Abstract
Therapies to revascularize ischemic tissue have long been a goal for the treatment of vascular disease and other disorders. Therapies using stem cell factor (SCF), also known as a c-Kit ligand, had great promise for treating ischemia for myocardial infarct and stroke, however clinical development for SCF was stopped due to toxic side effects including mast cell activation in patients. We recently developed a novel therapy using a transmembrane form of SCF (tmSCF) delivered in lipid nanodiscs. In previous studies, we demonstrated tmSCF nanodiscs were able to induce revascularization of ischemia limbs in mice and did not activate mast cells. To advance this therapeutic towards clinical application, we tested this therapy in an advanced model of hindlimb ischemia in rabbits with hyperlipidemia and diabetes. This model has therapeutic resistance to angiogenic therapies and maintains long term deficits in recovery from ischemic injury. We treated rabbits with local treatment with tmSCF nanodiscs or control solution delivered locally from an alginate gel delivered into the ischemic limb of the rabbits. After eight weeks, we found significantly higher vascularity in the tmSCF nanodisc-treated group in comparison to alginate treated control as quantified through angiography. Histological analysis also showed a significantly higher number of small and large blood vessels in the ischemic muscles of the tmSCF nanodisc treated group. Importantly, we did not observe inflammation or mast cell activation in the rabbits. Overall, this study supports the therapeutic potential of tmSCF nanodiscs for treating peripheral ischemia.
Similar content being viewed by others
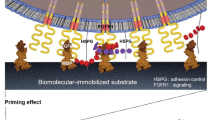
Introduction
Diabetes mellitus affects approximately 350 million people, leading to the death of an estimated 4.6 million people in the world per year1,2. Diabetes leads to a disturbance of the blood vessel by promoting vascular inflammation and endothelial cell dysfunction3,4. These abnormalities increase the severity of vascular disease in diabetic patients5. As a complication of diabetes, 30 to 40 percent of patients age 50 and older develop peripheral artery disease (PAD)6. Severe PAD increases the risk of non-healing ulcers, pain from intermittent claudication, and worst case for limb amputation. Current standard cares for PAD include physical therapy, medication, and surgical revascularization. Surgical bypass is an important treatment option for sever PAD, however many patients especially elderly patients are not eligible because of their diffusive arterial occlusions, no suitable veins for grafting and comorbidity7. Therapeutic angiogenesis by growth factors or growth factor genes is an appealing strategy to treat ischemia in this context but has been difficult to translate in the clinical setting. Many growth factors/gene therapies have shown promise in preclinical studies for ischemia only to show disappointing results in clinical trials in human patients8,9,10.
Stem cell factor (SCF) is a hematopoietic cytokine that communicates via the c-Kit receptor (CD117) and is also known as Kit ligand, Steel factor, or mast cell growth factor11. Stem cell factor is produced in cells as a transmembrane protein, which is then enzymatically processed into soluble SCF or a shorter version without the cleavable domain, that remains membrane-bound as transmembrane SCF (tmSCF)12. The activation of c-Kit through SCF signaling is crucial for maintaining hematopoietic stem cells (HSCs) and other progenitor cells in the bone marrow13,14. There are many potential therapeutic applications for SCF, including the enhancement of survival and expansion of HSCs after radiation exposure15,16, providing neuroprotection following a stroke17,18,19, and aiding the heart’s recovery after myocardial infarction20. In addition, SCF plays a crucial role in controlling mast cell development and activation21,22. Unfortunately, clinical and animal studies of SCF treatment have found induction of mast cell activation and anaphylaxis, significantly restricting its potential for therapeutic use22,23,24,25,26. We recently found that tmSCF delivered in proteoliposomes or lipid nanodiscs was effective in inducing revascularization of ischemic limbs in diabetic and wild type mice but did not lead to mast cell activation27. Thus, tmSCF delivered in nanocarriers may provide therapeutic benefits of soluble SCF without the toxic side effects that limited its usefulness as a therapeutic.
While our previous studies have supported the potential of tmSCF nanodiscs as therapies for ischemia, large animal studies are needed to demonstrate efficacy to support further studies in human trials. For peripheral ischemia, rabbits are typically used as the precursor to human studies for treating PAD due the well-developed musculature of the lower limb. Our group recently developed an optimized rabbit model of hindlimb ischemia that includes hyperlipidemia and diabetes28,29. A major advantage of this model is that it exhibits resistance to angiogenic therapies and longer-term ischemia, similar to human patients, in contrast to health rabbit models that revascularize more quickly and have been found to correlate poorly to outcomes in clinical trials. In this study, we used this advanced rabbit model of limb ischemia to evaluate the efficacy of tmSCF nanodiscs in treating peripheral ischemia. Overall, our results demonstrate that tmSCF nanodiscs improve revascularization in ischemia compared to alginate gel control in a large animal without significant inflammatory reaction.
Methods
Preparation of tmSCF nanodiscs
A solution of 50 mM 1-palmitoyl-2-oleoyl-sn-glycero-3-phosphocholine (POPC; Avanti Polar Lipids, Inc.) was created in chloroform and then evaporated to make a thin film. The POPC was resuspended in a solution of 100 mM sodium deoxycholate using multiple cycles of sonication. The MSP protein (5 mg/mL MSP1D1; Sigma, Inc.) was then added to phospholipid solution, and the detergent concentration was adjusted to 40 mM. This construct was then incubated for 15 min at 4 °C. To solubilize the membrane protein, tmSCF was incubated in the n-octyl-β-D-glucopyranoside (1% w/v; Sigma) for 15 min at 4 °C. In the separate tube, a solution was created of 1 µg/ml, 1% n-octyl-β-D-glucopyranoside (1% w/v) and 40 mM sodium deoxycholate. The POPC and tmSCF solution were combined for 1 h at 4 °C. The combined solution was then dialysed overnight use a 300 K MWCO dialysis membrane (Thermo Fisher). Biobeads (SM-2; Bio-Rad Laboratories, Inc) were then used to remove any residual detergent (Supplemental Fig. 1).
