Abstract
The aim of the study was to investigate the effect of the Fe3O4 nanoparticles (Fe-NPs) on the germination of sunflower seeds, early growth of seedlings and the concentration of selected elements in seedlings. The influence of constant magnetic fields in systems with and without Fe-NPs was investigated. Experiments were done on seeds subjected to germination under constant magnetic field (0 (control), 5, 25 and 120 mT) for 7 days in the presence of solution containing 0, 50 or 500 ppm Fe-NPs. No significant effect of Fe-NPs and the magnetic field on germination of seeds and the growth of seedlings has been demonstrated. In most cases, a decrease in germination parameters was observed. For the majority of samples the relative decrease in the concentrations of elements was demonstrated mainly for samples without Fe-NPs. Interestingly, a significant decrease in the concentrations of trivalent (including iron - Fe) and toxic elements in samples containing Fe-NPs in relation to control samples was observed. The authors suggest that in this case the binding (adsorption) of these elements in the roots and seeds of the sunflower by Fe-NPs took place. This explains the lower iron content in seedlings than in seeds prior to sowing.
Similar content being viewed by others
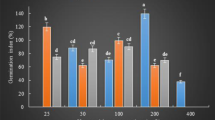
Introduction
Nanoparticles (NPs) have become a very attractive research object due to their unique physicochemical properties. There are many possibilities of their applications in industry, technology1 and medicine2,3 as well as in agriculture4. Agricultural applications include fertilizers and pesticides used to control pests or diseases5 as well as sensors for monitoring soil and plant quality6. Nano-layers of macro- and micronutrients or nano-carriers with nutrients are also used to enhance plant growth and protection7.
The effect of NPs on plants depends on their composition, concentration, size and physical properties as well as on plant species8. Rizwan et al.9 studied the effect of phosphorus nanoparticles (P-NPs) on cultivated plants whereas Siddiqui and Al-Whaibi10 applied SiO2 nanocrystals to tomato, both obtaining positive effects on plant growth, yield, mineral nutrition and photosynthesis. Ag-NPs influenced the mitotic index and root growth of Vicia faba seedlings11 and sorghum (bicolor Sorghum) and had growth-inhibiting effects depending on the concentration of NPs12. Mahmoodzadeh et al.13 treated canola seeds, Feizi et al.14 fennel seeds and Samadi et al.15 peppermint seeds (Mentha piperita) with TiO2-NPs and observed an increase in root length and a positive effect on the concentration of photosynthetic pigments.
The effect of toxicity was studied and observed for aluminum oxide (Al2O3), silicon dioxide (SiO2), magnetite (Fe3O4) and zinc oxide (ZnO) nanoparticles on Arabidopsis thaliana16 showing the highest phytotoxicity for ZnO-NPs. The influence of NPs (multilayer carbon nanotubes, aluminum, alumina, zinc and zinc oxide) on seed germination and plant roots growth (radish, rapeseed, ryegrass, lettuce, corn and cucumber) was investigated17. No NPs effect on germination of seeds was noted, however nano-Zn and nano-ZnO suspensions caused elongation of the roots of the studied plant species.
Zhu et al.18 investigated the effect of Fe3O4-NPs on pumpkins (Cucurbita maxima) and found that they can absorb, displace and accumulate nanoparticles in tissues.
The application of super paramagnetic iron oxide NPs (SPION)17 resulted in the increased chlorophyll levels in soybean leaves. Spherical Fe0-NPs and Fe3O4-NPs caused the inhibition of germination and leaf extension in soft wheat (Triticum vulgare Vill.)19. Fe-NPs positively influenced the germination and growth of wheat seedlings during exposure to drought and excessive salt - an increase in the weight and length of seedlings, shoots and roots was observed20.
Shrivastava et al.21 and Das et al.22 used aqueous suspension of non-paramagnetic nanoparticles of iron pyrite of (FeS2 + H2O) for the treatment of spinach seeds. In both cases aqueous suspension of nano iron pyrite, significantly increased the yield of spinach.
In the first experiment, seeds were soaked in an aqueous nanoparticle’s suspension and sown in the field. Seeds treated with water were used as control. The crop yields from iron-pyrite nanoparticle - treated seeds and control seeds were evaluated. The plants developed from nanoparticle - treated seeds exhibited significantly broader leaf morphology, larger leaf numbers, increased biomass; along with higher concentration of calcium (Ca), manganese (Mn) and zinc (Zn) in the leaves as compared to control.
To reduce the concentration of H2O2 generated during seed treatment with aqueous FeS2 nanoparticle’s suspension nano-ceria oxide CeO2 was added to the suspension. It was found that application of FeS2 resulted in obtaining leaves with increased chlorophyll and carbohydrate content which additionally were bigger than in the case of treatment with water (control), nano-CeO2 or FeS2 + CeO2. It was found that while nano-FeS2 hastened the germination and functioned as a seed vigor enhancer, nano-CeO2 had an opposite effect. The obtained results can be used to accelerate or delay germination, manipulate the weed population, store seeds in critical conditions and during other agro-technical treatments22.
The use of iron oxide nanomaterials in environmental protection technology has gained a lot of attention due to their unique properties: very small size, high surface area to volume ratio, surface modifiability, enhanced magnetic properties and excellent biocompatibility23. Celebi et al.24 used zero-valent iron nanoparticles (nZVI) to remove Ba2+ ions and Boparai et al.25 for removing Cd2+ ions, respectively. In order to remove water pollution with Cr6+, amorphous FeB alloy modified magnetite nanocomposites (Fe3O4-FeB) were used26. The removal of Pb2+ and Cd2+ traces from milk, human urine and blood plasma by selective ionic liquid ferrofluid in dispersive solid phase extraction was carried out by Ramandi and Shemirani27. Li and Zhang28 showed that Fe-NPs act as a sorbent and reductant when used to sequestrate Ni2+ in water.
