed Google Scholar
- R. L. Savage
You can also search for this author in PubMed Google Scholar
- R. Schilling
You can also search for this author in PubMed Google Scholar
- R. Schnabel
You can also search for this author in PubMed Google Scholar
- R. M. S. Schofield
You can also search for this author in PubMed Google Scholar
- D. Schuette
You can also search for this author in PubMed Google Scholar
- B. Schulz
You can also search for this author in PubMed Google Scholar
- B. F. Schutz
You can also search for this author in PubMed Google Scholar
- P. Schwinberg
You can also search for this author in PubMed Google Scholar
- J. Scott
You can also search for this author in PubMed Google Scholar
- S. M. Scott
You can also search for this author in PubMed Google Scholar
- F. Seifert
You can also search for this author in PubMed Google Scholar
- D. Sellers
You can also search for this author in PubMed Google Scholar
- A. S. Sengupta
You can also search for this author in PubMed Google Scholar
- A. Sergeev
You can also search for this author in PubMed Google Scholar
- D. A. Shaddock
You can also search for this author in PubMed Google Scholar
- M. S. Shahriar
You can also search for this author in PubMed Google Scholar
- M. Shaltev
You can also search for this author in PubMed Google Scholar
- Z. Shao
You can also search for this author in PubMed Google Scholar
- B. Shapiro
You can also search for this author in PubMed Google Scholar
- P. Shawhan
You can also search for this author in PubMed Google Scholar
- D. H. Shoemaker
You can also search for this author in PubMed Google Scholar
- T. L. Sidery
You can also search for this author in PubMed Google Scholar
- X. Siemens
You can also search for this author in PubMed Google Scholar
- D. Sigg
You can also search for this author in PubMed Google Scholar
- D. Simakov
You can also search for this author in PubMed Google Scholar
- A. Singer
You can also search for this author in PubMed Google Scholar
- L. Singer
You can also search for this author in PubMed Google Scholar
- A. M. Sintes
You can also search for this author in PubMed Google Scholar
- G. R. Skelton
You can also search for this author in PubMed Google Scholar
- B. J. J Slagmolen
You can also search for this author in PubMed Google Scholar
- J. Slutsky
You can also search for this author in PubMed Google Scholar
- J. R. Smith
You can also search for this author in PubMed Google Scholar
- M. R. Smith
You can also search for this author in PubMed Google Scholar
- R. J. E. Smith
You can also search for this author in PubMed Google Scholar
- N. D. Smith-Lefebvre
You can also search for this author in PubMed Google Scholar
- E. J. Son
You can also search for this author in PubMed Google Scholar
- B. Sorazu
You can also search for this author in PubMed Google Scholar
- T. Souradeep
You can also search for this author in PubMed Google Scholar
- M. Stefszky
You can also search for this author in PubMed Google Scholar
- E. Steinert
You can also search for this author in PubMed Google Scholar
- J. Steinlechner
You can also search for this author in PubMed Google Scholar
- S. Steinlechner
You can also search for this author in PubMed Google Scholar
- S. Steplewski
You can also search for this author in PubMed Google Scholar
- D. Stevens
You can also search for this author in PubMed Google Scholar
- A. Stochino
You can also search for this author in PubMed Google Scholar
- R. Stone
You can also search for this author in PubMed Google Scholar
- K. A. Strain
You can also search for this author in PubMed Google Scholar
- S. E. Strigin
You can also search for this author in PubMed Google Scholar
- A. S. Stroeer
You can also search for this author in PubMed Google Scholar
- A. L. Stuver
You can also search for this author in PubMed Google Scholar
- T. Z. Summerscales
You can also search for this author in PubMed Google Scholar
- S. Susmithan
You can also search for this author in PubMed Google Scholar
- P. J. Sutton
You can also search for this author in PubMed Google Scholar
- G. Szeifert
You can also search for this author in PubMed Google Scholar
- D. Talukder
You can also search for this author in PubMed Google Scholar
- D. B. Tanner
You can also search for this author in PubMed Google Scholar
- S. P. Tarabrin
You can also search for this author in PubMed Google Scholar
- R. Taylor
You can also search for this author in PubMed Google Scholar
- M. Thomas
You can also search for this author in PubMed Google Scholar
- P. Thomas
You can also search for this author in PubMed Google Scholar
- K. A. Thorne
You can also search for this author in PubMed Google Scholar
- K. S. Thorne
