« Prev Next »



Non-Invasive Genetic Analysis
The relatively recent development of non-invasive genetics has allowed primatologists to better understand the population and group dynamics of wild primates, simply by the fact that it is now possible to obtain genetic information by extracting DNA from by-products such as feces, shed hair, and urine. The first study of this kind was in chimpanzees (Pan troglodytes) in which Morin et al. (1993) analyzed patterns of gene flow in the Gombe chimpanzee community (Tanzania). Since then, major technical improvements in non-invasive genetics have greatly expanded our capacity to address a wide range of questions about the structure of primate populations, their evolutionary histories, and adaptation, while allowing the study of wild populations without direct contact with the animals (Charpentier et al. 2007, Tung et al. 2008). More importantly, the combination of genetics with long-term socio-ecological data has enabled comprehensive analysis at an individual and social group level for a wide range of primate species.
DNA obtained non-invasively can be analyzed in individuals and populations for a wide range of molecular genetic markers such as microsatellites, minisatellites, mitochondrial DNA, amplified fragment length polymorphism (AFLP) and the major histocompatibility complex (MHC). By using a variety of software to analyze the genetic data produced, primatologists can now obtain information on effective population size, parentage, relatedness, sex, dispersal, population structure, population assignment and gene flow. This is crucial if we are to fully understand population dynamics at a local scale and evaluate the threats and suggest appropriate conservation measures (Goossens & Bruford 2009).
Threats to Primate Conservation
Habitat fragmentation
Habitat fragmentation can influence several key features of primate populations: 1) their diet, 2) the social group size or density, and 3) the dispersal and gene flow between social groups or subpopulations (Marsh 2003, Frankham et al. 2002). Consequently, the capacity for populations to persist in fragmented landscapes is related to a species' particular characteristics (Marsh 2003) (Figure 2). It is theoretically possible for primate populations to increase in size within fragments-for example, if natural predators disappear (Strier 2007)-but more frequently, they decrease or become extinct. This can be due either to direct mortality, caused by an increased hunting pressure, since isolated areas become accessible for humans (Marsh 2003, Strier 2007), or due to genetic changes (Frankham et al. 2002) (Figure 3). In the long term, fragmentation can lead to a reduction in genetic diversity and increased genetic differentiation. This results from the decrease of gene flow between breeding groups and the action of random genetic drift and/or inbreeding (Frankham et al. 2002).
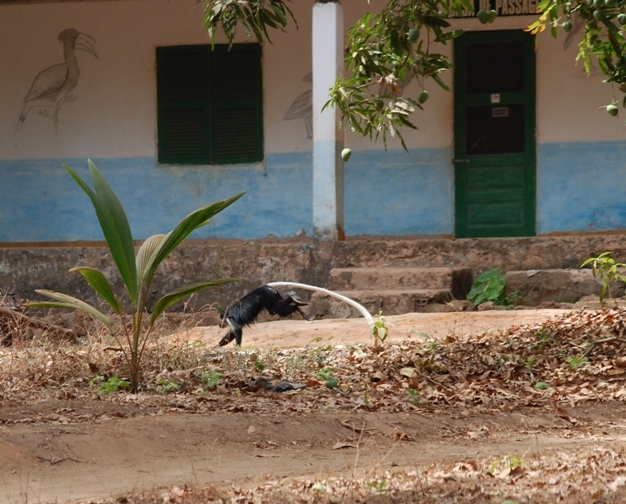

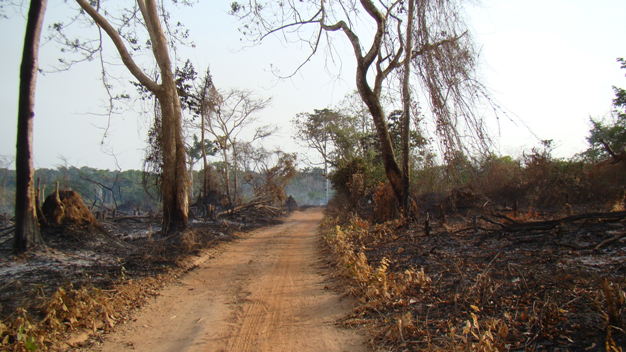

Each population fragment may show different levels of genetic diversity and significantly different allele frequencies from the other fragments. The risk of inbreeding depression is increased if the population is smaller and isolated, with lower genetic diversity. Migration of individuals between fragments and subsequent reproduction will introduce new alleles into the population (increasing genetic diversity) and it will counterbalance the effects of genetic drift and inbreeding, preventing complete fixation of alleles (Frankham et al. 2002). By using non-invasive genetic methods it is possible to identify the genetic structure of a fragmented population, and levels of gene flow between units, and determine whether ecological corridors should be created/maintained, or individuals should be translocated (e.g., Bruford et al. 2010).