Preparation of alginate gels and crosslinking
Sterile alginate powder (Sigma) was added to sterile saline to create a 2% w/v solution. The final concentration of tmSCF nanodiscs was adjusted to 50 µg/ml in this 2% alginate solution. To prepare the crosslinker, calcium sulfate was added to sterile saline to create 0.2% w/v solution. Both the alginate solution and the crosslinker were taken up into a 1 ml syringe just before injection (100 μl of each solution for 200 μl total per injection).
Induction of diabetes in rabbits
Studies involving animals were performed with the approval of the University of Texas at Austin and the University of Texas Health Science Center at Houston Institutional Animal Care and Use Committees (IACUCs), the Animal Care and Use Review Office (ACURO) of the United States Army Medical Research and Materiel Command Office of Research Protections, and in accordance with NIH guidelines for animal care. The studies performed were in accordance with ARRIVE guidelines. The model was performed as described in our previous studies in which we optimized the model for reduced recovery from ischemia28,29. New Zealand rabbits (male; 4–6 months of age; Charles River, Inc.) were transitioned from standard alfalfa chow to a 0.1% cholesterol diet over the course of five days. After two weeks on the 0.1% cholesterol diet, rabbits were induced with diabetes using an intravenous alloxan injection. After sedating the rabbits, a bassline blood glucose measurement was attained. Alloxan (100 mg/kg; Sigma) was injected through an IV into the rabbit at flow rate of 1 ml/min for eight minutes using a syringe pump. Blood glucose levels were monitored closely for 12 h after the injection. A successful induction of diabetes was determined if the rabbit’s blood glucose level remained over 150 mg/dl prior to insulin administration.
Hind limb ischemia surgery and treatments
Rabbits were initially anesthetized with a subcutaneous injection of 20–40 mg/kg ketamine and 2 mg/kg midazolam. During the initial sedation, the rabbits were anesthetized with 1.5–3% isoflurane gas using a mask. To sustain the anesthesia, an intramuscular injection of alfaxalone at a dosage of 3 mg/kg was used. Once the rabbit had been anesthetized, the mask was removed and a cuffed endotracheal tube, connected to a ventilator, was inserted into the airway. The administration of isoflurane continued at 1.5–3%. Blood was collected from the central artery of either ear for a baseline chemistry panel. A 22G ear vein catheter was placed in the lateral ear vein for a Lactated Ringer’s Solution drip throughout the surgical procedure. Using the lateral vein in the opposite ear, a catheter was placed in the vein and alfaxalone was delivered at 6 mg/kg/hr. The alfaxalone was gradually increased to 8 mg/kg/hr while decreasing isoflurane to 0.6% during the prep period. To limit pain and risk of infection, buprenorphine (0.01 mg/kg) and Baytril (5 mg/kg) were administered using a subcutaneous injection with a 25G needle. Further details of the procedure can be found in our previous publications28,29. To induce ischemia in the hind limb of New Zealand rabbits, a longitudinal incision was made in the skin over the femoral artery. The femoral artery was exposed using blunt dissection. One percent lidocaine was applied to the area to reduce nerve irritation and promote vasodilation. Continued blunt dissection was used to expose the entire length of the femoral artery and branches including the inferior epigastric, deep femoral, lateral circumflex, and superficial epigastric arteries. The tissue was kept moistened with saline to avoid damage. The femoral artery was then carefully separated from vein and nerve. The femoral artery was then ligated with 4.0 silk sutures (Ethicon), cut, and excised. 2 weeks after hind limb ischemia surgery, ten syringes containing 100 μl of alginate with treatment and 100 μl of calcium sulfate crosslinker were prepared for intramuscular injection (200 μl total volume of injection). The injections were placed evenly along both sides of the femoral artery on the thigh. To achieve uniform injections, a silicone sheet with holes to guide the injection was used28,29. Treatments included tmSCF nanodiscs in alginate and an alginate-only control. At the study endpoint, angiograph was performed, the animals were euthanized and pressure perfused as described in our previous studies28,29.
Angiography quantification
Angiograms were quantified using grid analysis techniques with ImageJ Software. Briefly, brightness and contrast were adjusted to better visualize vessels. A grid overlay of 100 pixels per square was used for foot quantification and 750 pixels per square was used for the thigh vasculature quantification. The multi-point tool was used to count intersections between the grid and vessels. To access the carotid artery, an incision was made just lateral to the trachea. Blunt dissection was used to expose the carotid artery and separate it from the jugular vein and vagus nerve. Ligatures were placed at the proximal and distal ends of the carotid artery. The distal end was tied off and a ligaloop (Covidien) was placed at the proximal end. A wire insertion tool was then inserted into the artery. Using the tool, a guidewire was fed into the artery to the aortic bifurcation in the descending aorta. The insertion tool was then removed and a 3F pigtail angiographic catheter (Boston Scientific) was placed over the wire and advanced 2 cm proximal to the aortic bifurcation. Nitroglycerine and lidocaine were given to increase vasodilation. Contrast media was injected through the catheter using an automated angiographic injector. Angiography was performed before femoral ligation, after femoral ligation, and before sacrifice at week 10.
Histological analysis of muscle vascularity
The samples underwent a standard procedure of formalin fixation and paraffin embedding before being sectioned. Using a microtome, the samples were sliced into sections, each measuring 6 µm in thickness. Deparaffinization was carried out on the sections, followed by a three-hour treatment with an antigen retrieval solution (Dako) at 80 °C. Once cooled to room temperature, the sections were blocked with 20% fetal bovine serum for 45 min and then immunostained using the EnVision + Kit (Dako). The staining process included an overnight incubation at 4 °C with a 1:100 dilution of the primary antibody to PECAM-1 (Thermo Scientific; PA5-16,301). After staining, the samples were rinsed and processed for secondary staining as per the manufacturer's guidelines. The samples were then visualized under a bright field microscope (Meiji). For quantification, sections were analyzed from the three biopsies of the tissue from each rabbit. Three low magnification images were taken from each biopsy and the vessel density was quantified by using manual vessel counting. Analyses were performed in a blinded manner.
