Abstract
A major genetic risk factor for psychosis is 22q11.2 deletion (22q11.2DS). However, robust and replicable functional brain signatures of 22q11.2DS and 22q11.2DS-associated psychosis remain elusive due to small sample sizes and a focus on small single-site cohorts. Here, we identify functional brain signatures of 22q11.2DS and 22q11.2DS-associated psychosis, and their links with idiopathic early psychosis, using one of the largest multi-cohort data to date. We obtained multi-cohort clinical phenotypic and task-free fMRI data from 856 participants (101 22q11.2DS, 120 idiopathic early psychosis, 101 idiopathic autism, 123 idiopathic ADHD, and 411 healthy controls) in a case-control design. A novel spatiotemporal deep neural network (stDNN)-based analysis was applied to the multi-cohort data to identify functional brain signatures of 22q11.2DS and 22q11.2DS-associated psychosis. Next, stDNN was used to test the hypothesis that the functional brain signatures of 22q11.2DS-associated psychosis overlap with idiopathic early psychosis but not with autism and ADHD. stDNN-derived brain signatures distinguished 22q11.2DS from controls, and 22q11.2DS-associated psychosis with very high accuracies (86–94%) in the primary cohort and two fully independent cohorts without additional training. Robust distinguishing features of 22q11.2DS-associated psychosis emerged in the anterior insula node of the salience network and the striatum node of the dopaminergic reward pathway. These features also distinguished individuals with idiopathic early psychosis from controls, but not idiopathic autism or ADHD. Our results reveal that individuals with 22q11.2DS exhibit a highly distinct functional brain organization compared to controls. Additionally, the brain signatures of 22q11.2DS-associated psychosis overlap with those of idiopathic early psychosis in the salience network and dopaminergic reward pathway, providing substantial empirical support for the theoretical aberrant salience-based model of psychosis. Collectively, our findings, replicated across multiple independent cohorts, advance the understanding of 22q11.2DS and associated psychosis, underscoring the value of 22q11.2DS as a genetic model for probing the neurobiological underpinnings of psychosis and its progression.
This is a preview of subscription content, access via your institution
Access options
Subscribe to this journal
Receive 12 print issues and online access
$259.00 per year
only $21.58 per issue
Buy this article
- Purchase on Springer Link
- Instant access to full article PDF
Prices may be subject to local taxes which are calculated during checkout





Similar content being viewed by others
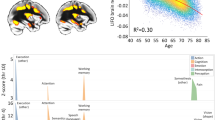
Data availability
The processed fMRI regional time-series data along with demographic information will be made available upon reasonable request.
References
McDonald-McGinn DM, Sullivan KE, Marino B, Philip N, Swillen A, Vorstman JAS, et al. 22q11.2 deletion syndrome. Nat Rev Dis Prim. 2015;1:15071.
Oskarsdottir S, Vujic M, Fasth A. Incidence and prevalence of the 22q11 deletion syndrome: a population-based study in Western Sweden. Arch Dis Child. 2004;89:148–51.
Shprintzen RJ. Velo-cardio-facial syndrome: 30 Years of study. Dev Disabil Res Rev. 2008;14:3–10.
Goodship J, Cross I, LiLing J, Wren C. A population study of chromosome 22q11 deletions in infancy. Arch Dis Child. 1998;79:348–51.
Blagojevic C, Heung T, Theriault M, Tomita-Mitchell A, Chakraborty P, Kernohan K, et al. Estimate of the contemporary live-birth prevalence of recurrent 22q11.2 deletions: a cross-sectional analysis from population-based newborn screening. CMAJ Open. 2021;9:E802–9.
Shaikh TH, O’Connor RJ, Pierpont ME, McGrath J, Hacker AM, Nimmakayalu M, et al. Low copy repeats mediate distal chromosome 22q11.2 deletions: sequence analysis predicts breakpoint mechanisms. Genome Res. 2007;17:482–91.
Edelmann L, Pandita RK, Morrow BE. Low-copy repeats mediate the common 3-Mb deletion in patients with velo-cardio-facial syndrome. Am J Hum Genet. 1999;64:1076–86.