For the removal of Pb2+ from the aqueous solution, synthesized kaolin-supported nanoscale zero-valent iron (K-nZVI)29 was used, which was also effective in removing Ni2+, Cd2+, Pb2+ (98.8%) and Cr (99.8%) from galvanic sewage.
Ge et al.30 used Fe3O4 polymer-modified magnetic nanoparticles to remove heavy metal ions (Cd2+, Zn2+, Pb2+ and Cu2+) from an aqueous solution.
Surface-active magnetic Fe3O4-NPs showed superparamagnetic behavior and a high effectiveness in removing toxic metal ions (Cr3+, Co2+, Ni2+, Cu2+, Cd2+, Pb2+ and As3+) and bacteria from contaminated water31 Badruddoza et al.32 produced carboxymethyl-β-cyclodextrin-modified Fe3O4 nanoparticles (CMCD-MNPs) to remove Cu2+ ions from the aqueous solution.
Bimetallic iron nanoparticles possess significantly better physical and chemical properties (including magnetism) and the ability to reduce various metals as compared to mono-metallic Fe-NPs33.
Ferromagnetic carbon-coated Fe-NPs showed the ability to remove more than 95% of Cr6+ in wastewater by means of physical adsorption on coating34 containing certain amount of a carboxylic functional group. Wang et al.35 developed a novel magnetic nanocomponent (core-shell) Fe3O4@SiO2 to remove heavy metal ions from aqueous media.
Studies on the use of Fe2O3-NPs for the selective removal of toxic metals (Cr6+, Cu2+ and Ni2+) from industrial wastewater have shown that the adsorption process is solution pH-dependent36. Liu et al.37 used Fe3O4-NPs coated with humic acid (Fe3O4/HA), to remove more than 99% of Hg2+ and Pb2+ and more than 95% of Cu2+ and Cd2+ from water. Savina et al.38 developed nanocomposite materials in which Fe-NPs were embedded in the walls of a macro-porous polymer. They showed excellent ability to remove trace concentrations of As3+ from the solution. Tang et al.39 created iron doped ordered mesoporous carbon (Fe/CMK-3) to remove Cr6+ (97%).
Corredor et al.40 used NPs to the targeted delivery of nourishing substances to treat plant diseases. The movement of Fe- and Cu-NPs in plant cells of pumpkin (Cucurbita pepo) was discovered.
Constant and variable magnetic and electric fields, ionizing radiation, microwave and laser radiation41,42,43 as well as magnetized water44 were frequently used for pre-sowing seed stimulation. Positive influence on germination and improvement of crop yield from seeds treated with electromagnetic (ELM) fields has been found45. According to Binhi46, the magneto-biological effects in a living cell are reproducible when the so-called “electromagnetic window” coincides with the “physiological” window. Positive or negative effects can be observed depending on the intensity, frequency, amplitude and the exposure time to field operation.
García Reina et al.47 suggested that the magnetic field interacts with ionic currents in the embryo’s cell membrane and, as a result of changes in ion concentration and osmotic pressure, regulates the penetration of water into seeds. Magnetic field can also affect the permeability of ion channels in the membrane and therefore the transport of substances into cells, it can enhance the formation of free radicals and induce changes in the concentration of hormones, enzymes as well as changes in DNA synthesis or transmission48. Under the influence of the magnetic field, the external electron shells of the water molecules and dissolved ions can became polarized. This can result in the changes of the conditions of ion hydration, which can serve as crystallization nuclei. Interaction of magnetic field with water in plant material can result in an increase in electrical conductivity and a decrease in the surface tension of water. Such an effect may be due to the Lorenz’s force exerted on the charged particles (ions) in water, which causes their temporary polarization corresponding to the external magnetic field49,50.
Research is rare in which seeds are exposed in magnetic fields throughout the period of germination and growth until seedlings are achieved51. Mroczek-Zdyrska et al.52 during the 14 day vegetation period exposed lupine seeds and emerging seedlings to constant magnetic field of 130 mT induction. The influence of a variable magnetic field with an induction of 0.2 mT and frequencies of 16 and 50 Hz on the seeds of narrow-leafed lupine (Lupinus angustifolius L.) was also investigated. Variable magnetic field for both frequencies reduced the content of chlorophyll and carotenoids in leaves53.
The analysis of the available literature showed that there were no reports on the impact of ferromagnetic particles on the germination of seeds and the growth of plants in the presence of constant magnetic fields. Not many reports exist concerning the concentrations of selected elements in the seeds and seedlings subjected to magnetic fields.
The aim of the study was to investigate the effect of aqueous solution of iron nanoparticles on the germination of sunflower Heliantus annuus L. seeds, the growth of seedlings and the content of selected elements in seedlings. The influence of constant magnetic fields on the above processes in systems with nanoparticles and without nanoparticles was investigated.
Results
The description of samples used in the experiment is presented in Table 1. The results of experiments are presented as relative values showing the effects of the used factors on the parameters of seedlings and the content of elements. The effect of magnetic field was calculated against samples K-0-500 and K-0-50, respectively. The effect of nanoparticles was shown in relation to the corresponding control samples P-5-0, P-25-0 and P-120-0. The synergistic effects from the combined factors were calculated against the K-0-0 control sample.
Additionally, for samples P-5-0, P-25-0 and P-120-0 (no Fe-NPs), the effect of the magnetic field was determined against K-0-0 sample and for samples K-0-500 and K-0-50 (no magnetic field) the effect of nanoparticles was estimated with relation to K-0-0 control sample.