In the fragmented range of the Cross River Gorilla (Gorilla gorilla diehli) three sub-populations have been uncovered using microsatellite markers (Bergl & Vigilant 2007). Although this genetic structure corresponds broadly to the pattern of habitat fragmentation, migrants between fragments could be identified. Since different levels of genetic diversity were found between the sub-populations, it was suggested that the conservation of the most genetically diverse sub-population should be prioritized. Also, habitat corridors between fragments, along with measures to control hunting in areas between fragments, were recommended (Bergl et al. 2008). The Bornean orang-utan (Pongo pymaeus), living in forest fragments of the Lower Kinabatangan flood plain in Sabah, Malaysia, shows a different pattern: high levels of heterozygosity within fragments, with a relative scarcity of rare alleles, suggesting that this population was large in the past and has suffered a recent major reduction (Goossens et al. 2005). Goossens et al. (2006), using extensive non-invasive sampling across the area and 14 microsatellite loci, showed that the Bornean orang-utans population has decreased in size by 95% over the last decades or centuries, due to anthropogenetic fragmentation of the habitat. Therefore, the high genetic diversity found is transitory and may disappear if forest corridors alongside the riverbank are not established (Bruford et al. 2010).
Hunting
The impact of hunting pressure on primate populations is often difficult to evaluate. Although information on the amount of harvested primates can be obtained by counting carcasses in urban bushmeat markets, morphological identification can be hindered if a carcass has been processed, or if the meat has been smoked (Figure 4).


Primatologists can use molecular PCR-based tools to taxonomically identify unknown specimens. After extracting DNA and amplifying a specific DNA fragment, these fragments can then be compared with other DNA fragments obtained from specimens of known species. The comparison can also be accomplished by verifying the presence/size of the fragment after PCR: for a review of the techniques see Fajardo et al. (2010).
In many cases however, the researcher might not have access to specimens of known species. To overcome this difficulty, it is necessary to amplify a standard gene fragment that can be compared with fragments from voucher species deposited in public databases. A fragment of 648bp from the mitochondrial cytochrome c oxidase (COI) gene was proposed by Hebert et al. (2003) as a standard fragment for DNA barcoding, the data for which are deposited in the Barcode of Life Database (http://www.barcodeoflife.org/) as well as in public databases such as GenBank. Lorenz et al. (2005) tested the use of this mitochondrial DNA region to identify the species of primate samples. All samples, representing 56 primate species, amplified with at least one of the 3 different primers used and, with few exceptions, the fragments obtained clustered together with sequences retrieved from GenBank (Figure 5). More recently, Rönn et al. (2009) proposed the use of a micro-array system to assign samples of primates to the genus level, using both nuclear and mitochondrial genes. This technique uses 111 diagnostic nucleotide positions to perform a hierarchical assignment of samples. This method can be used to process a large number of samples at a relatively low cost, and 45 out of the 64 samples were correctly assigned to their Primates genus.