Maynard TM, Haskell GT, Peters AZ, Sikich L, Lieberman JA, LaMantia AS. A comprehensive analysis of 22q11 gene expression in the developing and adult brain. Proc Natl Acad Sci USA. 2003;100:14433–8.
Guna A, Butcher NJ, Bassett AS. Comparative mapping of the 22q11.2 deletion region and the potential of simple model organisms. J Neurodevelopmental Disord. 2015;7:18.
Murphy KC, Jones LA, Owen MJ. High rates of schizophrenia in adults with velo-cardio-facial syndrome. Arch Gen Psychiatry. 1999;56:940–5.
Marshall CR, Howrigan DP, Merico D, Thiruvahindrapuram B, Wu W, Greer DS, et al. Contribution of copy number variants to schizophrenia from a genome-wide study of 41,321 subjects. Nat Genet. 2017;49:27–35.
Bassett AS, Chow EW. Schizophrenia and 22q11.2 deletion syndrome. Curr Psychiatry Rep. 2008;10:148–57.
Green T, Gothelf D, Glaser B, Debbane M, Frisch A, Kotler M, et al. Psychiatric disorders and intellectual functioning throughout development in velocardiofacial (22q11.2 deletion) syndrome. J Am Acad Child Adolesc Psychiatry. 2009;48:1060–8.
Schneider M, Debbane M, Bassett AS, Chow EW, Fung WL, van den Bree M, et al. Psychiatric disorders from childhood to adulthood in 22q11.2 deletion syndrome: results from the International Consortium on Brain and Behavior in 22q11.2 Deletion Syndrome. Am J Psychiatry. 2014;171:627–39.
Siebner HR, Callicott JH, Sommer T, Mattay VS. From the genome to the phenome and back: linking genes with human brain function and structure using genetically informed neuroimaging. Neuroscience. 2009;164:1–6.
Fiksinski AM, Hoftman GD, Vorstman JAS, Bearden CE. A genetics-first approach to understanding autism and schizophrenia spectrum disorders: the 22q11.2 deletion syndrome. Mol Psychiatry. 2023;28:341–53.
Jonas RK, Montojo CA, Bearden CE. The 22q11.2 deletion syndrome as a window into complex neuropsychiatric disorders over the lifespan. Biol Psychiatry. 2014;75:351–60.
Lewandowski KE, Bouix S, Ongur D, Shenton ME. Neuroprogression across the early course of psychosis. J Psychiatry Brain Sci. 2020;5:e200002.
Padula MC, Schaer M, Scariati E, Schneider M, Van De Ville D, Debbane M, et al. Structural and functional connectivity in the default mode network in 22q11.2 deletion syndrome. J Neurodev Disord. 2015;7:23.
Schreiner MJ, Karlsgodt KH, Uddin LQ, Chow C, Congdon E, Jalbrzikowski M, et al. Default mode network connectivity and reciprocal social behavior in 22q11.2 deletion syndrome. Soc Cogn Affect Neurosci. 2014;9:1261–7.
Zoller D, Schaer M, Scariati E, Padula MC, Eliez S, Van De Ville D. Disentangling resting-state BOLD variability and PCC functional connectivity in 22q11.2 deletion syndrome. Neuroimage. 2017;149:85–97.
Debbane M, Lazouret M, Lagioia A, Schneider M, Van De Ville D, Eliez S. Resting-state networks in adolescents with 22q11.2 deletion syndrome: associations with prodromal symptoms and executive functions. Schizophr Res. 2012;139:33–9.
Scariati E, Schaer M, Richiardi J, Schneider M, Debbane M, Van De Ville D, et al. Identifying 22q11.2 deletion syndrome and psychosis using resting-state connectivity patterns. Brain Topogr. 2014;27:808–21.
Schreiner M, Forsyth JK, Karlsgodt KH, Anderson AE, Hirsh N, Kushan L, et al. Intrinsic connectivity network-based classification and detection of psychotic symptoms in youth with 22q11.2 deletions. Cereb Cortex. 2017;27:3294–306.
Mattiaccio LM, Coman IL, Thompson CA, Fremont WP, Antshel KM, Kates WR. Frontal dysconnectivity in 22q11.2 deletion syndrome: an atlas-based functional connectivity analysis. Behav Brain Funct. 2018;14:2.