Statistical analysis
All results are shown as mean ± standard error of the mean. Comparisons between only two groups were performed using a 2-tailed Student’s t-test. Differences were considered significant at p < 0.05. Multiple comparisons between groups were analyzed by 2-way ANOVA followed by a Tukey post-hoc test. A 2-tailed probability value < 0.05 was considered statistically significant.
Ethics approval and consent to participate
Studies involving animals were performed with the approval of the University of Texas at Austin and the UTHealth Science Center at Houston Institutional Animal Care and Use Committee (IACUC), the Animal Care and Use Review Office (ACURO) of The United States Army Medical Research and Materiel Command Office of Research Protections, and in accordance with NIH guidelines for animal care.
Results
Transmembrane SCF nanodiscs enhance revascularization of the ischemic thigh muscles of diabetic, hyperlipidemic rabbits
Rabbits were given a high fat diet for 4 weeks and were induced to develop diabetes two weeks prior to surgery for inducing unilateral limb ischemia as described previously28,29. Angiograms were taken before and immediately after the surgery to assess the induction of ischemia in the limbs (Supplemental Fig. 2). The rabbits were then allowed to recover for two weeks to avoid treatment during the acute healing phase of recovery29. The rabbits were given ten injections of alginate with or without the tmSCF nanodiscs and recovery was assess using angiography after 10 weeks (Fig. 1A; Supplemental Fig. 2). Vessel grid intersection counts showed significant vasculature increase for the tmSCF nanodisc group compared to the alginate control (Fig. 1B). When ratioed to the contralateral control limb at week 10, the tmSCF nanodisc group significantly improved recovery compared to the alginate control (Fig. 1C). A ratio was also taken of the grid intersections counted in the ischemic thigh at week 10 to the intersections counted in the ischemic thigh before hind limb surgery. The results show a significant increase in vascularity for the tmSCF nanodisc group in comparison to the control group (Fig. 1D). A new vessel formation is indicated by tortuosity (corkscrew like morphology), which was also counted to assess angiogenic capacity of tmSCF nanodiscs. The results also showed a significant increase in tmSCF nanodisc group in comparison to control. (Fig. 1E).
Transmembrane SCF nanodiscs enhance revascularization in the thigh of diabetic, hyperlipidemic rabbits with hindlimb ischemia. (A) Angiograms of the thighs of the rabbits at week 10. The control limb is shown on the right and the limb with the ligated femoral artery is on the left. (B) Quantification of vessel grid intersections counted in the ischemic thigh at the model endpoint. The vessels are counted as number of intersections with an overlayed grid. (C) Relative vascularity of the ischemic thigh ratioed to the contralateral control thigh at the model endpoint. (D) Relative vascularity of the ischemic thigh ratioed to the thigh at day 1 prior to ligation. (E) Quantification of new vessels counted in the ischemic thigh at the model endpoint. *p < 0.05 versus alginate; **p < 0.01 versus alginate. (n = 3).
Transmembrane SCF nanodiscs enhance revascularization of the ischemic calf and foot of diabetic, hyperlipidemic rabbits
Postoperative angiogram showed the most severe ischemia is the area of the foot and calf muscles (Supplemental Fig. 2). 10 weeks after the operation, robust vascular network formation were observed at the foot of the tmSCF nanodiscs treated group while almost few or no blood vessels was observed in the control group by angiography (Fig. 2A). Quantitative analysis on vessel count showed a significantly higher blood vessel numbers in tmSCF nanodisc group in comparison to the control (Fig. 2B). Similarly, significantly higher ratio of vascular count at the ischemic calf and foot when ratioed to the contralateral control at week 10 (Fig. 2C). A ratio of vessel counts at the ischemic thigh at week 10 to the vessel counts before surgery also demonstrated the effectiveness of the tmSCF nanodisc treatment (Fig. 2D).
tmSCFND enhances revascularization in the calf and foot of diabetic, hyperlipidemic rabbits with hindlimb ischemia. (A) Angiograms of the lower limb of the rabbits at week 10. (B) Quantification of vessels counted in the ischemic calf and foot at the model endpoint. The vessels are counted as number of intersections with an overlayed grid. (C) Relative vascularity of the ischemic limb ratioed to the contralateral control limb at the model endpoint. (D) Relative vascularity of the ischemic calf and foot ratioed to the calf and foot at day 1 prior to ligation. *p < 0.05 versus alginate; **p < 0.05 versus alginate (n = 3).
Histological analysis confirmed increased vascularity in tmSCF nanodisc treated leg of rabbits with diabetes and hyperlipidemia
Injection of soluble SCF leads to activation of mast cells and mast cell infiltration22,23,30,31,32,33. This led to SCF inducing anaphylactic type response in some human patients and in animal models, which is a major limitation in translating soluble SCF in to a clnical therapy22,23,34,35,36. We next performed a histological analysis on biopsies from the ischemic muscle of the rabbits. Three biopsies were taken from the muscle of each rabbit close to the site of injection. PECAM immunostaining was used to count small and large vessels formation (Fig. 3A). Significantly more small vessels and large vessels were confirmed with tmSCF nanodisc treatment, indicating that tmSCF nanodiscs induced both angiogenesis and arteriogenesis in the rabbit ischemia model (Fig. 3B). If there were mast cell activation or an inflammatory response, mast cell activation and infiltration would be expected to lead to a rash, edema and infiltration of immune cells22,23,34,35,36. However, no signs of inflammation or edema were found on the H&E staining of thigh and calf muscle tissues. Mast cell activation would also have several rapid effects including potentially a drop in blood pressure and a reduction in body temperature. Blood pressure and body temperature were monitored during the injection and we did not observe any difference between the groups during the injection.
Histological analysis of vascularization of calf treated by tmSCF nanodiscs. (A) Upper: H&E staining analysis of biopsies from the ischemic hind limbs of rabbits. Bottom: PECAM staining analysis of biopsies from the ischemic hind limbs of rabbits. Scale bar = 100 µm. (B) Left: Quantification of small vessels counted in the ischemic calf at week 10. Right: Quantification of arterioles vessels counted in the ischemic calf at week 10. *p < 0.05 versus alginate group (n = 3).