The influence of Fe-NPs and magnetic field on sunflower seedlings is presented in Table 2. The highest germination capacity G was found for samples with no Fe-NPs (81–94%) and the lowest for those containing NPs at a concentration of 50 ppm for all magnetic field inductions as well as for the control sample K-0-50.
The highest mean water content Hss was found in seedlings without Fe-NPs (89–94%) for all inductions of magnetic field and the K-0-0 sample. The lowest water content occurred in the remaining samples (82–86%). A similar effect was observed for the mass of single seedling Wss. G parameter was lower in samples without nanoparticles but higher for samples containing 500 ppm of Fe-NPs as compared with 50 ppm Fe-NPs. Hss and Wss in the case of samples containing nanoparticles was also lower than in that without NPs. These parameters were lower at samples containing 500 ppm of NPs than for that with 50 ppm NPs.
In the case of relative values, for most of the samples subjected to magnetic field, GREL and HRELSS were lower than the control regardless of field induction and Fe-NPs concentration (between 0.9–1.0 - Table 2).
GREL below 1, indicates lower germination capacity for samples subjected to magnetic field apart from the P-120-50 sample. H REL SS above 1 were obtained only for samples P-5-500 (increase by 5.8%), P-25-0 (10.6%) and P-25-500 (5.8%). For other samples, the effect of all the factors used (magnetic field and Fe-NPs) produced values lower that for the respective control.
In the case of parameters determined in relation to the K-0-0 sample (the effect of combined factors), all relative parameters – GREL, HREL SS and WREL SS were lower than 1 but were the highest for samples without Fe-NPs. The exception was the WSS parameter for sample P-25-0. GREL was higher for the sample with 500 ppm Fe-NPs than for 50 ppm Fe-NPs samples for all magnetic field inductions. HREL SS and WREL SS were higher for a sample containing 50 ppm Fe-NPs as compared with sample containing 500 ppm Fe-NPs - unlike in the case of GREL.
The influence of the applied factors on the concentration of elements in sunflower seedlings is presented in Table 3. Elements are organized in a way that at the top of the table macro elements are placed followed by microelements and toxic elements. It is impossible to assign some of the elements to microelements or toxic elements because the biological effects depend strongly on their concentration.
The mostly pronounced increase in the concentration occurred for Ca (samples P-5-0: 1.23 times and P-5-50: 1.2 times) and Na (2.56–4.8) and a decrease in Ca (1.2–2.2 times; the highest for 500 ppm), K (1.13–1.2) and P (1.13–1.19). In the case of combined factors (magnetic field and Fe-NPs), there was an increase for Na (1.14–4.8) and a decrease in Ca (1.34–1.9), P (1.12–1.36) and K (1.13–1.18). The effect of the increase in the content of Zn (1.14 times for P-120-500), Mo (1.23 for P-5-0), Ca (1.2–1.23 for 5 mT and for both Fe-NPs concentrations) and Al (1.4–1.6 for 5 mT and 25 mT) in the seedlings upon magnetic field exposition was observed.
The relative decrease in Al (1.4 for P-25-50) was observed accompanied with an increase in Pb (1.13–1.43 mainly for B = 5 mT and 25 mT) and a decrease for Pb (1.4 for P- 120-500). It should be noted that for the majority of samples, the influence of the magnetic field was slight, and the relative decrease in the content of elements mainly concerns samples without Fe-NPs.
In the case of the influence of Fe-NPs on the concentration of examined elements, the relative decrease in all samples was found for Ni (1.2–4.7 times, max for K-25-500), Mo (2.7–4.1 max for 5 mT), Mn (1.6–1.27), Fe (2–2.9 max for P-5-500), Cu (1.3–1.03) and Al (10.3–34.6 max for 5 mT of both concentrations Fe-NPs).
The presence of Fe-NPs resulted in the decrease in Se concentration for all samples (range of 3.3–7.8 times; max for the magnetic field induction - 5 mT and both Fe-NPs concentrations). The decrease was also observed for P (1.18–1.36) and K (1.12–1.2). In the case of toxic elements, the decrease occurred for Hg (5.15–5.9 fold; max for 50 ppm of Fe-NPs), Cr (35–45 times; max for induction of 5 mT and 0 mT) and Pb (1.9–6.2; max for 500 ppm of Fe-NPs). The relative increase for all samples in the case of Cu (1.3 max for 25 mT), Na (2.56–4.8 max for 50 ppm) as well as for toxic elements Cd (1.16–1.6) and Sr (1.6–3.6 max for P-5-50) was observed.
The analysis of the effect of combined factors (Fe-NPs and magnetic field) for all samples showed the decrease for the following elements: Ni (1.36–6.3 times; max for Fe-NPs concentration 500 ppm), Mo (3–3.55), Mn (1.1–1.29), Fe (1.12–2.8; max for B = 0), Cu (1.14–1.22), Al (1.3 for P-25-0 to 20.3), Se (1.7–6 the majority within the limits of 6), P (1.15–1.36), Na (2.5–2.9), K (1.13–1.2) and Ca (1.4–1.9). For the toxic elements, the decrease occurred for Cr (37–43 fold), Hg (4.6–5.9 fold; max for all samples with Fe-NPs concentration of 50 ppm) and Pb (1.96–5.9 times; max for 500 ppm). The following relative increase rates values for all the examined samples were found: Na (2.9–4.8), Mg (1.1–1.14), and Al (2 for P-5-0). The relative increase rates for toxic elements were in the range of 1.17–4.14 for Sr and between 1.15–1.4 for Cd.
