« Prev Next »

Most people are familiar with the hungry caterpillar in the vegetable garden or the elusive beetle in the pantry, and contending with these unwelcomed pests is a longstanding problem. Ever since humans started farming they have shared part of their harvest with insects. Growers of maize, Zea mays (corn), are challenged with a number of pests, but the most important are lepidopteran larvae (i.e., caterpillars) that are stalk borers, ear or leaf feeders, and coleopteran larvae (i.e., beetle grubs) that feed on roots. The European corn borer, Ostrinia nubilalis, for example, was nicknamed the "billion dollar bug" because it cost growers over a billion dollars annually in insecticides and lost crop yields (Figure 1). Most maize growers rely on traditional crop protection practices to manage these insects, including cultural, biological or chemical (insecticide) methods or a balance of these methods that aims to minimize environmental impact called integrated pest management (IPM) (Hellmich et al. 2008). However, in 1996 USA growers were introduced to commercial maize that was genetically engineered (GE) with resistance to European corn borer and other lepidopteran maize pests. In 2003 another GE maize was introduced that killed corn rootworm larvae (beetle grubs), especially larvae of the western corn rootworm, Diabrotica virgifera virgifera, another "billion dollar bug" (Figure 2). These GE plants produce crystal (Cry) proteins or toxins derived from the soil bacterium, Bacillus thuringiensis (Bt), hence the common name "Bt maize". Bt maize has revolutionized pest control in a number of countries, but there still are questions about its use and impact. This article will focus on the opportunities and challenges presented by Bt maize as they relate to current insect-resistant products.
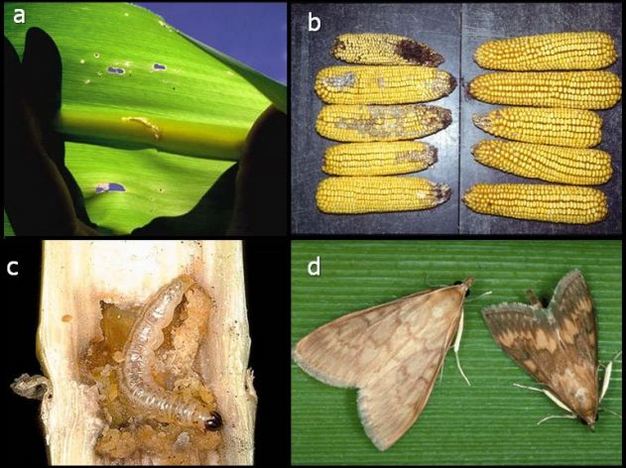

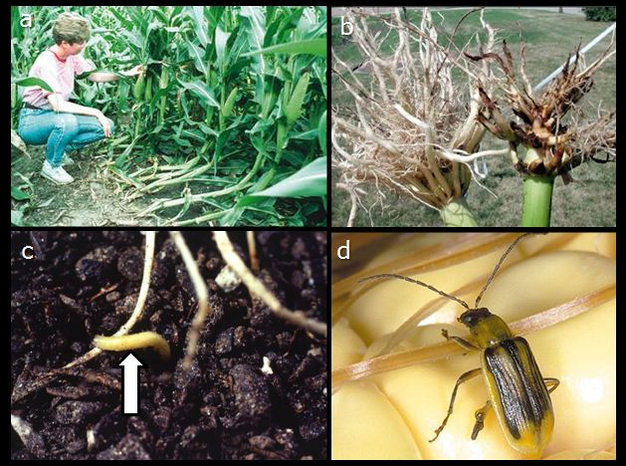

What is Bt Maize?
There are a number of Cry toxins that are categorized by their spectrum of activity. For maize pests, primary Cry proteins are Cry1 and Cry2 for Lepidoptera and Cry3 proteins for Coleoptera (Schnepf et al. 1998). Maize can be genetically engineered to produce these specific Cry toxins. As such, aspects of Bt maize are similar to host plant resistance traits such as DIMBOA (2,4-dihydroxy-7-methoxy-1,4-benzoxazin-3-one), which at high levels reduces damage by European corn borer (Klun et al. 1967). Seed providers often combine or stack traits for Lepidoptera and Coleoptera control into the same plant. Also, different types of Bt toxins targeted for the same insects are often combined into more effective plant protectants called pyramids. This multiple toxin approach is useful for managing insect resistance to Bt maize.