Discussion
Stem cell factor is a therapeutic protein with many potential uses for treating disease. Unfortunately, its use has been severely limited by toxic effects that were observed in animal studies and human clinical trials22,23,24,25,26. Managing ischemia in diabetic individuals has presented continuing challenges in the clinical setting, particularly for those undergoing bypass operations or percutaneous procedures37,38 where diabetic patients have worse outcomes and reduced benefits from therapy and interventions. Our previous work demonstrated that tmSCF nanodiscs enhance revascularization in healthy and diabetic mice27. In this work, we evaluated the efficacy of tmSCF embedded in nanodiscs for therapeutic angiogenesis using an optimized rabbit model of hindlimb ischemia with diabetes and hyperlipidemia28,29. Angiography of the thigh and calf muscle treated with tmSCF nanodiscs showed significantly higher numbers of functional blood vessels and new vessels. Histological analysis on PECAM staining and H&E staining also demonstrated significantly higher numbers of small and large vessel formations compared control, indicating that tmSCF nanodiscs support both angiogenesis and arteriogenesis. The findings are particularly significant given the rabbit model’s resistance to angiogenesis and longer-term ischemia28,29. Finally, we did not observe any signs of mast cell activation on histological analysis, consistent with our prior studies in mice27.
A major goal of this study was to provide evidence in support of the translation of tmSCF nanodiscs toward clinical studies. This study successfully provides evidence of efficacy in a second non-rodent animal model when combined with our prior study in mice27. A rabbit model was selected as this is often the most appropriate preclinical large animal model for limb ischemia due to its significant lower limb musculature in rabbits compared to other large animals commonly used in preclinical research. The specific rabbit model used was previously optimized by our group to which demonstrating that diabetic hyperlipidemic rabbits experience reduced recovery and extended ischemia, contrasting starkly with healthier counterparts28,29. In accordance with the findings of our study, the use of an optimized rabbit model of hindlimb ischemia with diabetes and hyperlipidemia provides a more clinically relevant and representative model for studying therapeutic interventions for ischemic conditions. Our study supports that tmSCF nanodiscs significantly enhanced revascularization in the diabetic, hyperlipidemic rabbit model. This is a notable finding considering the model's resistance to angiogenesis and extended ischemia periods28,29. However, the challenges of comparing with other studies due to varying techniques and use of healthy rabbits in most prior studies must be recognized. Despite these challenges, our findings using a diseased rabbit model are comparable, if not superior, to previous studies utilizing healthy rabbit models, suggesting potential applicability of tmSCF nanodiscs as a therapeutic for ischemia in the human clinical setting, even in the context of therapeutic resistance as seen in patients with hyperlipidemia and diabetes.
Our study also found no evidence of activation of mast cells in the muscle tissues of the rabbits by the tmSCF nanodiscs. Studies have shown that rabbits, being phylogenetically closer to humans than rodents, exhibit similarities in mast cell activation by stem cell factor (SCF) to that of humans, indicating a potential "nerve-mast cell-myofibroblast axis" in some inflammatory processes leading to fibrogenic outcomes39. Stem cell factor is highly conserved among mammals and there is an 86% similarity between the human and rabbit SCF amino acid sequences. In addition, a mast cell inhibitory drug that works on human mast cells also works to inhibit mast cells in a joint fibrosis model in rabbits40,41. For their similar reasons, rabbits have also been used as a preclinical model of asthma and respiratory mast cell activation42. Similarly, isolated mast cells from rabbits can be stimulated to have dose dependent release of histamine in response several drugs in a way similar to that of human mast cells43. Histamine regulation of glaucoma in rabbits in the eye also behaves similarly to human and rabbit mast cell express similar receptors to human mast cells44. Thus, this work provides additional evidence that the tmSCF nanodisc treatments do not activate mast cells to a significant extent, consistent with our previous findings in mice27.
Prior studies have explored the use of many treatments in rabbits and other large animals for treating peripheral ischemia and ischemia wounds45,46. A lack of uniformity in the techniques employed for performing and analyzing preclinical hindlimb ischemia models in rabbits makes it difficult to directly compare with other studies. In addition, the majority of prior studies have also used healthy rabbits, which have more rapid recovery from ischemia and responsiveness to angiogenic treatments. Overall, the improvement in revascularization observed in our research using a diseased rabbit model is comparable or superior to earlier studies that utilized healthy rabbit models of hindlimb ischemia to assess protein therapeutics47,48,49,50,51,52,53,54,55,56,57,58,59,60,61,62,63,64,65,66,67,68,69, cell therapies70,71,72,73,74, or gene therapies67,75,76,77,78,79,80,81,82,83,84,85,86,87. Consequently, this work supports that tmSCF nanodiscs would have potential as a therapeutic for ischemia, even in the context of therapeutic resistance as would be found in many human patients that have hyperlipidemia and diabetes.
Data availability
The datasets used and/or analyzed during the current study are available from the corresponding author on reasonable request.
References
Liang, J. et al. The C-kit receptor-mediated signal transduction and tumor-related diseases. Int. J. Biol. Sci. 9, 435–443. https://doi.org/10.7150/ijbs.6087 (2013).
Futrega, K., King, M., Lott, W. B. & Doran, M. R. Treating the whole not the hole: necessary coupling of technologies for diabetic foot ulcer treatment. Trends Mol. Med. 20, 137–142. https://doi.org/10.1016/j.molmed.2013.12.004 (2014).
Rajendran, P. et al. The vascular endothelium and human diseases. Int. J. Biol. Sci. 9, 1057–1069. https://doi.org/10.7150/ijbs.7502 (2013).
Avogaro, A., Albiero, M., Menegazzo, L., de Kreutzenberg, S. & Fadini, G. P. Endothelial dysfunction in diabetes. Role Reparat. Mech. 34, S285–S290. https://doi.org/10.2337/dc11-s239%JDiabetesCare (2011).