Diseases
Disease is another important aspect for primate conservation. The Ebola and anthrax outbreaks that have occurred in Central Africa in recent decades caused a dramatic decline in gorilla and chimpanzee populations (Leendertz et al. 2006, Bermejo et al. 2007, Campbell et al. 2008). Additionally, recent studies on parasite infection dynamics have demonstrated an association with hunting, human population growth, and fragmentation in wild primates (Gillespie & Chapman 2006, Goldberg et al. 2007, Gillespie et al. 2008, Riley & Fuentes 2011). With the incorporation of molecular approaches to epidemiology, Johnston et al. (2010) have demonstrated cross species transmission of Giardia duodenalis between humans, livestock, and wild primates in Western Uganda. Likewise, Goldberg et al. (2009) discovered three novel retroviruses in red colobus monkeys, shedding light on the dynamics of primate retroviral transmission. More recently, Yildirim et al. (2010) unveiled the gut microbial community of three nonhuman primate species by sequencing the small subunit rRNA unit from fecal samples, allowing future analysis on comparative and evolutionary studies of human gut microbes and other primates. Furthermore, using an innovative method that combines a single-genome amplification of Plasmodium sp. recovered non-invasively from fecal material of great apes, Liu et al. (2010) inferred that the origin of the human malignant malaria Plasmodium falciparum is gorilla-derived. This result argues against the previous study from Prugnolle et al. (2009) that showed that P. falciparum emerged from P. reichenowi by a single transfer from chimpanzees. Similarly, HIV/AIDS is the result of a cross-species transmission event of simian immunodeficiency virus (SIV) to humans from non-human African primates, and much attention has been paid to the understanding of the evolutionary history of these emerging infection diseases (Gao et al. 1999, Damond et al. 2004, Liu et al. 2008). By using a molecular dating technique, Wertheim and Worobey (2009) estimated a surprisingly recent common ancestor of infectious SIV in chimpanzees (between 1266 to 1685 years ago) and sooty mangabeys (between 1729 to 1875 years ago), the reservoirs of HIV-1 and HIV-2, respectively. Conversely, human transmitted pathogens to great apes such as bacteria (e.g., Streptococcus pneumoniae) or viruses (e.g., human metapneumovirus) are causing fatal respiratory outbreaks (Chi et al. 2007, Kaur et al. 2008, Köndgen et al. 2008, 2011, Palacios et al. 2011) and to mitigate the risk of disease transmission the use of face masks by researchers, tourists and staff is advocated as a good practice (Macfie & Williamson 2010). These studies emphasize the fact that there is much to be learned concerning disease transmission and its implications for wild primates using molecular tools.
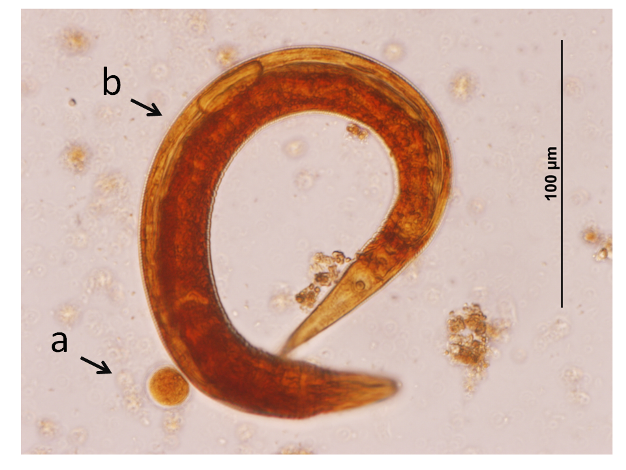

Applying Conservation Genetics
Primate census
"Evolutionary significant units" (ESUs) and "management units" (MUs)
Population and habitat viability analysis
Population and habitat viability analysis (PHVA) evaluates the risk of extinction within a certain period of time (e.g., 100 or 200 years) and identifies which factors play a major role in the extinction process. PHVA relies on stochastic modeling by using simulation software, such as VORTEX (Miller & Lacy 2005), and requires the input of parameters on the ecology and life history of the species (e.g., population size, mortality and birth rates, sex ratio, dispersal rates, and main threats to the habitat) to be able to simulate (by Monte Carlo iterations) species responses that are realistic. Molecular census and genetic data can also be very important parameters for PHVA. Moreover, it allows the introduction of different and combined management measures (e.g., ecological corridors, reintroduction, translocations, habitat rehabilitation) and simulates the evolution of the species under such interventions. This tool allows conservationists to detect the major threats for rare and endangered species and thereby help implement the most long-term viable conservation actions.
Bruford et al. (2010) incorporated the genetic data of 200 orangutans from the Kinabatangan floodplain in Sabah, Malaysia, to study the implications of non-intervention, translocation, corridor establishment, and a combination of the latter two measures, on the future genetic diversity of this highly fragmented population. They found that non-intervention would result in the extinction of some of the subpopulations within five generations, and that translocation or corridor establishment alone would not be sufficient to prevent high levels of inbreeding. Instead, a combination of the two measures would retain the demographic stability of even the most isolated subpopulations and constrain localized inbreeding to a sustainable threshold (Figure 7).


Summary
The extensive use of molecular techniques as tools has provided new opportunities to better understand the mechanisms underlying the evolution and adaptation of primates (Figure 8). By integrating genetic and ecological data into simulation models, conservation predictions will be more accurate, and long-term conservation strategies will be more effective.


All authors have contributed equally to this article.
References and Recommended Reading
Arandjelovic M. et al. Effective non-inasive genetic monitoring of multiple wild western gorilla groups. Biological Conservation 143, 1780-1791 (2010).
Bergl, R. & Vigilant, L. Genetic analysis reveals population structure and recent migration within the highly fragmented range of the Cross River gorilla (Gorilla gorilla diehli). Molecular Ecology 16, 501-516 (2007).