Why is Bt Maize Popular with Growers?
Reduced Use of Insecticides
Protected Yields
Improved Grain Quality
Why is Bt Maize Not Accepted by Some People?
Possible Effects on Non-target Organisms
Maize Gene Flow
Insect Resistance
Successful control of insects by Bt maize has many scientists concerned that overuse of Bt maize could produce pests resistant to Bt toxins. Field-evolved resistance to Bt maize has occurred for two moth species: fall armyworm, Spodoptera frugiperda, in Puerto Rico, and African stem borer, Busseola fusca, in South Africa, so this concern is warranted. Various strategies have been proposed for managing insect resistance to Bt maize, but currently the high-dose/refuge (HDR) strategy is the most commonly recommended (Bates et al. 2005, Tabashnik 1994). With this strategy, insects that feed on the Bt maize are exposed to an extremely high dose of toxin; and this is complemented with refuges, usually non-Bt maize, that provide a population of susceptible insects that are not exposed to Bt toxin (Figure 3). Consequently, rare resistant moths that develop on Bt maize, instead of mating with each other, mate with individuals among the overwhelming number of susceptible moths from the refuge (Gould 1998, Tabashnik & Croft 1982). This process essentially dilutes resistance genes and maintains a population of susceptible insects. The HDR strategy should be effective as long as plants express a high dose of the toxin, genes conferring resistance are rare, and there are many insects from the refuge available to mate randomly with resistant insects (Gould 1998). Sometimes convincing growers to plant non-Bt maize refuges is a challenge because it requires careful planning of where to plant the refuge and could reduce yields. Currently in the USA refuge recommendations range from 5–20 percent, depending on region of the country and type of Bt maize.


Pyramided maize that produces two Bt proteins with different modes of action targeted for the same insect has reduced refuges because the two-toxin "redundant killing" reduces the chances that insects will evolve resistance. A challenge with the HDR strategy is Bt maize is not high dose for many common maize pests. For example, lepidopteran Bt maize is not high dose for fall armyworm, S. frugiperda, corn earworm, Helicoverpa zea, and cutworm species (family Noctuidae), while coleopteran Bt maize is not high dose for corn rootworm (Diabrotica spp.). Both instances of field resistance, fall armyworm, S. frugiperda, in Puerto Rico, and African stem borer, B. fusca, in South Africa, involved species that were only moderately susceptible to Bt maize. But it is unclear if resistance evolution was due to lack of high dose or insufficient refuge.
Compatibility with Other Control Methods
Although Bt maize is an important tool for growers, it cannot completely replace other pest control tactics. Insecticides, for example, may be necessary to control secondary insect pests. Host plant resistance is important to maintain because if pests become resistant to Bt toxins it will be needed as a backup method of pest control. Finally, Bt maize should be especially compatible with biological control because reduced use of insecticides should lead to an increase in beneficial insects (Naranjo 2009). In general, traditional pest management practices must be maintained in order to avoid reliance on a single tactic.
Glossary
Active ingredient: Substance in a pesticide that is biologically active.
Biological control: Control of a pest by the introduction of a natural enemy (predator, parasite or pathogen).
Coleoptera: The insect order comprising beetles.
Crystal (Cry) proteins: Proteinacous inclusions produced by many strains of Bacillus thuringiensis during spore formation that have insecticidal activity.
Cultural control: Management techniques used in agricultural to reduce pest populations, such as crop rotation.
Deoxynivalenol: Mycotoxin produced by the fungus Fusarium graminearum and other fungi that occurs mainly in grains such as wheat, barley, oats and maize, also known as vomitoxin.
DIMBOA (2,4-dihydroxy-7-methoxy-1,4-benzoxazin-3-one): Naturally occurring compound (hydroxamic acid) present in maize and other related grasses that serves as a defense against insects, fungi and bacteria.
Entomologist: Person who studies insects.
European corn borer: Stem-boring insect native to Europe that was accidently introduced into North America in the first decade of the 20th century. Larval stage is a pest of grain, particularly maize.