Schleifer C, Lin A, Kushan L, Ji JL, Yang G, Bearden CE, et al. Dissociable disruptions in thalamic and hippocampal resting-state functional connectivity in youth with 22q11.2 deletions. J Neurosci. 2019;39:1301–19.
Chow EWC, Zipursky RB, Mikulis DJ, Bassett AS. Structural brain abnormalities in patients with schizophrenia and 22q11 deletion Syndrome. Biol Psychiatry. 2002;51:208–15.
Eliez S, Schmitt JE, White CD, Reiss AL. Children and adolescents with velocardiofacial syndrome: a volumetric MRI study. Am J Psychiatry. 2000;157:409–15.
Campbell LE, Daly E, Toal F, Stevens A, Azuma R, Catani M, et al. Brain and behaviour in children with 22q11.2 deletion syndrome: a volumetric and voxel-based morphometry MRI study. Brain. 2006;129:1218–28.
Schmitt JE, Vandekar S, Yi J, Calkins ME, Ruparel K, Roalf DR, et al. Aberrant cortical morphometry in the 22q11.2 deletion syndrome. Biol Psychiatry. 2015;78:135–43.
Jalbrzikowski M, Jonas R, Senturk D, Patel A, Chow C, Green MF, et al. Structural abnormalities in cortical volume, thickness, and surface area in 22q11.2 microdeletion syndrome: relationship with psychotic symptoms. Neuroimage-Clin. 2013;3:405–15.
Bearden CE, van Erp TGM, Dutton RA, Tran H, Zimmermann L, Sun DQ, et al. Mapping cortical thickness in children with 22q11.2 deletions. Cereb Cortex. 2007;17:1889–98.
Sun D, Ching CRK, Lin A, Forsyth JK, Kushan L, Vajdi A, et al. Large-scale mapping of cortical alterations in 22q11.2 deletion syndrome: Convergence with idiopathic psychosis and effects of deletion size. Mol Psychiatry. 2020;25:1822–34.
Ching CRK, Gutman BA, Sun D, Villalon Reina J, Ragothaman A, Isaev D, et al. Mapping subcortical brain alterations in 22q11.2 deletion syndrome: effects of deletion size and convergence with idiopathic neuropsychiatric illness. Am J Psychiatry. 2020;177:589–600.
Villalon-Reina JE, Martinez K, Qu X, Ching CRK, Nir TM, Kothapalli D, et al. Altered white matter microstructure in 22q11.2 deletion syndrome: a multisite diffusion tensor imaging study. Mol Psychiatry. 2020;25:2818–31.
Ryali S, Supekar K, Chen T, Kochalka J, Cai W, Nicholas J, et al. Temporal dynamics and developmental maturation of salience, default and central-executive network interactions revealed by variational bayes hidden markov modeling. PLoS Comput Biol. 2016;12:e1005138.
Taghia J, Cai W, Ryali S, Kochalka J, Nicholas J, Chen T, et al. Uncovering hidden brain state dynamics that regulate performance and decision-making during cognition. Nat Commun. 2018;9:2505.
Ryali S, Supekar K, Chen T, Menon V. Multivariate dynamical systems models for estimating causal interactions in fMRI. Neuroimage. 2011;54:807–23.
Calhoun VD, Miller R, Pearlson G, Adali T. The chronnectome: time-varying connectivity networks as the next frontier in fMRI data discovery. Neuron. 2014;84:262–74.
Supekar K, Ryali S, Yuan R, Kumar D, de Los Angeles C, Menon V. Robust, generalizable, and interpretable artificial intelligence-derived brain fingerprints of autism and social communication symptom severity. Biol Psychiatry. 2022;92:643–53.
Supekar K, de Los Angeles C, Ryali S, Cao K, Ma T, Menon V. Deep learning identifies robust gender differences in functional brain organization and their dissociable links to clinical symptoms in autism. Br J Psychiatry. 2022;220:202–9.
Durstewitz D, Koppe G, Meyer-Lindenberg A. Deep neural networks in psychiatry. Mol Psychiatry. 2019;24:1583–98.