You can also search for this author in PubMed Google Scholar
- E. Thrane
You can also search for this author in PubMed Google Scholar
- V. Tiwari
You can also search for this author in PubMed Google Scholar
- K. V. Tokmakov
You can also search for this author in PubMed Google Scholar
- C. Tomlinson
You can also search for this author in PubMed Google Scholar
- C. V. Torres
You can also search for this author in PubMed Google Scholar
- C. I. Torrie
You can also search for this author in PubMed Google Scholar
- G. Traylor
You can also search for this author in PubMed Google Scholar
- M. Tse
You can also search for this author in PubMed Google Scholar
- D. Ugolini
You can also search for this author in PubMed Google Scholar
- C. S. Unnikrishnan
You can also search for this author in PubMed Google Scholar
- H. Vahlbruch
You can also search for this author in PubMed Google Scholar
- M. Vallisneri
You can also search for this author in PubMed Google Scholar
- M. V. van der Sluys
You can also search for this author in PubMed Google Scholar
- A. A. van Veggel
You can also search for this author in PubMed Google Scholar
- S. Vass
You can also search for this author in PubMed Google Scholar
- R. Vaulin
You can also search for this author in PubMed Google Scholar
- A. Vecchio
You can also search for this author in PubMed Google Scholar
- P. J. Veitch
You can also search for this author in PubMed Google Scholar
- J. Veitch
You can also search for this author in PubMed Google Scholar
- K. Venkateswara
You can also search for this author in PubMed Google Scholar
- S. Verma
You can also search for this author in PubMed Google Scholar
- R. Vincent-Finley
You can also search for this author in PubMed Google Scholar
- S. Vitale
You can also search for this author in PubMed Google Scholar
- T. Vo
You can also search for this author in PubMed Google Scholar
- C. Vorvick
You can also search for this author in PubMed Google Scholar
- W. D. Vousden
You can also search for this author in PubMed Google Scholar
- S. P. Vyatchanin
You can also search for this author in PubMed Google Scholar
- A. Wade
You can also search for this author in PubMed Google Scholar
- L. Wade
You can also search for this author in PubMed Google Scholar
- M. Wade
You can also search for this author in PubMed Google Scholar
- S. J. Waldman
You can also search for this author in PubMed Google Scholar
- L. Wallace
You can also search for this author in PubMed Google Scholar
- Y. Wan
You can also search for this author in PubMed Google Scholar
- M. Wang
You can also search for this author in PubMed Google Scholar
- J. Wang
You can also search for this author in PubMed Google Scholar
- X. Wang
You can also search for this author in PubMed Google Scholar
- A. Wanner
You can also search for this author in PubMed Google Scholar
- R. L. Ward
You can also search for this author in PubMed Google Scholar
- M. Was
You can also search for this author in PubMed Google Scholar
- M. Weinert
You can also search for this author in PubMed Google Scholar
- A. J. Weinstein
You can also search for this author in PubMed Google Scholar
- R. Weiss
You can also search for this author in PubMed Google Scholar
- T. Welborn
You can also search for this author in PubMed Google Scholar
- L. Wen
You can also search for this author in PubMed Google Scholar
- P. Wessels
You can also search for this author in PubMed Google Scholar
- M. West
You can also search for this author in PubMed Google Scholar
- T. Westphal
You can also search for this author in PubMed Google Scholar
- K. Wette
You can also search for this author in PubMed Google Scholar
- J. T. Whelan
You can also search for this author in PubMed Google Scholar
- S. E. Whitcomb
You can also search for this author in PubMed Google Scholar
- A. G. Wiseman
You can also search for this author in PubMed Google Scholar
- D. J. White
You can also search for this author in PubMed Google Scholar
- B. F. Whiting
You can also search for this author in PubMed Google Scholar
- K. Wiesner
You can also search for this author in PubMed Google Scholar
- C. Wilkinson
You can also search for this author in PubMed Google Scholar
- P. A. Willems
You can also search for this author in PubMed Google Scholar
- L. Williams
You can also search for this author in PubMed Google Scholar
- R. Williams
You can also search for this author in PubMed Google Scholar
- T. Williams
You can also search for this author in PubMed Google Scholar
- J. L. Willis
You can also search for this author in PubMed Google Scholar
- B. Willke
You can also search for this author in PubMed Google Scholar
- M. Wimmer
You can also search for this author in PubMed Google Scholar
- L. Winkelmann