Fumonisin: Toxins produced by species of Fusarium molds that frequently occur in maize and other crops. Certain types are harmful to humans.
Gene flow: Transfer of genes from one population to another within the same species.
Genetic engineering: Insertion of a modified gene or gene from another organism using various recombinant DNA methods.
High-dose/refuge strategy: Tactic used to delay insect resistance to a genetically modified (GM) crop that involves planting non-GM plants (refuge) to produce susceptible insects. In order for the strategy to be effective the toxin dose in the plant must be sufficiently high to kill offspring derived from susceptible and resistant insects (i.e., heterozygous individuals).
Host plant resistance: Natural defenses plants evolved to reduce the impact of herbivores. Plant breeders often select these traits to reduce losses due to pests.
Insect resistance: Many insects have evolved resistance to insecticides, which can result in crop failures. Likewise insects can evolve resistance to GM plants.
Integrated pest management: A pest management strategy that uses a variety of methods usually biological control, host plant resistance, cultural control, and minimal use of pesticides to control crop pests.
Lepidoptera: An order of insects that includes butterflies and moths.
Leukoencephalomalacia: Disease of the central nervous system that affects horses, mules and donkeys, commonly called "Moldy Corn Poisoning".
Mycotoxins: A toxin produced by a fungus.
Natural insecticides: An insecticide produced from plant extracts, e.g., pyrethrum is produced from the flower chrysanthemum.
Open-pollinated: Pollination occurs by natural mechanisms such as insects, birds or wind. Maize is wind pollinated.
Plant pyramid: Plants with two or more Bt toxins targeted against the same pest.
Pulmonary edema: Abnormal build up of fluid in air sacs of lungs.
Western corn rootworm: A beetle from the family Chrysomelidae that is a major pest of maize in the United States. The main economic damage occurs when larvae feed on maize roots that can lead to plant lodging, harvesting difficulties and yield loss. In 1992 this beetle was discovered in Europe.
References and Recommended Reading
Bates, S. L. et al. Insect resistance management in GM crops: Past, present and future. Nature Biotechnology 23, 57-62 (2005). doi:10.1038/nbt1056
Brookes, G. & Barfoot, P. Global impact of biotech crops: Environmental effects, 1996-2008. AgBioForum 13, 76-94 (2010).
Candolfi, M. P. et al. A faunistic approach to assess potential side-effects of genetically modified Bt-corn on non-target arthropods under field conditions. Biocontrol Science and Technology 14, 129-170 (2004).
Dively, G. P. et al. Effects on monarch butterfly larvae (Lepidoptera: Danaidae) after continuous exposure to Cry1Ab-expressing corn during anthesis. Environmental Entomology 33, 1116-1125 (2004). doi:10.1603/0046-225x-33.4.1116
Dowd, P. F. Indirect reduction of ear molds and associated mycotoxins in Bacillus thuringiensis corn under controlled and open field conditions: Utility and limitations. Journal of Economic Entomology 93, 1669-1679 (2000).
Federici, B. A. "Case study: Bt crops - a novel mode of insect control," in Genetically Modified Crops: Assessing Safety, ed. K. T. Atherton (Taylor & Francis, 2002) 164-200.
Gómez-Barbero, M., Berbel, J., & Rodríguez-Cerezo, E. Bt corn in Spain - the performance of the EU's first GM crop. Nature Biotechnology 26, 384-386 (2008). doi:10.1038/nbt0408-384
Gould, F. Sustainability of transgenic insecticidal cultivars: Integrating pest genetics and ecology. Annual Review of Entomology 43, 701-726 (1998).
Hellmich, R. L. et al. "The present and future role of insect-resistant genetically modified maize in IPM," in Integration of Insect-Resistant Genetically Modified Crops within IPM Programs, eds. J. Romeis, A. M. Shelton, & G. G. Kennedy (Springer, 2008) 119-158.
Hellmich, R. L. et al. Monarch larvae sensitivity to Bacillus thuringiensis-purified proteins and pollen. Proceedings of the National Academy of Sciences of the United States of America 98, 11925-11930 (2001).