Thiruvoipati, T., Kielhorn, C. E. & Armstrong, E. J. Peripheral artery disease in patients with diabetes: Epidemiology, mechanisms, and outcomes. World J. Diabetes 6, 961–969. https://doi.org/10.4239/wjd.v6.i7.961 (2015).
Tursi, F. J. & Walker, C. Essential keys to diagnosing and treating PAD in patients with diabetes. Podiatry Today 30, 12–17 (2017).
Na, C. R., Wang, S., Kirsner, R. & Federman, G. The elderly and peripheral arterial disease. Clin. Geriatrics. 19, 41–46 (2011).
Simons, M. & Ware, J. A. Therapeutic angiogenesis in cardiovascular disease. Nat. Rev. Drug Discov. 2, 863–871. https://doi.org/10.1038/nrd1226 (2003).
Cooke, J. P. & Meng, S. Vascular regeneration in peripheral artery disease. Arterioscler. Thromb. Vasc. Biol. 40, 1627–1634. https://doi.org/10.1161/ATVBAHA.120.312862 (2020).
Annex, B. H. Therapeutic angiogenesis for critical limb ischaemia. Nat. Rev. Cardiol. 10, 387–396. https://doi.org/10.1038/nrcardio.2013.70 (2013).
Broudy, V. C. Stem cell factor and hematopoiesis. Blood 90, 1345–1364. https://doi.org/10.1182/blood.V90.4.1345 (1997).
Lennartsson, J. & Ronnstrand, L. Stem cell factor receptor/c-Kit: from basic science to clinical implications. Physiol. Rev. 92, 1619–1649. https://doi.org/10.1152/physrev.00046.2011 (2012).
Ding, L., Saunders, T. L., Enikolopov, G. & Morrison, S. J. Endothelial and perivascular cells maintain haematopoietic stem cells. Nature 481, 457–462. https://doi.org/10.1038/nature10783 (2012).
Li, C. L. & Johnson, G. R. Stem cell factor enhances the survival but not the self-renewal of murine hematopoietic long-term repopulating cells. Blood 84, 408–414 (1994).
Gardner, R. V., Oliver, P. & Astle, C. M. Stem cell factor improves the repopulating ability of primitive hematopoietic stem cells after sublethal irradiation (and, to a lesser extent) after bone marrow transplantation in mice. Stem Cells 16, 112–119. https://doi.org/10.1002/stem.160112 (1998).
Zsebo, K. M. et al. Radioprotection of mice by recombinant rat stem cell factor. Proc. Natl. Acad. Sci. U. S. A. 89, 9464–9468. https://doi.org/10.1073/pnas.89.20.9464 (1992).
Zhao, L. R., Piao, C. S., Murikinati, S. R. & Gonzalez-Toledo, M. E. The role of stem cell factor and granulocyte-colony stimulating factor in treatment of stroke. Recent Pat. CNS Drug Discov. 8, 2–12. https://doi.org/10.2174/1574889811308010002 (2013).
Ping, S. et al. Stem cell factor and granulocyte colony-stimulating factor promote brain repair and improve cognitive function through VEGF-A in a mouse model of CADASIL. Neurobiol. of Dis. 132, 104561. https://doi.org/10.1016/j.nbd.2019.104561 (2019).
Bath, P. M. W., Sprigg, N. & England, T. Colony stimulating factors (including erythropoietin, granulocyte colony stimulating factor and analogues) for stroke. Cochrane Database Syst. Rev. https://doi.org/10.1002/14651858.CD005207.pub4 (2013).
Yaniz-Galende, E. et al. Stem cell factor gene transfer promotes cardiac repair after myocardial infarction via in situ recruitment and expansion of c-kit+ cells. Circ. Res. 111, 1434–1445. https://doi.org/10.1161/CIRCRESAHA.111.263830 (2012).
Galli, S. J. et al. Reversible expansion of primate mast cell populations in vivo by stem cell factor. J. Clin. Invest. 91, 148–152. https://doi.org/10.1172/JCI116164 (1993).
Taylor, A. M., Galli, S. J. & Coleman, J. W. Stem-cell factor, the kit ligand, induces direct degranulation of rat peritoneal mast cells in vitro and in vivo: Dependence of the in vitro effect on period of culture and comparisons of stem-cell factor with other mast cell-activating agents. Immunology 86, 427–433 (1995).
Costa, J. J. et al. Recombinant human stem cell factor (kit ligand) promotes human mast cell and melanocyte hyperplasia and functional activation in vivo. J. Exp. Med. 183, 2681–2686. https://doi.org/10.1084/jem.183.6.2681 (1996).
Da Silva, C. A., Reber, L. & Frossard, N. Stem cell factor expression, mast cells and inflammation in asthma. Fundam. Clin. Pharmacol. 20, 21–39. https://doi.org/10.1111/j.1472-8206.2005.00390.x (2006).
Wershil, B. K., Tsai, M., Geissler, E. N., Zsebo, K. M. & Galli, S. J. The rat c-kit ligand, stem cell factor, induces c-kit receptor-dependent mouse mast cell activation in vivo. Evidence that signaling through the c-kit receptor can induce expression of cellular function. J. Exp. Med. 175, 245–255. https://doi.org/10.1084/jem.175.1.245 (1992).
Frangogiannis, N. G. et al. Stem cell factor induction is associated with mast cell accumulation after canine myocardial ischemia and reperfusion. Circulation 98, 687–698. https://doi.org/10.1161/01.cir.98.7.687 (1998).
Takematsu, E. et al. Transmembrane stem cell factor protein therapeutics enhance revascularization in ischemia without mast cell activation. Nat. Commun. 13, 2497. https://doi.org/10.1038/s41467-022-30103-2 (2022).
Sligar, A. D. et al. Syndecan-4 proteoliposomes enhance revascularization in a rabbit hind limb ischemia model of peripheral ischemia. Acta Biomater. 167, 425–435. https://doi.org/10.1016/j.actbio.2023.06.006 (2023).
Sligar, A. D. et al. Preclinical model of hind limb ischemia in diabetic rabbits. J .Vis. Exp. https://doi.org/10.3791/58964 (2019).