For samples P-5-0, P-25-0 and P-120-0 (no Fe-NPs) as well as K-0-500 and K-0-50 (no magnetic field) analyzed with respect to K-0-0 magnetic field produced the increase of the content of the following elements: Zn, S, Na, Pb (range between 1.1–1.15), Cd (1.24) and Al (2.06). The relative decrease of Ni concentration in the range of 2.04–4.23 as well as Fe, Al, Pb, Cd in the range between 1.01–1.04, Se (1.8) and Al (1.34) were observed.
In most cases, the effect of the magnetic field was insignificant - at a level of a few to a dozen or so percent. With the increase of the magnetic field induction, the number of elements which concentrations decreased in relation to K-0-0 control increased.
The presence of Fe-NPs resulted in an increase of Cd (rates between 1.31–1.41), Sr (1.54–2.53) and Na (2.87–4.8; higher for K-0-50). A decrease in concentration was observed for Al (17.73–19.57), Fe (2.68–2.83), Cr (41.16), Ni (5.62–5.85), Pb (2.45–5.13), Hg (5.13–5.45), Mo (3.34–3.55) and Se (5.82–6.02). Interestingly higher decrease was found for K-0–500 as compared to K-0-0. Hg concentration was close to detection limit of the method used, hence for the majority of cases its change was not statistically significant (Table 3). Interestingly, Fe content in seedlings germinating and growing in the presence of nanoparticles was lower than in the control sample without Fe-NPS (Table 3).
Table 4 shows the concentrations of elements in aerial parts of sunflower seedlings growing in soil or on blotting paper as well as in sunflower seeds. No Fe-NPs were used.
The increase in concentration for seedlings growing in the soil in relation to seedlings growing on paper was observed for Sr (increase rate of 4.15), Mo (3.63), Ca (4.29), Cr (2.03), Pb (2.4), K (3.44), Fe (2.68), Co (2.55) and Al (3.3). There was no decrease in the content of elements for any of the samples, which indicates that the elements were taken from the soil, not only from the seeds germinated on the paper. The increase in the concentration of elements for seedlings growing on blotting paper with respect to seeds was observed for Sr (20.85), Mo (3.52), Ba (9.22), Ca (2.98), Zn (3.54), Mn (2.6) and Mg (2.6) - Table 4. We are not able to explain that phenomenon at this stage. The decrease in elements’ concentrations was found for Cr (9.26), Pb (4.8), Co (2.16) and B (2.95-fold). The increase in the concentration of elements in seedlings growing in the soil in relation to seeds was as follows: Sr (86.48), Mo (12.76), Ca (12.79), Ba (15.93), Zn (4.27), Mn (3.7), Mg (2.9), K (5.56), Fe(3) (5.44) and Al (4.67). The decrease occurred for Pb, B (1.72) and Cr (4.57). The obtained results indicate a higher content of elements in seedlings growing in the soil than on blotting paper in relation to the composition of seeds.
Discussion
The most important elements (macroelements) related to sprouting and growth of seedlings include Ca, Mg, K, P and Na. Metals such as cobalt (Co), copper (Cu), chromium (Cr), iron (Fe), magnesium (Mg), manganese (Mn), molybdenum (Mo), nickel (Ni), selenium (Se) and zinc (Zn) are essential nutrients (micronutrients) required for various biochemical and physiological functions. However, the content of these metals is strictly limited due to their toxic nature, which depends on their content in a living organism. The third group consists of heavy metals toxic to living organisms in almost any quantity. These include cadmium (Cd), mercury (Hg) and lead (Pb) which are priority metals for public health.
Considering reduction of germination parameters of seeds and the growth of sunflower seedlings one can conclude that it was the result of a decrease in the content of the elements associated with this process (Ca, Mg, K, P and Na). It can be explained in terms of adsorption of these elements by Fe-NPs.
Iron nanoparticles (Fe-NPs) are known for their metal binding properties in diverse environmental systems. They have been successfully used for the purification of water and food products from metals and other toxic compounds. Their action in the above cases was explained by the adsorption of metals on nanoparticles. A similar mechanism is probably responsible for the observed changes in the content of elements in sunflower seedlings after the addition of Fe-NPs in our experiment. This can be supported by the fact that other authors observed the penetration of nanoparticles into the seed and root of plants54.
Upon exposition of the sunflower roots (Helianthus annuus L.) to three different concentrations of nano-maghemite (NM: Fe2O3, γ-Fe2O3)54 reduction of the hydraulic root conductivity and lower nutrient uptake were observed. A decrease in root functionality for water uptake to 57% was observed with respect to control value at a dose of 50 mg∙l−1 and was reduced to 26% at a dose twice as high. This is consistent with our findings where smaller water content was found in samples subjected to NPs.
The concentrations of Ca, K, Mg and S in the shoots were also reduced compared to the control plants, which also resulted in the reduction of chlorophyll pigments in the plant. The above confirms the results obtained in our study. The decrease in macronutrients as Ca (mostly pronounced at 500 ppm of Fe-NPs), K, P and Na at the level of several percent upon treatment with NPs was observed. The decrease of parameters GREL, HREL SS and WREL SS, indicate a negative impact on seed germination and development of seedlings (eventually no effect) for the majority of the cases when magnetic field of different induction and/or Fe-NPs at different concentrations were applied. In particular, interesting is the negative impact of the nanoparticles on control samples (samples containing Fe-NPs in relation to K-0-0). In that case, for higher concentrations of Fe-NPs in aqueous solution (500 ppm of Fe-NPs) a lower relative water content and relative mass of a single seedling was found.