Hutchison, W. D. et al. Areawide suppression of European corn borer with Bt maize reaps savings to non-Bt maize growers. Science 330, 222-225 (2010). doi:10.1126/science.1190242
James, C. Global review of commercialized transgenic crops: 2002 Feature: Bt maize. ISAAA Briefs No. 29. (ISAAA, 2003) xiii + 182 pp. (article)
Jesse, L. C. H., & Obrycki, J. J. Field deposition of Bt transgenic corn pollen: Lethal effects on the monarch butterfly. Oecologia 125, 241-248 (2000).
Klun, J. A., Tipton, C. L., & Brindley, T. A. 2,4-dihydroxy-7-methoxy-1, 4-benzoxazin-3-one (DIMBOA), an active agent in the resistance of maize to the European corn borer. Journal of Economic Entomology 60, 1529-1533 (1967).
Losey, J. E., Rayor, L. S., & Carter, M. E. Transgenic pollen harms monarch larvae. Nature 399, 214 (1999).
Messeguer, J. Gene flow assessment in transgenic plants. Plant Cell Tissue and Organ Culture 73, 201-212 (2003).
Munkvold, G. P., Hellmich, R. L., & Rice, L. G. Comparison of fumonisin concentrations in kernels of transgenic Bt maize hybrids and nontransgenic hybrids. Plant Disease 83, 130-138 (1999).
Naranjo, S. E. Impacts of Bt crops on non-target invertebrates and insecticide use pattern. CAB Reviews: Perspectives in Agriculture, Veterinary Science, Nutrition and Natural Resources 11, 1-23 (2009). doi: 10.1079/PAVSNNR20094011 (link)
O'Callaghan, M. et al. Effects of plants genetically modified for insect resistance on nontarget organisms. Annual Review of Entomology 50, 271-292 (2005). doi:10.1146/annurev.ento.50.071803.130352
Pilcher, C. D., Rice, M. E., & Obrycki, J. J. Impact of transgenic Bacillus thuringiensis corn and crop phenology on five nontarget arthropods. Environmental Entomology 34, 1302-1316 (2005).
Pilcher, C. D. et al. Biotechnology and the European corn borer: Measuring historical farmer perceptions and adoption of transgenic Bt corn as a pest management strategy. Journal of Economic Entomology 95, 878-892 (2002).
Rice, M. E. Transgenic rootworm corn: Assessing potential agronomic, economic, and environmental benefits. Plant Health Progress (2004). doi:10.1094/PHP-2004-0301-01-RV
Romeis, J. et al. Assessment of risk of insect-resistant transgenic crops to nontarget arthropods. Nature Biotechnology 26, 203-208 (2008). doi:10.1038/nbt1381
Schnepf, E. et al. Bacillus thuringiensis and its pesticidal crystal proteins. Microbiology and Molecular Biology Reviews 62, 775-806 (1998).
Sears, M. K. et al. Impact of Bt corn pollen on monarch butterfly populations: A risk assessment. Proceedings of the National Academy of Sciences of the United States of America 98, 11937-11942 (2001).
Stanley-Horn, D. E. et al. Assessing the impact of Cry1Ab-expressing corn pollen on monarch butterfly larvae in field studies. Proceedings of the National Academy of Sciences of the United States of America 98, 11931-11936 (2001).
Storer, N. P., Dively, G. P., & Herman, R. A. "Landscape effects of insect-resistant genetically modified crops," in Integration of Insect-Resistant Genetically Modified Crops within IPM Programs, eds. J. Romeis, A. M. Shelton, & G. G. Kennedy (Springer, 2008) 273-302.
Tabashnik, B. E. Evolution of resistance to Bacillus thuringiensis. Annual Review of Entomology 39, 47-79 (1994).
Tabashnik, B. E., & Croft, B. A. Managing pesticide resistance in crop-arthropod complexes - Interactions between biological and operational factors. Environmental Entomology 11, 1137-1144 (1982).
Wu, F. Mycotoxin reduction in Bt corn: Potential economic, health, and regulatory impacts. Transgenic Research 15, 277-289 (2006). doi:10.1007/s11248-005-5237-1
Wu, F., Miller, J. D., & Casman, E. A. The economic impact of Bt corn resulting from mycotoxin reduction. Journal of Toxicology —Toxin Reviews 23, 397-424 (2004). doi:10.1081/txr-200027872