Furuta, G. T., Ackerman, S. J., Lu, L., Williams, R. E. & Wershil, B. K. Stem cell factor influences mast cell mediator release in response to eosinophil-derived granule major basic protein. Blood 92, 1055–1061 (1998).
Feldweg, A. M. et al. gp49B1 suppresses stem cell factor-induced mast cell activation-secretion and attendant inflammation in vivo. Eur. J. Immunol. 33, 2262–2268. https://doi.org/10.1002/eji.200323978 (2003).
MacNeil, A. J., Junkins, R. D., Wu, Z. & Lin, T. J. Stem cell factor induces AP-1-dependent mast cell IL-6 production via MAPK kinase 3 activity. J. Leukoc. Biol. 95, 903–915. https://doi.org/10.1189/jlb.0713401 (2014).
Nam, S. Y., Kim, H. Y., Kim, H. M. & Jeong, H. J. Betaeta-eudesmol reduces stem cell factor-induced mast cell migration. Int. Immunopharmacol. 48, 1–7. https://doi.org/10.1016/j.intimp.2017.04.017 (2017).
Shpall, E. J. et al. A randomized phase 3 study of peripheral blood progenitor cell mobilization with stem cell factor and filgrastim in high-risk breast cancer patients. Blood 93, 2491–2501 (1999).
Facon, T. et al. Stem cell factor in combination with filgrastim after chemotherapy improves peripheral blood progenitor cell yield and reduces apheresis requirements in multiple myeloma patients: a randomized, controlled trial. Blood 94, 1218–1225 (1999).
Maslak, P. & Nimer, S. D. The efficacy of IL-3, SCF, IL-6, and IL-11 in treating thrombocytopenia. Semin. Hematol. 35, 253–260 (1998).
Fitridge, R., Pena, G. & Mills, J. L. The patient presenting with chronic limb-threatening ischaemia. Does diabetes influence presentation, limb outcomes and survival?. Diabetes Metab. Res. Rev. https://doi.org/10.1002/dmrr.3242 (2019).
Takahara, M., Iida, O., Kohsaka, S., Soga, Y., Fujihara, M., Shinke, T., Amano, T., Ikari, Y., & JEVT, investigators JP. Diabetes mellitus and other cardiovascular risk factors in lower-extremity peripheral artery disease versus coronary artery disease: an analysis of 1,121,359 cases from the nationwide databases. Cardiovasc. Diabetol. 18, 155. https://doi.org/10.1186/s12933-019-0955-5 (2019).
Hart, D. A. Curbing inflammation in multiple sclerosis and endometriosis: Should mast cells be targeted?. Int J Inflam. 2015, 452095. https://doi.org/10.1155/2015/452095 (2015).
Monument, M. J. et al. The mast cell stabilizer ketotifen fumarate lessens contracture severity and myofibroblast hyperplasia: A study of a rabbit model of posttraumatic joint contractures. J. Bone Joint. Surg. Am. 92, 1468–1477. https://doi.org/10.2106/JBJS.I.00684 (2010).
Monument, M. J. et al. The mast cell stabilizer ketotifen reduces joint capsule fibrosis in a rabbit model of post-traumatic joint contractures. Inflamm. Res. 61, 285–292. https://doi.org/10.1007/s00011-011-0409-3 (2012).
Kamaruzaman, N. A., Kardia, E., Kamaldin, N., Latahir, A. Z. & Yahaya, B. H. The rabbit as a model for studying lung disease and stem cell therapy. Biomed. Res. Int. 2013, 691830. https://doi.org/10.1155/2013/691830 (2013).
Ennis, M., Lorenz, W., Gerland, W. & Heise, J. Isolation of mast cells from rabbit lung and liver: comparison of histamine release induced by the hypnotics Althesin and propanidid. Agents Actions. 20, 219–222. https://doi.org/10.1007/BF02074674 (1987).
Lanzi, C. et al. Role of histamine H(3) receptor antagonists on intraocular pressure reduction in rabbit models of transient ocular hypertension and glaucoma. Int. J. Mol. Sci. https://doi.org/10.3390/ijms20040981 (2019).
Annex, B. H. & Cooke, J. P. New directions in therapeutic angiogenesis and arteriogenesis in peripheral arterial disease. Circ. Res. 128, 1944–1957. https://doi.org/10.1161/CIRCRESAHA.121.318266 (2021).
Veith, A. P., Henderson, K., Spencer, A., Sligar, A. D. & Baker, A. B. Therapeutic strategies for enhancing angiogenesis in wound healing. Adv. Drug Deliv. Rev. 146, 97–125. https://doi.org/10.1016/j.addr.2018.09.010 (2019).
Witzenbichler, B. et al. Vascular endothelial growth factor-C (VEGF-C/VEGF-2) promotes angiogenesis in the setting of tissue ischemia. Am. J. Pathol. 153, 381–394. https://doi.org/10.1016/S0002-9440(10)65582-4 (1998).
Taniyama, Y. et al. Therapeutic angiogenesis induced by human hepatocyte growth factor gene in rat and rabbit hindlimb ischemia models: Preclinical study for treatment of peripheral arterial disease. Gene Ther. 8, 181–189. https://doi.org/10.1038/sj.gt.3301379 (2001).
Takeshita, S. et al. Therapeutic angiogenesis following arterial gene transfer of vascular endothelial growth factor in a rabbit model of hindlimb ischemia. Biochem. Biophys. Res. Commun. 227, 628–635. https://doi.org/10.1006/bbrc.1996.1556 (1996).
Pu, L. Q. et al. Enhanced revascularization of the ischemic limb by angiogenic therapy. Circulation 88, 208–215. https://doi.org/10.1161/01.cir.88.1.208 (1993).
Morishita, R. et al. Therapeutic angiogenesis induced by human recombinant hepatocyte growth factor in rabbit hind limb ischemia model as cytokine supplement therapy. Hypertension 33, 1379–1384. https://doi.org/10.1161/01.hyp.33.6.1379 (1999).