Cifuentes et al.55 studied the effects of carbon-coated magnetic nanoparticles in the form of bio-ferrofluid on four plants: peas, sunflower, tomato and wheat. NPs were not detected outside the vascular tissues of the sunflower, unlike other plants. This means that the uptake of NPs by the roots of this plant is much slower than in other species. The above research showed that the sunflower had a lower radial ability to move bio-ferrofluid outside the vascular tissues, which might be the reason for low iron concentration in the plant tissue, similarly to our studies. In our case, the use of non-coated NPs (non-functionalized) was intended to facilitate their better penetration through the cell walls of the plant. Transmission electron microscopy images of wheat root cross-sections showed that Fe-NPs entered the root through the apoplastic path and were subsequently detected in the walls of the root epithelium cells56. A huge increment of Fe content in the wheat roots was observed. The authors did not detect NPs in the aboveground part (seedling), which would indicate that magnetite nanoparticles were not displaced by vascular tissues in wheat plants, which is opposite to the results found in our research.
López-Moreno et al.57 investigated tolerance of tomato Lycopersicum Solanum L. to CoFe2O4-NPs. In the above studies, seed exposure to NPs did not significantly affect the germination and growth of plants. The authors observed the absorption of Fe and Co into plant tissues and their effect on the concentration changes of Mg and Ca in the plant leaves.
Interestingly, much higher decrease in the content of trivalent and toxic elements occurred in our study for samples treated with the combined factors: magnetic field and Fe-NPs in comparison with treatment with Fe-NPs. The obtained results indicate the strengthening (synergistic) effect of the magnetic field in combination with nanoparticles. In our studies, a significant effect on the concentration of the elements is observed only for the samples treated with Fe-NPs. In the case of an increase in the content of elements effects of and magnetic field overlap only for Cd and Sr. In the case of a decrease in the content of elements in seedlings in relation to the control, the effect corresponds with the results of the Fe-NPs impact on the content of elements for all samples.
In conclusion, the analysis of agricultural crops exposed to NPs shows that they can be taken up by plants through roots or leaves9. However, the mechanisms of interaction between plants and NPs are still poorly understood and more research is needed on this subject, especially at the molecular level. Detailed studies to quantify the adsorption and uptake of NPs in different crops and under different growth conditions are necessary. In addition, there is an absence of legitimate soil-based studies with appropriate environmental exposure conditions.
Conclusions
-
1.
Magnetic fields had a small, mostly negative effect on germination process and germination parameters of sunflower seedlings. A clear negative effect of the presence of Fe-NPs in water on germination and sunflower seedling parameters was observed. Interestingly in many cases the decrease in the content of macro elements such as Ca, Mg, K, P and Na occurred (up to twofold).
-
2.
For microelements, especially trivalent ones, the largest decrease in concentration occurred for samples subjected to combined magnetic field and Fe-NPs and exclusively for Fe-NPs (Al: up to 20-fold, greater for 500 ppm than for 50 ppm, Cr: up to 40-fold, Fe: up to 3-fold, Ni: up to 5,9 fold and Mn: up to 1.3-fold.
-
3.
Application of the combined magnetic field and Fe-NPs as well as Fe-NPsalone resulted in a decrease in the content of toxic elements such as Hg and Pb (over 4 times).
-
4.
The obtained results may indicate the strengthening effect of the magnetic field in combination with Fe-NPs (synergistic effect).
-
5.
The obtained results may indicate that additional magnetization of Fe-NPs could increase the ability to adsorb selected elements in the roots and seeds of sunflower by iron nanoparticles.
Further studies on the composition of sunflower seedlings and roots treated with solutions with Fe-NPs of different concentrations are required. It would be desirable to determine the lowest concentrations (limit) at which the effects take place.
Materials and Methods
Sunflower seeds were subjected to germination in the presence of Fe-NPs solution at two concentrations: 50 ppm and 500 ppm (0.005% and 0.05%). Samples were exposed to constant magnetic fields with induction of B = 5, 25 and 120 mT produced by electromagnets. Samples with B = 0 were used as controls. Samples consisted of 30 seeds placed on wet blotting paper in transparent PVC containers. The construction of containers ensured constant humidity for germinating seeds and growing seedlings.
Before sowing, the seeds were sterilized in 0.65% sodium hypochlorite solution (Aquapool, Poland) for about 5 min, then rinsed in distilled water and dried on blotting paper. The containers and the germination system were sterilized in the same way. The blotting paper was irradiated with a UV lamp for 2–3 hours before being placed in the containers.
The volume of the solution was approximately 300 mL, therefore no water or Fe-NPs solution were added to the seeds during the entire growth process. During the experiment, the containers were covered with a glass lid providing light access. The experiment was carried out in a vegetation room with a constant temperature of about 23 °C ± 2 °C, where the light intensity was E = 500 ± 5 lux (at the level of germinating seeds) and a photo-period amounted to 16/8 hours for a day and night, respectively. The lighting from the white LED tape was directed downwards to seeds and seedlings. For each of the combinations, three 7-days series of measurements were carried out.
Additionally, seeds were germinated in pots with universal soil based on high peat with an admixture of sand (20%) with a pH between 5.5 and 6.5 (TORFIKS, Poland). Germination process was conducted in a vegetation room under the above-described temperature and light conditions. No Fe-NPs was used.
The germination capacity of sunflower seeds was defined as a final number of seedlings after 7 days. The mass of a single seedling was determined by dividing the mass of all seedlings in sample by their number. The water content was measured gravimetrically and given as an average value (in %) for the whole sample. After weighing seedlings were dried in an oven at 90 °C for 24 h, and then ground with a knife mill. Ground samples were than subjected to the measurement of the concentration of selected elements.
The content of elements in the roots of sunflower seedlings germinated on blotting paper (hydroponic culture) was not measured because of the difficulty of separating them from the paper. The authors of the work assumed that it is not possible to obtain an Fe-NPs - free (uncontaminated) root sample. The content of elements in seedlings obtained from pot tests was also measured only for the aboveground part of seedlings and not for their roots. The purpose of this measurement was to be able to compare the composition of seedlings from hydroponic culture and seedlings from pot tests and to compare with the concentration of elements in seeds.
