Abstract
Hydroxyproline (Hyp) O-arabinosylation is a post-translational modification that is prominent in extracellular glycoproteins in plants. Hyp O-arabinosylation is generally found in these glycoproteins in the form of linear oligoarabinoside chains and has a key role in their function by contributing to conformational stability. However, Hyp O-arabinosyltransferase (HPAT), a key enzyme that catalyzes the transfer of the L-arabinose to the hydroxyl group of Hyp residues, has remained undiscovered. Here, we purified and identified Arabidopsis HPAT as a Golgi-localized transmembrane protein that is structurally similar to the glycosyltransferase GT8 family. Loss-of-function mutations in HPAT-encoding genes cause pleiotropic phenotypes that include enhanced hypocotyl elongation, defects in cell wall thickening, early flowering, early senescence and impaired pollen tube growth. Our results indicate essential roles of Hyp O-arabinosylation in both vegetative and reproductive growth in plants.
This is a preview of subscription content, access via your institution
Access options
Subscribe to this journal
Receive 12 print issues and online access
$259.00 per year
only $21.58 per issue
Buy this article
- Purchase on Springer Link
- Instant access to full article PDF
Prices may be subject to local taxes which are calculated during checkout




Similar content being viewed by others
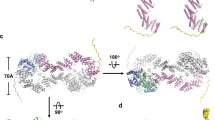
References
Lamport, D.T.A. Hydroxyproline-O-glycosidic linkage of the plant cell wall glycoprotein extensin. Nature 216, 1322–1324 (1967).
Kieliszewski, M.J., Lamport, D.T., Tan, L. & Cannon, M.C. Hydroxyproline-rich glycoproteins: form and function. Annu. Plant Rev. 41, 321–342 (2011).
Kieliszewski, M.J. & Lamport, D.T. Extensin: repetitive motifs, functional sites, post-translational codes, and phylogeny. Plant J. 5, 157–172 (1994).
Kieliszewski, M., de Zacks, R., Leykam, J.F. & Lamport, D.T. A repetitive proline-rich protein from the gymnosperm douglas fir is a hydroxyproline-rich glycoprotein. Plant Physiol. 98, 919–926 (1992).
Ellis, M., Egelund, J., Schultz, C.J. & Bacic, A. Arabinogalactan-proteins: key regulators at the cell surface? Plant Physiol. 153, 403–419 (2010).
Lamport, D.T. & Miller, D.H. Hydroxyproline arabinosides in the plant kingdom. Plant Physiol. 48, 454–456 (1971).
Showalter, A.M. Structure and function of plant cell wall proteins. Plant Cell 5, 9–23 (1993).
Akiyama, Y., Mori, M. & Kato, K. 13C-NMR analysis of hydroxyproline arabinosides from Nicotiana tabacum. Agric. Biol. Chem. 44, 2487–2489 (1980).
van Holst, G.J. & Varner, J.E. Reinforced polyproline II conformation in a hydroxyproline-rich cell wall glycoprotein from carrot root. Plant Physiol. 74, 247–251 (1984).
Ohyama, K., Shinohara, H., Ogawa-Ohnishi, M. & Matsubayashi, Y. A glycopeptide regulating stem cell fate in Arabidopsis thaliana. Nat. Chem. Biol. 5, 578–580 (2009).
Amano, Y., Tsubouchi, H., Shinohara, H., Ogawa, M. & Matsubayashi, Y. Tyrosine-sulfated glycopeptide involved in cellular proliferation and expansion in Arabidopsis. Proc. Natl. Acad. Sci. USA 104, 18333–18338 (2007).
Pearce, G., Moura, D.S., Stratmann, J. & Ryan, C.A. Production of multiple plant hormones from a single polyprotein precursor. Nature 411, 817–820 (2001).
Shinohara, H. & Matsubayashi, Y. Chemical synthesis of Arabidopsis CLV3 glycopeptide reveals the impact of hydroxyproline arabinosylation on peptide conformation and activity. Plant Cell Physiol. 54, 369–374 (2013).