Li, M. et al. Mutant hypoxia inducible factor-1alpha improves angiogenesis and tissue perfusion in ischemic rabbit skeletal muscle. Microvasc. Res. 81, 26–33. https://doi.org/10.1016/j.mvr.2010.09.008 (2011).
Karatzas, A. et al. NGF promotes hemodynamic recovery in a rabbit hindlimb ischemic model through trkA- and VEGFR2-dependent pathways. J. Cardiovasc. Pharmacol. 62, 270–277. https://doi.org/10.1097/FJC.0b013e3182982de7 (2013).
Hopkins, S. P. et al. Controlled delivery of vascular endothelial growth factor promotes neovascularization and maintains limb function in a rabbit model of ischemia. J. Vasc. Surg. 27, 886–894. https://doi.org/10.1016/s0741-5214(98)70269-1 (1998).
Fabre, J. E., Rivard, A., Magner, M., Silver, M. & Isner, J. M. Tissue inhibition of angiotensin-converting enzyme activity stimulates angiogenesis in vivo. Circulation 99, 3043–3049. https://doi.org/10.1161/01.cir.99.23.3043 (1999).
Chung, Y. I. et al. Efficient revascularization by VEGF administration via heparin-functionalized nanoparticle-fibrin complex. J. Control Release. 143, 282–289. https://doi.org/10.1016/j.jconrel.2010.01.010 (2010).
Nitta, N. et al. Vascular regeneration by pinpoint delivery of growth factors using a microcatheter reservoir system in a rabbit hind-limb ischemia model. Exp. Ther. Med. 4, 201–204. https://doi.org/10.3892/etm.2012.574 (2012).
Doi, K. et al. Enhanced angiogenesis by gelatin hydrogels incorporating basic fibroblast growth factor in rabbit model of hind limb ischemia. Heart Vessels 22, 104–108. https://doi.org/10.1007/s00380-006-0934-0 (2007).
Wafai, R., Tudor, E. M., Angus, J. A. & Wright, C. E. Vascular effects of FGF-2 and VEGF-B in rabbits with bilateral hind limb ischemia. J. Vasc. Res. 46, 45–54. https://doi.org/10.1159/000139132 (2009).
Lebherz, C. et al. Therapeutic angiogenesis/arteriogenesis in the chronic ischemic rabbit hindlimb: effect of venous basic fibroblast growth factor retroinfusion. Endothelium 10, 257–265. https://doi.org/10.1080/10623320390246432 (2003).
Seko, A. et al. Vascular regeneration by repeated infusions of basic fibroblast growth factor in a rabbit model of hind-limb ischemia. AJR Am. J. Roentgenol. 192, W306-310. https://doi.org/10.2214/AJR.07.4013 (2009).
Kondoh, K. et al. Conduction performance of collateral vessels induced by vascular endothelial growth factor or basic fibroblast growth factor. Cardiovasc. Res. 61, 132–142. https://doi.org/10.1016/j.cardiores.2003.10.003 (2004).
Hosaka, A. et al. Gelatin hydrogel microspheres enable pinpoint delivery of basic fibroblast growth factor for the development of functional collateral vessels. Circulation 110, 3322–3328. https://doi.org/10.1161/01.CIR.0000147779.17602.18 (2004).
Li, J. et al. Synergistic effects of FGF-2 and PDGF-BB on angiogenesis and muscle regeneration in rabbit hindlimb ischemia model. Microvasc. Res. 80, 10–17. https://doi.org/10.1016/j.mvr.2009.12.002 (2010).
Lederman, R. J. et al. Therapeutic angiogenesis with recombinant fibroblast growth factor-2 for intermittent claudication (the TRAFFIC study): A randomised trial. Lancet 359, 2053–2058. https://doi.org/10.1016/s0140-6736(02)08937-7 (2002).
Simons, M. et al. Pharmacological treatment of coronary artery disease with recombinant fibroblast growth factor-2: Double-blind, randomized, controlled clinical trial. Circulation 105, 788–793. https://doi.org/10.1161/hc0802.104407 (2002).
Gowdak, L. H. et al. Adenovirus-mediated VEGF(121) gene transfer stimulates angiogenesis in normoperfused skeletal muscle and preserves tissue perfusion after induction of ischemia. Circulation 102, 565–571. https://doi.org/10.1161/01.cir.102.5.565 (2000).
Cao, R. et al. Angiogenic synergism, vascular stability and improvement of hind-limb ischemia by a combination of PDGF-BB and FGF-2. Nat Med. 9, 604–613. https://doi.org/10.1038/nm848 (2003).
Takeshita, S. et al. Therapeutic angiogenesis. A single intraarterial bolus of vascular endothelial growth factor augments revascularization in a rabbit ischemic hind limb model. J Clin Invest. 93, 662–670. https://doi.org/10.1172/JCI117018 (1994).
Chua, K. et al. Cord lining mesenchymal stem cells have a modest positive effect on angiogenesis in hindlimb ischemia. Front. Cell Dev. Biol. 8, 596170. https://doi.org/10.3389/fcell.2020.596170 (2020).
Mikami, S. et al. Autologous bone-marrow mesenchymal stem cell implantation and endothelial function in a rabbit ischemic limb model. PLoS One. 8, e67739. https://doi.org/10.1371/journal.pone.0067739 (2013).
Kim, M. S. et al. Priming with angiopoietin-1 augments the vasculogenic potential of the peripheral blood stem cells mobilized with granulocyte colony-stimulating factor through a novel Tie2/Ets-1 pathway. Circulation 120, 2240–2250. https://doi.org/10.1161/CIRCULATIONAHA.109.856815 (2009).
Fan, C. L. et al. Therapeutic neovascularization by autologous transplantation with expanded endothelial progenitor cells from peripheral blood into ischemic hind limbs. Acta Pharmacol. Sin. 26, 1069–1075. https://doi.org/10.1111/j.1745-7254.2005.00168.x (2005).
Vaughan, E. E. et al. Pretreatment of endothelial progenitor cells with osteopontin enhances cell therapy for peripheral vascular disease. Cell Transpl. 21, 1095–1107. https://doi.org/10.3727/096368911X623880 (2012).