Magnetic nanoparticles (Fe-NPs) were synthesized according to the method described by Khalafalla and Reimers58 involving co-precipitation of ferric and ferrous ions Fe2+/Fe3+ in molar ratio of 1:2. For this purpose, 5 ml of ammonium hydroxide (NH4OH) 56% (= 28% NH3) was slowly added to 5 ml of an aqueous solution containing 1.2 g of FeCl2*4aq and 2.4 g of FeCl3*6aq and solution was vigorously stirred. After the addition was completed, the solution was stirred for 10 min. The pellet was washed with 10 ml of solution containing 0.5 ml NH4OH and 9.5 ml of water and after about 15 min. decanted. The above procedure was repeated four times. The mass of the obtained nanoparticles was determined after drying.
To estimate the size of the nanoparticles, a scanning electron-ion microscope FEI Quanta 3D FEG was used. The nanoparticle suspension was applied to a copper microscope mesh with a polymer film. STEM imaging was performed along with the measurement of the distribution of the size of the observed Fe-NPs (Fig. 1). The measurement consisted of adjusting the ellipse with the five-point method, and then calculating the diameter corresponding to circular objects with the same surface area as the fitted ellipses. As a result the diameter and its distribution d: 12.50 ± 4.10 nm (Fig. 2) was obtained. The uncertainty given is a doubled standard deviation for the population of 140 objects tested.
The concentration of elements was measured by the ICP-OES method according to the procedure published in Sujak et al.59.
Statistical analysis was performed using ANOVA software and the Tukey test at the significance level of p < 0.05.
Data availability
Data will be available on request.
References
Santos C. S. et al. Industrial applications of nanoparticles – a prospective overview. Materials Today: Proceedings 2, 456–465, doi:10.131140/2.1.5100.6726 (2015).
Chudzik, B. et al. Effectiveness of magnetic fluid hyperthermia against Candida albicans cells. International Journal of Hyperthermia 32(8), 842–857 (2016).
Sharma, K. S. et al. Synthesis and characterization of monodispersed water dispersible Fe3O4 nanoparticles and in vitro studies on human breast carcinoma cell line under hyperthermia condition. Sci Rep 8, 14766, https://doi.org/10.1038/s41598-018-32934-w (2018).
Servin, A. D. et al. A review of the use of engineered nanomaterials to suppress plant disease and enhance crop yield. J Nanoparticle Res 17, 92–112 (2015).
Rai, M. & Ingle, A. Role of nanotechnology in agriculture with special reference to management of insect pests. Appl Microbiol Biotechnol 94, 287–293 (2012).
Servin, A. D. & White, J. C. Nanotechnology in agriculture: Next steps for understanding engineered nanoparticle exposure and risk. NanoImpact 1, 9–12 (2016).
Liu, R. & Lal, R. Potentials of engineered nanoparticles as fertilizers for increasing agronomic productions. Sci Total Environ 514, 131–139 (2015).
Ma, X., Geiser-Lee, J., Deng, Y. & Kolmakov, A. Interactions between engineered nanoparticles (ENPs) and plants: Phytotoxicity, uptake and accumulation. Sci Total Environ. 408, 3053–3061 (2010).
Rizwan, M. et al. Effect of metal and metal oxide nanoparticles on growth and physiology of globally important food crops: A critical review. J Hazardous Materials 322, 2–16 (2017).
Siddiqui, M. H. & Al-Whaibi, M. H. Role of nano-SiO2 in germination of tomato (Lycopersicum esculentum seeds Mill.). Saudi J Biol Sci 21, 13–17 (2014).
Abdel-Azeem, E. A. & Elsayed, B. A. Phytotoxicity of silver nanoparticles on Vicia faba seedlings. New York Science J. 6(12), 148–156 (2013).
Lee, W.-M., Kwak, J. I. & An, Y.-J. Effect of silver nanoparticles in crop plants Phaseolus radiatus and Sorghum bicolor: Media effect on phytotoxicity. Chemosphere 86, 491–499 (2012).
Mahmoodzadeh, H., Nabavi, M. & Kashefi, H. Effect of Nanoscale Titanium Dioxide Particles on the Germination and Growth of Canola (Brassica napus). Journal of Ornamental and Hortic. Plants 3(1), 25–32 (2013).
Feizi, H., Kamali, M., Jafari, L. & Moghaddam, P. R. Phytotoxicity and stimulatory impacts of nanosized and bulk titanium dioxide on fennel (Foeniculum vulgare Mill). Chemosphere 91, 506–511 (2013).
Samadi, N., Yahyaabadi, S. & Rezayatmand, Z. Effect of TiO2 and TiO2 Nanoparticle on Germination, Root and Shoot Length and Photosynthetic Pigments of Mentha Piperita. Int J Plant. Soil Sci 3(4), 408–418 (2014).
Lee, C. W. et al. Developmental phytotoxicity of metal oxide nanoparticles to Arabidopsis thaliana. Environ Toxicol Chem 29(3), 669–675 (2010).
Ghafariyan, M. H., Malakouti, M. J., Dadpour, M. R., Stroeve, P. & Mahmoudi, M. Effects of magnetic nanoparticles on soybean chlorophyll. Env Sci Technol 47, 10645–10652 (2013).
Zhu, H., Han, J., Xiao, J. Q. & Jin, Y. Uptake, translocation, and accumulation of manufactured iron oxide nanoparticles by pumpkin plants. J Env Monitoring 10, 713–717 (2008).