Axiomatic attribution for deep networks. Proceedings of the proceedings of the 34th international conference on machine learning-Volume 702017. JMLR. org.
Jalbrzikowski M. Neuroimaging phenotypes associated with risk and resilience for psychosis and autism spectrum disorders in 22q11.2 microdeletion syndrome. Biol Psychiatry Cogn Neurosci Neuroimaging. 2021;6:211–24.
Menon V, Palaniyappan L, Supekar K. Integrative brain network and salience models of psychopathology and cognitive dysfunction in schizophrenia. Biol Psychiatry. 2023;94:108–20.
Bassett AS, Chow EW, AbdelMalik P, Gheorghiu M, Husted J, Weksberg R. The schizophrenia phenotype in 22q11 deletion syndrome. Am J Psychiatry. 2003;160:1580–6.
Armando M, Girardi P, Vicari S, Menghini D, Digilio MC, Pontillo M, et al. Adolescents at ultra-high risk for psychosis with and without 22q11 deletion syndrome: a comparison of prodromal psychotic symptoms and general functioning. Schizophr Res. 2012;139:151–6.
Richards C, Jones C, Groves L, Moss J, Oliver C. Prevalence of autism spectrum disorder phenomenology in genetic disorders: a systematic review and meta-analysis. Lancet Psychiatry. 2015;2:909–16.
Tang KL, Antshel KM, Fremont WP, Kates WR. Behavioral and psychiatric phenotypes in 22q11.2 deletion syndrome. J Dev Behav Pediatr. 2015;36:639–50.
Button KS, Ioannidis JP, Mokrysz C, Nosek BA, Flint J, Robinson ES, et al. Power failure: why small sample size undermines the reliability of neuroscience. Nat Rev Neurosci. 2013;14:365–76.
Schmitt JE. Dynamic functional connectivity: a new perspective on 22q11.2 deletion syndrome and psychosis. Biol Psychiatry Cogn Neurosci Neuroimaging. 2019;4:852–3.
Seeley WW, Menon V, Schatzberg AF, Keller J, Glover GH, Kenna H, et al. Dissociable intrinsic connectivity networks for salience processing and executive control. J Neurosci. 2007;27:2349–56.
Haber SN, Knutson B. The reward circuit: linking primate anatomy and human imaging. Neuropsychopharmacology. 2010;35:4–26.
Hikida T, Morita M, Macpherson T. Neural mechanisms of the nucleus accumbens circuit in reward and aversive learning. Neurosci Res. 2016;108:1–5.
Abraham AD, Neve KA, Lattal KM. Dopamine and extinction: a convergence of theory with fear and reward circuitry. Neurobiol Learn Mem. 2014;108:65–77.
Lenz JD, Lobo MK. Optogenetic insights into striatal function and behavior. Behav Brain Res. 2013;255:44–54.
Lammel S, Lim BK, Malenka RC. Reward and aversion in a heterogeneous midbrain dopamine system. Neuropharmacology. 2014;76:351–9.
Berridge KC, Kringelbach ML. Pleasure systems in the brain. Neuron. 2015;86:646–64.
Krotewicz M, Romaniuk A. Social interactions, brain monoamines, and GABA alterations in MFB-lesioned cats. Pharm Biochem Behav. 1998;60:533–8.
Boylan CB, Blue ME, Hohmann CF. Modeling early cortical serotonergic deficits in autism. Behav Brain Res. 2007;176:94–108.
Ungerleider LG, Haxby JV. ‘What’ and ‘where’ in the human brain. Curr Opin Neurobiol. 1994;4:157–65.
Bearden CE, Woodin MF, Wang PP, Moss E, McDonald-McGinn D, Zackai E, et al. The neurocognitive phenotype of the 22q11.2 deletion syndrome: selective deficit in visual-spatial memory. J Clin Exp Neuropsychol. 2001;23:447–64.
Simon TJ, Bearden CE, Mc-Ginn DM, Zackai E. Visuospatial and numerical cognitive deficits in children with chromosome 22q11.2 deletion syndrome. Cortex. 2005;41:145–55.
Simon TJ, Wu Z, Avants B, Zhang H, Gee JC, Stebbins GT. Atypical cortical connectivity and visuospatial cognitive impairments are related in children with chromosome 22q11.2 deletion syndrome. Behav Brain Funct. 2008;4:25.