Pyun, W. B. et al. Naked DNA expressing two isoforms of hepatocyte growth factor induces collateral artery augmentation in a rabbit model of limb ischemia. Gene Ther. 17, 1442–1452. https://doi.org/10.1038/gt.2010.101 (2010).
Tokunaga, N. et al. Adrenomedullin gene transfer induces therapeutic angiogenesis in a rabbit model of chronic hind limb ischemia: Benefits of a novel nonviral vector, gelatin. Circulation 109, 526–531. https://doi.org/10.1161/01.CIR.0000109700.81266.32 (2004).
Vincent, K. A. et al. Angiogenesis is induced in a rabbit model of hindlimb ischemia by naked DNA encoding an HIF-1alpha/VP16 hybrid transcription factor. Circulation 102, 2255–2261. https://doi.org/10.1161/01.cir.102.18.2255 (2000).
Shyu, K. G., Manor, O., Magner, M., Yancopoulos, G. D. & Isner, J. M. Direct intramuscular injection of plasmid DNA encoding angiopoietin-1 but not angiopoietin-2 augments revascularization in the rabbit ischemic hindlimb. Circulation 98, 2081–2087. https://doi.org/10.1161/01.cir.98.19.2081 (1998).
Kobayashi, K. et al. Combination of in vivo angiopoietin-1 gene transfer and autologous bone marrow cell implantation for functional therapeutic angiogenesis. Arterioscler. Thromb. Vasc. Biol. 26, 1465–1472. https://doi.org/10.1161/01.ATV.0000223865.64812.26 (2006).
Pinkenburg, O. et al. Recombinant adeno-associated virus-based gene transfer of cathelicidin induces therapeutic neovascularization preferentially via potent collateral growth. Hum Gene Ther. 20, 159–167. https://doi.org/10.1089/hum.2007.178 (2009).
Chen, F., Tan, Z., Dong, C. Y., Chen, X. & Guo, S. F. Adeno-associated virus vectors simultaneously encoding VEGF and angiopoietin-1 enhances neovascularization in ischemic rabbit hind-limbs. Acta Pharmacol. Sin. 28, 493–502. https://doi.org/10.1111/j.1745-7254.2007.00527.x (2007).
Zhang, C. et al. Angiopoiesis and bone regeneration via co-expression of the hVEGF and hBMP genes from an adeno-associated viral vector in vitro and in vivo. Acta Pharmacol. Sin. 31, 821–830. https://doi.org/10.1038/aps.2010.67 (2010).
Dai, Q. et al. Engineered zinc finger-activating vascular endothelial growth factor transcription factor plasmid DNA induces therapeutic angiogenesis in rabbits with hindlimb ischemia. Circulation 110, 2467–2475. https://doi.org/10.1161/01.CIR.0000145139.53840.49 (2004).
Jiang, H., Zhang, T. & Sun, X. Vascular endothelial growth factor gene delivery by magnetic DNA nanospheres ameliorates limb ischemia in rabbits. J. Surg. Res. 126, 48–54. https://doi.org/10.1016/j.jss.2005.01.005 (2005).
Cui, K. et al. Dual gene transfer of bFGF and PDGF in a single plasmid for the treatment of myocardial infarction. Exp. Ther. Med. 7, 691–696. https://doi.org/10.3892/etm.2014.1485 (2014).
Ohara, N. et al. Adenovirus-mediated ex vivo gene transfer of basic fibroblast growth factor promotes collateral development in a rabbit model of hind limb ischemia. Gene Ther. 8, 837–845. https://doi.org/10.1038/sj.gt.3301475 (2001).
Nishikage, S. et al. In vivo electroporation enhances plasmid-based gene transfer of basic fibroblast growth factor for the treatment of ischemic limb. J. Surg. Res. 120, 37–46. https://doi.org/10.1016/j.jss.2003.12.016 (2004).
Acknowledgements
The authors gratefully acknowledge funding through the DOD CDMRP (W81XWH-16-1-0580; W81XWH-16-1-0582) and the National Institutes of Health (1R01HL141761-01) to ABB.
Funding
Funding was received through the DOD CDMRP (W81XWH-16-1-0580; W81XWH-16-1-0582) and the National Institutes of Health (1R01HL141761-01).
Author information
Authors and Affiliations
Contributions
E.T. analyzed data and wrote/edited the manuscript. M.M., G.H., J.G., P.F., L.M., G.C., A.D.S. and R.S. performed experiments, data collection and analysis. A.B.B. conceived the original idea, analyzed experiments, and wrote/edited the manuscript.
Corresponding author
Ethics declarations
Competing interests
The authors (ET and ABB) have patented the technology presented in this manuscript. The other authors (MM, GH, JG, PF, LM, GC, ADS, and RS) on the study do not have competing interests.
Additional information
Publisher's note
Springer Nature remains neutral with regard to jurisdictional claims in published maps and institutional affiliations.
Supplementary Information
Rights and permissions
Open Access This article is licensed under a Creative Commons Attribution 4.0 International License, which permits use, sharing, adaptation, distribution and reproduction in any medium or format, as long as you give appropriate credit to the original author(s) and the source, provide a link to the Creative Commons licence, and indicate if changes were made. The images or other third party material in this article are included in the article's Creative Commons licence, unless indicated otherwise in a credit line to the material. If material is not included in the article's Creative Commons licence and your intended use is not permitted by statutory regulation or exceeds the permitted use, you will need to obtain permission directly from the copyright holder. To view a copy of this licence, visit http://creativecommons.org/licenses/by/4.0/.
About this article
Cite this article
Takematsu, E., Massidda, M., Howe, G. et al. Transmembrane stem factor nanodiscs enhanced revascularization in a hind limb ischemia model in diabetic, hyperlipidemic rabbits. Sci Rep 14, 2352 (2024). https://doi.org/10.1038/s41598-024-52888-6
Received:
Accepted:
Published:
DOI: https://doi.org/10.1038/s41598-024-52888-6
Comments
By submitting a comment you agree to abide by our Terms and Community Guidelines. If you find something abusive or that does not comply with our terms or guidelines please flag it as inappropriate.