Lebedev, S. V., Korotkova, A. M. & Osipova, E. A. Influence of Fe0 Nanoparticles, Magnetite Fe3O4 Nanoparticles, and Iron (II) Sulfate (FeSO4) Solutions on the Content of Photosynthetic Pigments in Triticum vulgare. Russian. J Plant Physiol 61(4), 564–569 (2014).
Yasmeen, F., Raja, N. I., Razzaq, A. & Komatsu, S. Gel-free/label-free proteomic analysis of wheat shoot in stress tolerant varieties under iron nanoparticles exposure. Biochim Biophys Acta 1864, 1586–1598 (2016).
Srivastava, G. et al. Seed treatment with iron pyrite (FeS2) nanoparticles increases the production of spinach. RSC Advances 4(102), 58495–58504 (2014).
Das, C. K. et al. The seed stimulant effect of nano iron pyrite is compromised by nano cerium oxide: regulation by the trace ionic species generated in the aqueous suspension of iron pyrite. RSC Advances 6(71), 67029–67038 (2016).
Xu, P. et al. Use of iron oxide nanomaterials in wastewater treatment: A review. Sci Total Environ 424, 1–10 (2012).
Celebi, O., Uzumb, C., Shahwan, T. & Erten, H. N. A radiotracer study of the adsorption behavior of aqueous Ba2+ ions on nanoparticles of zero-valent iron. Journal of Hazardous Materials 148, 761–767 (2007).
Boparai, H. K., Joseph, M. & O’Carroll, D. M. Kinetics and thermodynamics of cadmium ion removal by adsorption onto nano zerovalent iron particles. Journal of Hazardous Materials 186, 458–465 (2011).
Shen, W., Mu, Y., Xiao, T. & Ai, Z. Magnetic Fe3O4-FeB nanocomposites with promoted Cr(VI) removal performance. Chem Eng J 285, 57–68 (2016).
Ramandi, N. F. & Shemirani, F. Selective ionic liquid ferrofluid based dispersive-solid phase extraction for simultaneous preconcentration/separation of lead and cadmium in milk and biological samples. Talanta 131, 404–411 (2015).
Li, X. & Zhang, W. Iron Nanoparticles: the Core−Shell Structure and Unique Properties for Ni(II) Sequestration. Langmuir 22(10), 4638–4642 (2006).
Zhang, X., Lin, S., Lu, X.-Q. & Chen, Z. Removal of Pb(II) from water using synthesized kaolin supported nanoscale zero-valent iron. Chem Eng J 163, 243–248 (2010).
Ge, F., Li, M.-M., Ye, H. & Zhao, B.-X. Effective removal of heavy metal ions Cd2+, Zn2+, Pb2+, Cu2+ from aqueous solution by polymer-modified magnetic nanoparticles. Journal of Hazardous Materials 211–212, 366–372 (2012).
Singh, S., Barick, K. C. & Bahadur, D. Surface engineered magnetic nanoparticles for removal of toxic metal ions and bacterial pathogens. J Hazardous Materials 192, 1539–1547 (2011).
Badruddoza, A. Z. M., Tay, A. S. H., Tan, P. Y., Hidajat, K. & Uddin, M. S. Carboxymethyl-β-cyclodextrin conjugated magnetic nanoparticles as nano-adsorbents for removal of copper ions: Synthesis and adsorption studies. Journal of Hazardous Materials 185, 1177–1186 (2011).
Liu, W.-J., Qian, T.-T. & Jiang, H. Bimetallic Fe nanoparticles: Recent advances in synthesis and application in catalytic elimination of environmental pollutants. Chem Eng J 236, 448–463 (2014).
Zhang, D. et al. Carbon-stabilized iron nanoparticles for environmental remediation. Nanoscale 2, 917–919 (2010).
Wang, J. et al. Amino-functionalized Fe3O4@SiO2 core–shell magnetic nanomaterial as a novel adsorbent for aqueous heavy metals removal. J Colloid Interface Sci 349, 293–299 (2010).
Hu, J., Chen, G. & Lo, I. M. C. Selective Removal of Heavy Metals from Industrial Wastewater Using Maghemite Nanoparticle: Performance and Mechanisms. J Env Eng 132(7), 709–715 (2006).
Liu, J. F., Zao, Z. S. & Jang, G. B. Coating Fe3O4 Magnetic Nanoparticles with Humic Acid for High Efficient Removal of Heavy Metals in Water. Environ. Sci. Technol. 42, 6949–6954 (2008).
Savina, I. N. et al. High Efficiency Removal of Dissolved As(III) using Iron Nanoparticle-Embedded Macroporous Polymer Composites. J Hazardous Materials 192, 31002–31008 (2011).
Tang, L. et al. Synergistic effect of iron doped ordered mesoporous carbon on adsorption-coupled reduction of hexavalent chromium and the relative mechanism study. Chemical Eng. J 239, 114–122 (2014).
Corredor, E. et al. Nanoparticle penetration and transport in living pumpkin plants: in situ subcellular identification. BMC Plant Biology 9(45), 1–11 (2009).
Galland, P. & Pazur, A. Magnetoreception in plants. J. Plant. Res. 118, 371–389 (2005).
Kornarzyński, K. & Łacek, R. Wpływ pola magnetycznego i elektrycznego na kiełkowanie nasion wybranych roślin kwiatowych. Inżynieria Rolnicza 5(80), 305–312 (2006).
Aladjadjiyan, A. 2010. Influence of stationary magnetic field on lentil seeds. Int. Agrophys. 24, 321–324 (2010).
Kornarzyński K., Pietruszewski S. Wpływ wody uzdatnianej magnetycznie na kiełkowanie nasion grochu i łubinu. Acta Agrophysica 192, 18(1), 101-111 2011.