Bergl, R. A. et al. Effects of habitat fragmentation, population size and demographic history on genetic diversity: The Cross River gorilla in a comparative context. American Journal of Primatology 70, 848-859 (2008).
Bermejo, M. et al. Ebola outbreak killed 5000 gorillas. Science 314, 1564 (2006).
Bruford, M. W. et al. Projecting genetic diversity and population viability for the fragmented orangutan population in the Kinabatangan floodplain, Sabah, Malaysia. Endangered Species Research 12, 249-261 (2010).
Campbell, G. et al. Alarming decline of West African chimpanzees in Côte d' Ivoire. Current Biology 18, 903-904 (2008).
Chapman, C. A. & Russo, S. E. "Primate seed dispersal: Linking behavioral ecology with forest community structure," in Primates in Perspective, eds. C. J. Campbell et al. (New York, NY: Oxford University Press, 2007) 510-525.
Charpentier, M. J. E. et al. Inbreeding depression in non-human primates: A historical review of methods used and empirical data. American Journal of Primatology 69, 1370-1386 (2007).
Chi, F. et al. New Streptococcus pneumoniae clones in deceased wild chimpanzees. Journal of Bacteriology 189, 6085-6088 (2007).
Cowlishaw, G. & Dumbar, R. Primate Conservation Biology. Chicago, IL: Chicago University Press, 2000.
Damond, F. et al. Identification of a highly divergent HIV type 2 and proposal for a change in HIV type 2 classification. AIDS Research and Human Retroviruses 20, 666-672 (2004).
Fajardo, V. et al. A review of current PCR-based methodologies for the authentication of meats from game animal species. Trends in Food Science & Technology 21, 408-421 (2010).
Frankham, R. et al. Introduction to Conservation Genetics. New York, NY: Cambridge University Press, 2002.Gao, F. et al. Origin of HIV-1 in the chimpanzee Pan troglodytes troglodytes. Nature 397, 436-441 (1999).
Gibbons, M. A. & Harcourt, A. H. Biological correlates of extinction and persistence of primates in small forest fragments: A global analysis. Tropical Conservation Science 2, 388-403 (2009).
Gillespie, T. R. & Chapman, C. A. Prediction of parasite infection dynamics in primate metapopulations based on attributes of forest fragmentation. Conservation Biology 20, 441-448 (2006).
Gillespie, T. R. et al. Integrative approaches to the study of primate infectious disease: Implications for biodiversity conservation and global health. Yearbook of Physical Anthropology 51, 53-69 (2008).
Goldberg, T. L. et al. Patterns of gastrointestinal bacterial exchange between chimpanzees and humans involved in research and tourism in western Uganda. Biological Conservation 135, 511-517 (2007).
Goldberg, T. L. et al. Co-infection of Ugandan Red Colobus (Procolobus [Piliocolobus] rufomitratus tephrosceles) with novel, divergent delta-, lenti-, and spumaretroviruses. Journal of Virology 83, 11318-11329 (2009).
Goossens, B. et al. Patterns of genetic diversity and migration in increasingly fragmented and declining orang-utan (Pongo pygmaeus) populations from Sabah, Malaysia. Molecular Ecology 14, 441-456 (2005).
Goossens, B. et al. Genetic signature of anthropogenic population collapse in orang-utans. PLoS Biology 4, e25 (2006).
Goossens, B. & Bruford, M. W. "Non-invasive genetic analysis in conservation," in Population Genetics for Animal Conservation, eds. G. Bertorelle et al. (Cambridge, UK: Cambridge University Press, 2009) 167-201.
Guschanski, K. et al. Counting elusive animals: Comparing field and genetic census of the entire mountain gorilla population of Bwindi impenetrable National Park, Uganda. Biological Conservation 142, 290-300 (2009).
Harcourt, A. H. et al. Rarity, specialization and extinction in primates. Journal of Biogeography 29, 445-456 (2002).
Harcourt, A. H. & Parks, S. A. Threatened primates experience high human densities: Adding an index of threat to the IUCN Red List criteria. Biological Conservation 109, 137-149 (2003).
Hebert, P. et al. Barcoding animal life: Cytochrome c oxidase subunit 1 divergences among closely related species. Proceedings of the Royal Society B: Biological Sciences 270 (Supplement), S96-S99 (2003).
Johnston, A. R. et al. Molecular epidemiology of cross-species Giardia duodenalis transmission in Western Uganda. PLoS Neglected Tropical Diseases 4, 683-689 (2010).