Owens, R.J. & Northcote, D.H. The location of arabinosyl:hydroxyproline transferase in the membrane system of potato tissue culture cells. Biochem. J. 195, 661–667 (1981).
Shpak, E., Leykam, J.F. & Kieliszewski, M.J. Synthetic genes for glycoprotein design and the elucidation of hydroxyproline-O-glycosylation codes. Proc. Natl. Acad. Sci. USA 96, 14736–14741 (1999).
Lairson, L.L., Henrissat, B., Davies, G.J. & Withers, S.G. Glycosyltransferases: structures, functions, and mechanisms. Annu. Rev. Biochem. 77, 521–555 (2008).
Nikolovski, N. et al. Putative glycosyltransferases and other plant Golgi apparatus proteins are revealed by LOPIT proteomics. Plant Physiol. 160, 1037–1051 (2012).
Basu, D. et al. Functional identification of a hydroxyproline-O-galactosyltransferase specific for arabinogalactan protein biosynthesis in Arabidopsis. J. Biol. Chem. 288, 10132–10143 (2013).
Schnabel, E.L. et al. The ROOT DETERMINED NODULATION1 gene regulates nodule number in roots of Medicago truncatula and defines a highly conserved, uncharacterized plant gene family. Plant Physiol. 157, 328–340 (2011).
Okamoto, S., Shinohara, H., Mori, T., Matsubayashi, Y. & Kawaguchi, M. Root-derived CLE glycopeptides control nodulation by direct binding to HAR1 receptor kinase. Nat. Commun. 4, 2191 (2013).
Uemura, T. et al. Systematic analysis of SNARE molecules in Arabidopsis: dissection of the post-Golgi network in plant cells. Cell Struct. Funct. 29, 49–65 (2004).
Singer, T. et al. A high-resolution map of Arabidopsis recombinant inbred lines by whole-genome exon array hybridization. PLoS Genet. 2, e144 (2006).
Taylor, L.P. & Hepler, P.K. Pollen germination and tube growth. Annu. Rev. Plant Physiol. Plant Mol. Biol. 48, 461–491 (1997).
Cannon, M.C. et al. Self-assembly of the plant cell wall requires an extensin scaffold. Proc. Natl. Acad. Sci. USA 105, 2226–2231 (2008).
Stafstrom, J.P. & Staehelin, L.A. The role of carbohydrate in maintaining extensin in an extended conformation. Plant Physiol. 81, 242–246 (1986).
Ohyama, K., Ogawa, M. & Matsubayashi, Y. Identification of a biologically active, small, secreted peptide in Arabidopsis by in silico gene screening, followed by LC-MS–based structure analysis. Plant J. 55, 152–160 (2008).
Hall, Q. & Cannon, M.C. The cell wall hydroxyproline-rich glycoprotein RSH is essential for normal embryo development in Arabidopsis. Plant Cell 14, 1161–1172 (2002).
Okamoto, S. et al. Nod factor/nitrate-induced CLE genes that drive HAR1-mediated systemic regulation of nodulation. Plant Cell Physiol. 50, 67–77 (2009).
Reid, D.E., Ferguson, B.J. & Gresshoff, P.M. Inoculation- and nitrate-induced CLE peptides of soybean control NARK-dependent nodule formation. Mol. Plant Microbe Interact. 24, 606–618 (2011).
Mortier, V. et al. CLE peptides control Medicago truncatula nodulation locally and systemically. Plant Physiol. 153, 222–237 (2010).
Lamport, D.T., Kieliszewski, M.J., Chen, Y. & Cannon, M.C. Role of the extensin superfamily in primary cell wall architecture. Plant Physiol. 156, 11–19 (2011).
Gille, S., Hansel, U., Ziemann, M. & Pauly, M. Identification of plant cell wall mutants by means of a forward chemical genetic approach using hydrolases. Proc. Natl. Acad. Sci. USA 106, 14699–14704 (2009).