Kikinis Z, Makris N, Finn CT, Bouix S, Lucia D, Coleman MJ, et al. Genetic contributions to changes of fiber tracts of ventral visual stream in 22q11.2 deletion syndrome. Brain Imaging Behav. 2013;7:316–25.
Padula MC, Schaer M, Scariati E, Maeder J, Schneider M, Eliez S. Multimodal investigation of triple network connectivity in patients with 22q11DS and association with executive functions. Hum Brain Mapp. 2017;38:2177–89.
Sridharan D, Levitin DJ, Menon V. A critical role for the right fronto-insular cortex in switching between central-executive and default-mode networks. Proc Natl Acad Sci USA. 2008;105:12569–74.
Palaniyappan L, Liddle PF. Does the salience network play a cardinal role in psychosis? An emerging hypothesis of insular dysfunction. J Psychiatry Neurosci. 2012;37:17–27.
Menon V. Large-scale brain networks and psychopathology: a unifying triple network model. Trends Cogn Sci. 2011;15:483–506.
Supekar K, Cai W, Krishnadas R, Palaniyappan L, Menon V. Dysregulated brain dynamics in a triple-network saliency model of schizophrenia and its relation to psychosis. Biol Psychiatry. 2019;85:60–9.
Kapur S. Psychosis as a state of aberrant salience: a framework linking biology, phenomenology, and pharmacology in schizophrenia. Am J Psychiatry. 2003;160:13–23.
Winton-Brown TT, Fusar-Poli P, Ungless MA, Howes OD. Dopaminergic basis of salience dysregulation in psychosis. Trends Neurosci. 2014;37:85–94.
Roiser JP, Howes OD, Chaddock CA, Joyce EM, McGuire P. Neural and behavioral correlates of aberrant salience in individuals at risk for psychosis. Schizophr Bull. 2013;39:1328–36.
Howes OD, Hird EJ, Adams RA, Corlett PR, McGuire P. Aberrant salience, information processing, and dopaminergic signaling in people at clinical high risk for psychosis. Biol psychiatry. 2020;88:304–14.
McCutcheon RA, Krystal JH, Howes OD. Dopamine and glutamate in schizophrenia: biology, symptoms and treatment. World Psychiatry. 2020;19:15–33.
McCutcheon RA, Abi-Dargham A, Howes OD. Schizophrenia, dopamine and the striatum: from biology to symptoms. Trends Neurosci. 2019;42:205–20.
Howes OD, Montgomery AJ, Asselin M-C, Murray RM, Valli I, Tabraham P, et al. Elevated striatal dopamine function linked to prodromal signs of schizophrenia. Arch Gen Psychiatry. 2009;66:13–20.
Kegeles LS, Abi-Dargham A, Frankle WG, Gil R, Cooper TB, Slifstein M, et al. Increased synaptic dopamine function in associative regions of the striatum in schizophrenia. Arch Gen psychiatry. 2010;67:231–9.
Rogdaki M, Devroye C, Ciampoli M, Veronese M, Ashok AH, McCutcheon RA, et al. Striatal dopaminergic alterations in individuals with copy number variants at the 22q11. 2 genetic locus and their implications for psychosis risk: a [18F]-DOPA PET study. Mol Psychiatry. 2023;28:1995–2006.
McCutcheon RA, Nour MM, Dahoun T, Jauhar S, Pepper F, Expert P, et al. Mesolimbic dopamine function is related to salience network connectivity: an integrative positron emission tomography and magnetic resonance study. Biol Psychiatry. 2019;85:368–78.
Cheon EJ, Bearden CE, Sun D, Ching CRK, Andreassen OA, Schmaal L, et al. Cross disorder comparisons of brain structure in schizophrenia, bipolar disorder, major depressive disorder, and 22q11.2 deletion syndrome: a review of ENIGMA findings. Psychiatry Clin Neurosci. 2022;76:140–61.