Kaur, T. et al. Descriptive epidemiology of fatal respiratory outbreaks and detection of a human-related metapneumovirus in wild chimpanzees (Pan troglodytes) at Mahale Mountains National Park, Western Tanzania. American Journal of Primatology 70, 755-765 (2008).
Köndgen, S. et al. Pandemic human viruses cause decline of endangered great apes. Current Biology 18, 260-264 (2008).
Köndgen, S. et al. Pasteurella multocida involved in respiratory diseases of wild chimpanzees. PLoS ONE 6, e24236 (2011).
Lambert, J. E. Primate seed dispersers as umbrella species: A case study from Kibale National Park, Uganda with implications for afrotropical forest conservation. American Journal of Primatology 73, 9-24 (2011).
Leendertz, F. H. et al. Pathogens as drivers of population declines: The importance of systematic monitoring in great apes and other threatened mammals. Biological Conservation 131, 325-337 (2006).
Liu, W. et al. Origin of the human malaria parasite Plasmodium falciparum in gorillas. Nature 467, 420-425 (2010).
Liu, W. et al. Molecular ecology and natural history of simian foamy virus infection in wild-living chimpanzees. PLoS Pathogens 4, e1000097 (2008).
Lorenz, J. G. et al. The problems and promise of DNA barcodes for species diagnosis of primate biomaterials. Philosophical Transactions of the Royal Society B: Biological Sciences 360, 1869-1877 (2005).
Macfie, E. & Williamson, E. Best Practice Guidelines for Great Ape Tourism. Gland, Switzerland: IUCN/SSC Primate Specialist Group, 2010.
Marsh, L. K. "The nature of fragmentation," in Primates in Fragments: Ecology and Conservation, ed. L. K. Marsh (New York, NY: Kluwer Academic/Plenum Publishers, 2003) 1-10.
Miller, P. S. & Lacy, R. C. VORTEX: A stochastic simulation of the simulation process. Version 9.50 user's manual. Apple Valley, MN: IUCN/SSC Conservation Breeding Specialist Group, 2005.
Mittermeier, R. A. et al. Primates in peril: The world's 25 most endangered primates 2008-2010. Primate Conservation 24, 1-57 (2009).
Morin, P. A. et al. Non-invasive sampling and DNA amplification for paternity exclusion, community structure and phylogeography in wild chimpanzees. Primates 34, 347-356 (1993).
Norconk, M. A. et al. Primates in 21st century ecosystems: Does primate conservation promote ecosystem conservation? American Journal of Primatology 73, 3-8 (2011).
Paetkau, D. Using genetics to identify intraspecific conservation units: A critique of current methods. Conservation Biology 13, 1507-1509 (1999).
Palacios, G. et al. Human metapneumovirus infection in wild mountain gorillas, Rwanda. Emerging Infectious Diseases 17, 711-713 (2011).
Prugnolle, F. et al. African great apes are natural hosts of multiple related malaria species, including Plasmodium falciparum. Proceedings of National Academy of Sciences of the United States of America 107, 1458-1463 (2010).
Riley, E. P. & Fuentes, A. Conserving social-ecological systems in Indonesia: Human-nonhuman primate interconnections in Bali and Sulawesi. American Journal of Primatology 73, 62-74 (2011).
Rönn, A-C. et al. First generation microarray-system for identification of primate species subject to bushmeat trade. Endangered Species Research 9, 133-142 (2009).
Storfer, A. et al. Putting the "landscape" in landscape genetics. Heredity 98, 128-142 (2007).
Strier, K. B. Primate Behavioral Ecology. Boston, MA: Pearson, 2000.
Tung, J. et al. Genetic evidence reveals temporal change in hybridization patterns in a wild baboon population. Molecular Ecology 17, 1998-2011 (2008).
Vigilant, L. & Guschanski, K. Using genetics to understand the dynamics of wild primate populations. Primates 50, 105-120 (2009).
Wertheim, J. & Worobei, M. Dating the age of the SIV lineages that gave rise to HIV-1 and HIV-2. PLoS Computational Biology 5, e1000377 (2009).
WWF (World Wide Fund for Nature). Living Planet Report 2010. WWF, 2010.Yildirim, S. et al. Characterization of the fecal microbiome from non-human wild primates reveals species specific microbial communities. PLoS ONE 5, 13963-13976 (2010).
Zhan, X. et al. Molecular censusing increases key giant panda population estimate by more than 100%. Current Biology 16, 451-452 (2006).