Cakmak, T., Cakmak, Z. E., Dumlupinar, R. & Tekinay, T. Analysis of apoplastic and symplastic antioxidant system in shallot leaves: impacts of weak static electric and magnetic field. J Plant Physiol 169, 1066–1073 (2012).
Binhi, V. N. Magnetobiology Underlying Physical Problems, 1-471 (Academic Press, 2002).
Garciá Reina, F., Arza Pascual, L. & Almanza Fundora, I. Influence of a stationary magnetic field on water relations in lettuce seeds. Part II: Experimental results. Bioelectromagnetics 22, 596–602 (2001).
Strašák, L., Vetterl, V. & Šmarda, J. Effects of low-frequency magnetic fields on bacteria Escherichia coli. Bioelectrochemistry 55(1-2), 161–164 (2002).
Goldsworthy, A., Whitney, H. & Morris, E. Biological effects of physically conditioned water. Water Res. 33(7), 1618–1626 (1999).
Coey, J. M. D. & Cass, S. Magnetic water treatment. J. Magnetism Magnetic Materials 209, 71–74 (2000).
Pietruszewski, S. & Kornarzyński, K. Germination of wheat grain in an alternating magnetic field. Int Agrophys 15, 269–271 (2001).
Mroczek-Zdyrska, M., Kornarzyński, K., Pietruszewski, S. & Gagoś, M. Stimulation with a 130-mT magnetic field improves growth and biochemical parameters in lupin (Lupinus angustifolius L.). Turkish. J Biol 40, 699–705 (2016).
Mroczek-Zdyrska, M., Kornarzyński, K., Pietruszewski, S. & Gagoś, M. The effects of low-frequency magnetic field exposure on the growth and biochemical parameters in lupin (Lupinus angustifolius L.). Plant Biosystems 151(3), 504–511 (2017).
Martínez-Fernández, D., Barroso, D. & Komárek, M. Root water transport of Helianthus annuus L. under iron oxide nanoparticle exposure. Environ Sci Pollut Res 23(2), 1732–1741 (2015).
Cifuentes, Z. Absorption and translocation to the aerial part of magnetic carbon-coated nanoparticles through the root of different crop plants. J Nanobiotechnology 8, 1–8 (2010).
Iannone, M. F., Gropp, M. G., de Sousa, M. E., Fernández van Raap, M. B. & Benavides, M. P. Impact of magnetite iron oxide nanoparticles on wheat (Triticum aestivum L.) development: Evaluation of oxidative damage. Env. Exp Botany 131, 77–88 (2016).
López-Moreno, M. L. et al. Effect of cobalt ferrite (CoFe2O4) nanoparticles on the growth and development of Lycopersicon lycopersicum (tomato plants). Sci Total Environ 550, 45–52 (2016).
Khalafalla, S. E. & Reimers, G. W. Preparation of dilution-stable aqueous magnetic fluids. IEEE Trans Magn 16, 178–183 (1980).
Sujak, A., Wiącek, D., Jakubas, D., Komosa, A. & Kitowski, I. Mallards Anas platyrhynchos shot in Eastern Poland: ecological risk evaluated by analysis of trace elements in liver. 25(8), 2116–2132 Hum Ecol Risk Assessment https://doi.org/10.1080/10807039.2018.1489716 (2019).
Author information
Authors and Affiliations
Contributions
K.K. and A.S. wrote the main manuscript text, carried out experiments on seed germination and prepared samples for elemental analysis; G.C.Z. prepared magnetic nanoparticles and took part in manuscript writting, D.W. carried out mesurements of concentrations of elements. All authors reviewed the manuscript.
Corresponding author
Ethics declarations
Competing interests
The authors declare no competing interests.
Additional information
Publisher’s note Springer Nature remains neutral with regard to jurisdictional claims in published maps and institutional affiliations.
Rights and permissions
Open Access This article is licensed under a Creative Commons Attribution 4.0 International License, which permits use, sharing, adaptation, distribution and reproduction in any medium or format, as long as you give appropriate credit to the original author(s) and the source, provide a link to the Creative Commons license, and indicate if changes were made. The images or other third party material in this article are included in the article’s Creative Commons license, unless indicated otherwise in a credit line to the material. If material is not included in the article’s Creative Commons license and your intended use is not permitted by statutory regulation or exceeds the permitted use, you will need to obtain permission directly from the copyright holder. To view a copy of this license, visit http://creativecommons.org/licenses/by/4.0/.
About this article
Cite this article
Kornarzyński, K., Sujak, A., Czernel, G. et al. Effect of Fe3O4 nanoparticles on germination of seeds and concentration of elements in Helianthus annuus L. under constant magnetic field. Sci Rep 10, 8068 (2020). https://doi.org/10.1038/s41598-020-64849-w
Received:
Accepted:
Published:
DOI: https://doi.org/10.1038/s41598-020-64849-w
This article is cited by
-
Advances in transport and toxicity of nanoparticles in plants
Journal of Nanobiotechnology (2023)
-
Nanoengineered particles for sustainable crop production: potentials and challenges
3 Biotech (2023)
-
Impact of magnetic field on the translocation of iron oxide nanoparticles (Fe3O4) in barley seedlings (Hordeum vulgare L.)
3 Biotech (2023)
-
Improved iron use efficiency in tomato using organically coated iron oxide nanoparticles as efficient bioavailable Fe sources
Chemical and Biological Technologies in Agriculture (2022)
-
Nano-priming as emerging seed priming technology for sustainable agriculture—recent developments and future perspectives
Journal of Nanobiotechnology (2022)
Comments
By submitting a comment you agree to abide by our Terms and Community Guidelines. If you find something abusive or that does not comply with our terms or guidelines please flag it as inappropriate.