Chow EW, Ho A, Wei C, Voormolen EH, Crawley AP, Bassett AS. Association of schizophrenia in 22q11.2 deletion syndrome and gray matter volumetric deficits in the superior temporal gyrus. Am J Psychiatry. 2011;168:522–9.
van Amelsvoort T, Daly E, Henry J, Robertson D, Ng V, Owen M, et al. Brain anatomy in adults with velocardiofacial syndrome with and without schizophrenia: preliminary results of a structural magnetic resonance imaging study. Arch Gen Psychiatry. 2004;61:1085–96.
Bakker G, Caan MWA, Vingerhoets WAM, da Silva-Alves F, de Koning M, Boot E, et al. Cortical morphology differences in subjects at increased vulnerability for developing a psychotic disorder: a comparison between subjects with ultra-high risk and 22q11.2 deletion syndrome. Plos One. 2016;11:e0159928.
Miller TJ, McGlashan TH, Rosen JL, Cadenhead K, Ventura J, McFarlane W, et al. Prodromal assessment with the structured interview for prodromal syndromes and the scale of prodromal symptoms: predictive validity, interrater reliability, and training to reliability. Schizophr Bull. 2003;29:703–15.
Kay SR, Fiszbein A, Opler LA. The positive and negative syndrome scale (PANSS) for schizophrenia. Schizophr Bull. 1987;13:261–76.
Abrams DA, Padmanabhan A, Chen T, Odriozola P, Baker AE, Kochalka J, et al. Impaired voice processing in reward and salience circuits predicts social communication in children with autism. Elife. 2019;8:e39906.
Abrams DA, Lynch CJ, Cheng KM, Phillips J, Supekar K, Ryali S, et al. Underconnectivity between voice-selective cortex and reward circuitry in children with autism. Proc Natl Acad Sci USA. 2013;110:12060–5.
Lynch CJ, Uddin LQ, Supekar K, Khouzam A, Phillips J, Menon V. Default mode network in childhood autism: posteromedial cortex heterogeneity and relationship with social deficits. Biol psychiatry. 2013;74:212–9.
Davatzikos C. Machine learning in neuroimaging: progress and challenges. Neuroimage. 2019;197:652–6.
Kingma DP, Ba J Adam: A method for stochastic optimization. Proceedings of the 3rd international conference on learning representations (ICLR) 1412. (2015).
van den Heuvel MP, Hulshoff Pol HE. Exploring the brain network: a review on resting-state fMRI functional connectivity. Eur Neuropsychopharmacol. 2010;20:519–34.
Zang YF, He Y, Zhu CZ, Cao QJ, Sui MQ, Liang M, et al. Altered baseline brain activity in children with ADHD revealed by resting-state functional MRI. Brain Dev. 2007;29:83–91.
Allen EA, Damaraju E, Plis SM, Erhardt EB, Eichele T, Calhoun VD. Tracking whole-brain connectivity dynamics in the resting state. Cereb Cortex. 2014;24:663–76.
Author information
Authors and Affiliations
Contributions
KS designed and conceptualized the study, contributed methods, performed investigation, wrote the original draft of the manuscript and reviewed and edited it. CDLA helped in data analysis. SR contributed to the development of the deep neural network model. LK, CS, GR, NC, and TS supervised and contributed to data collection. CB supervised data collection and reviewed and edited the manuscript. VM designed and conceptualized the study, and reviewed and edited the manuscript.
Corresponding authors
Ethics declarations
Competing interests
The authors declare no competing interests.
Additional information
Publisher’s note Springer Nature remains neutral with regard to jurisdictional claims in published maps and institutional affiliations.
Supplementary information
Rights and permissions
Springer Nature or its licensor (e.g. a society or other partner) holds exclusive rights to this article under a publishing agreement with the author(s) or other rightsholder(s); author self-archiving of the accepted manuscript version of this article is solely governed by the terms of such publishing agreement and applicable law.
About this article
Cite this article
Supekar, K., de los Angeles, C., Ryali, S. et al. Robust and replicable functional brain signatures of 22q11.2 deletion syndrome and associated psychosis: a deep neural network-based multi-cohort study. Mol Psychiatry (2024). https://doi.org/10.1038/s41380-024-02495-8
Received:
Revised:
Accepted:
Published:
DOI: https://doi.org/10.1038/s41380-024-02495-8