You can also search for this author in PubMed Google Scholar
- W. Winkler
You can also search for this author in PubMed Google Scholar
- C. C. Wipf
You can also search for this author in PubMed Google Scholar
- H. Wittel
You can also search for this author in PubMed Google Scholar
- G. Woan
You can also search for this author in PubMed Google Scholar
- R. Wooley
You can also search for this author in PubMed Google Scholar
- J. Worden
You can also search for this author in PubMed Google Scholar
- J. Yablon
You can also search for this author in PubMed Google Scholar
- I. Yakushin
You can also search for this author in PubMed Google Scholar
- H. Yamamoto
You can also search for this author in PubMed Google Scholar
- C. C. Yancey
You can also search for this author in PubMed Google Scholar
- H. Yang
You can also search for this author in PubMed Google Scholar
- D. Yeaton-Massey
You can also search for this author in PubMed Google Scholar
- S. Yoshida
You can also search for this author in PubMed Google Scholar
- H. Yum
You can also search for this author in PubMed Google Scholar
- M. Zanolin
You can also search for this author in PubMed Google Scholar
- F. Zhang
You can also search for this author in PubMed Google Scholar
- L. Zhang
You can also search for this author in PubMed Google Scholar
- C. Zhao
You can also search for this author in PubMed Google Scholar
- H. Zhu
You can also search for this author in PubMed Google Scholar
- X. J. Zhu
You can also search for this author in PubMed Google Scholar
- N. Zotov
You can also search for this author in PubMed Google Scholar
- M. E. Zucker
You can also search for this author in PubMed Google Scholar
- J. Zweizig
You can also search for this author in PubMed Google Scholar
Contributions
The activities of the LIGO Scientific Collaboration (LSC) include modelling astrophysical sources of gravitational waves, setting sensitivity requirements for observatories, designing, building and running observatories, carrying out research and development of new techniques to increase the sensitivity of these observatories, and performing searches for astrophysical signals contained in the data. S. Dwyer, S. Chua, L. Barsotti and D. Sigg were the leading scientists on this experiment, but a number of LSC members contributed directly to its success. M. Stefszky, A. Khalaidovski, M. Factourovich and C. Mow-Lowry assisted with the development of the squeezed vacuum source under the leadership of N. Mavalvala, D. McClelland and R. Schnabel. K. Kawabe supervised the integration of the squeezed vacuum source into the LIGO interferometer, with invaluable support from M. Landry and the LIGO Hanford Observatory staff. N. Smith-Lefebvre, M. Evans, R. Schofield and C. Vorvick kept the LIGO interferometer at its peak sensitivity and supported the integration of the squeezed vacuum source, with contributions from G. Meadors and D. Gustafson. The initial manuscript was written by L. Barsotti, N. Mavalvala, D. Sigg and D. McClelland. The LSC review of the manuscript was organized by S. Whitcomb. All authors approved the final version of the manuscript.
Corresponding author
Correspondence to L. Barsotti.
Ethics declarations
Competing interests
The authors declare no competing financial interests.
Supplementary information
Supplementary information
Supplementary information (PDF 798 kb)
Rights and permissions
About this article
Cite this article
Aasi, J., Abadie, J., Abbott, B. et al. Enhanced sensitivity of the LIGO gravitational wave detector by using squeezed states of light. Nature Photon 7, 613–619 (2013). https://doi.org/10.1038/nphoton.2013.177
Received:
Accepted:
Published:
Issue Date:
DOI: https://doi.org/10.1038/nphoton.2013.177
Share this article
Anyone you share the following link with will be able to read this content:
Sorry, a shareable link is not currently available for this article.
Provided by the Springer Nature SharedIt content-sharing initiative
This article is cited by
-
Correlated sensing with a solid-state quantum multisensor system for atomic-scale structural analysis
- Wentao Ji
- Zhaoxin Liu
- Jiangfeng Du
Nature Photonics (2024)
-
Light squeezing enhancement by coupling nonlinear optical cavities
- H. Jabri
- H. Eleuch
Scientific Reports (2024)
-
A squeezed mechanical oscillator with millisecond quantum decoherence
- Amir Youssefi
- Shingo Kono
- Tobias J. Kippenberg
Nature Physics (2023)
-
Quantum-enhanced sensing by echoing spin-nematic squeezing in atomic Bose–Einstein condensate
- Tian-Wei Mao
- Qi Liu
- Li You
Nature Physics (2023)
-
Entanglement-enhanced dual-comb spectroscopy
- Haowei Shi
- Zaijun Chen
- Quntao Zhuang
npj Quantum Information (2023)