Egelund, J. et al. Molecular characterization of two Arabidopsis thaliana glycosyltransferase mutants, rra1 and rra2, which have a reduced residual arabinose content in a polymer tightly associated with the cellulosic wall residue. Plant Mol. Biol. 64, 439–451 (2007).
Velasquez, S.M. et al. O-glycosylated cell wall proteins are essential in root hair growth. Science 332, 1401–1403 (2011).
Matsubayashi, Y. Post-translational modifications in secreted peptide hormones in plants. Plant Cell Physiol. 52, 5–13 (2011).
Abel, S. & Theologis, A. Transient transformation of Arabidopsis leaf protoplasts: a versatile experimental system to study gene expression. Plant J. 5, 421–427 (1994).
Moran, R. Formulae for determination of chlorophyllous pigments extracted with N,N-dimethylformamide. Plant Physiol. 69, 1376–1381 (1982).
Boavida, L.C. & McCormick, S. Temperature as a determinant factor for increased and reproducible in vitro pollen germination in Arabidopsis thaliana. Plant J. 52, 570–582 (2007).
Muschietti, J., Dircks, L., Vancanneyt, G. & McCormick, S. LAT52 protein is essential for tomato pollen development: pollen expressing antisense LAT52 RNA hydrates and germinates abnormally and cannot achieve fertilization. Plant J. 6, 321–338 (1994).
Schnabelrauch, L.S., Kieliszewski, M., Upham, B.L., Alizedeh, H. & Lamport, D.T. Isolation of pl 4.6 extensin peroxidase from tomato cell suspension cultures and identification of Val-Tyr-Lys as putative intermolecular cross-link site. Plant J. 9, 477–489 (1996).
Acknowledgements
We thank the National Institute for Basic Biology Functional Genomics Facility for MALDI-TOF MS analysis and T. Ueda (University of Tokyo) for providing the mRFP-SYP31 expression vector. This research was supported by the Funding Program for Next Generation World-Leading Researchers from the Japan Society for the Promotion of Science (no. GS025) and Grants-in-Aid for Scientific Research (S) from the Ministry of Education, Culture, Sports, Science and Technology (no. 25221105).
Author information
Authors and Affiliations
Contributions
M.O.-O. and Y.M. designed experiments. M.O.-O., W.M. and Y.M. performed and analyzed experiments. M.O.-O. and Y.M. wrote the manuscript. Y.M. supervised the project.
Corresponding author
Ethics declarations
Competing interests
The authors declare no competing financial interests.
Supplementary information
Supplementary Text and Figures
Supplementary Results, Supplementary Tables 1–3 and Supplementary Figures 1–10. (PDF 8342 kb)
Rights and permissions
About this article
Cite this article
Ogawa-Ohnishi, M., Matsushita, W. & Matsubayashi, Y. Identification of three hydroxyproline O-arabinosyltransferases in Arabidopsis thaliana. Nat Chem Biol 9, 726–730 (2013). https://doi.org/10.1038/nchembio.1351
Received:
Accepted:
Published:
Issue Date:
DOI: https://doi.org/10.1038/nchembio.1351
This article is cited by
-
The hydroxyproline O-arabinosyltransferase FIN4 is required for tomato pollen intine development
Plant Reproduction (2023)
-
Mobile Signaling Peptides: Secret Molecular Messengers with a Mighty Role in Plant Life
Journal of Plant Growth Regulation (2023)
-
CLE peptides: critical regulators for stem cell maintenance in plants
Planta (2022)
-
Advances in Fe(II)/2-ketoglutarate-dependent dioxygenase-mediated C–H bond oxidation for regioselective and stereoselective hydroxyl amino acid synthesis: from structural insights into practical applications
Systems Microbiology and Biomanufacturing (2021)
-
Pathway-specific enzymes from bamboo and crop leaves biosynthesize anti-nociceptive C-glycosylated flavones
Communications Biology (2020)