Abstract
Blindness leads to substantial enhancements in many auditory abilities, and deficits in others. It is unknown how severe visual losses need to be before changes in auditory abilities occur, or whether the relationship between severity of visual loss and changes in auditory abilities is proportional and systematic. Here we show that greater severity of visual loss is associated with increased auditory judgments of distance and room size. On average participants with severe visual losses perceived sounds to be twice as far away, and rooms to be three times larger, than sighted controls. Distance estimates for sighted controls were most accurate for closer sounds and least accurate for farther sounds. As the severity of visual impairment increased, accuracy decreased for closer sounds and increased for farther sounds. However, it is for closer sounds that accurate judgments are needed to guide rapid motor responses to auditory events, e.g. planning a safe path through a busy street to avoid collisions with other people, and falls. Interestingly, greater visual impairment severity was associated with more accurate room size estimates. The results support a new hypothesis that crossmodal calibration of audition by vision depends on the severity of visual loss.
Similar content being viewed by others
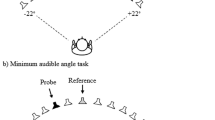
Introduction
The World Health Organization (WHO) estimated that globally 188.5 million people have mild visual loss, 217 million have moderate to severe losses, and 36 million are blind1. It has now been well established that full blindness (total visual loss or light perception only) can result in enhancement of certain auditory spatial abilities and worsening of others (for reviews, see2,3,4,5,6,7). For example, blindness often results in dramatic improvements in echolocation skills4,8 and the ability to locate sounds in azimuth (left-front-right judgments)9,10, but leads to significantly poorer ability to judge the vertical position of sounds11,12, or judge sound position with respect to external acoustic landmarks13. It has been suggested that the changes underlying enhanced performance are fundamentally related to adaptations within the occipital cortex, where visual areas of the brain are recruited to process auditory inputs in the event of visual loss5,14,15. However, the underlying principles of what drives changes in auditory abilities following visual loss are not well understood. It is not yet known how severe the visual loss needs to be before significant alterations in auditory abilities are observed, or whether the relationship between severity of visual loss and changes in auditory abilities is systematic. If it is systematic, then people with more modest visual losses should exhibit smaller changes in auditory abilities than those with more severe visual losses.
Previous work showed that compared to sighted controls, individuals with total vision loss estimate near sound sources to be farther away and estimate farther sound sources to be closer16,17, demonstrating the critical role that vision plays in calibrating auditory space18. A number of studies have also shown that partial visual deprivation affects auditory localization abilities. Myopic (short-sighted) participants are more accurate than sighted controls for azimuthal localization19 and echolocation, and show greater sensitivity to echoic spatial cues20. Myopic and amblyopic participants have been reported to show significantly smaller self-positioning errors using sound to assess their position in a room than sighted controls21. Also, partially sighted participants self-reported better azimuthal sound localization and improved abilities to follow speech when switching between different talkers22.
Although partial loss of vision entails an increased reliance on hearing for awareness and safety, communication and interaction, and for enjoyment through sound23, it is not known how hearing is affected by the severity of partial visual loss. We explored this by obtaining judgments of the distance of sound sources and of room size using sound for participants with a range of severities of visual loss, to test the hypothesis that crossmodal calibration is dependent on the magnitude of the sensory loss. It was hypothesized that systematic increases in auditory judgments of distance and room size would be associated with the severity of visual loss, with greater estimates associated with more severe visual loss. To investigate whether this was true, and whether it generalized across different room environments and stimuli, participants were tested in virtual anechoic and reverberant rooms, using speech, music and noise stimuli. The different stimuli were chosen as they varied in their spectro-temporal characteristics. It was hypothesized that in a virtual reverberant room, estimates of distance and room size would be greater than in the virtual anechoic room, as research suggests that greater reverberation is associated with increased perceived distance24 and room size estimates25,26 for normally sighted participants. It was hypothesized that estimates would be more veridical for speech27 than for noise28 or music, as normally sighted participants were previously shown to be able to utilize their familiarity with the acoustic characteristics of speech to give more veridical distance estimates29.
Methods
Participants
The participants were recruited from Sankara Nethralaya Eye Hospital in Chennai, India. Fifty six participants took part. They were categorized into four groups according to their visual acuities, with category boundaries chosen to include a wide range of visual losses. Group 1 consisted of sighted controls (n = 18, 13 females, mean age 21.1 yrs, range 20–25 yrs, LogMAR [Logarithm of the Minimum Angle of Resolution, used to estimate visual acuity] = 0), group 2 had mild visual impairment (n = 16, 4 females, mean age 21.7 yrs, range 18–31 yrs, LogMAR 0.1–1), group 3 had mid-range visual impairment (n = 12, 7 females, mean age 21.1 yrs, range 17–28 yrs, LogMAR 1.1 to 2.9), and group 4 had severe visual impairment (n = 10, 5 females, mean age 21.9 yrs, range 18–31 yrs, LogMAR 3–4). See Supplementary Table S1 online for a summary of group characteristics. Some of the participants with mild visual impairment wore corrective glasses. For reference, legal blindness in the United Kingdom and United States of America is defined as having a better eye visual acuity when using a corrective lens equal to or worse than LogMAR = 1. One-way ANOVAs showed no significant differences in age between the four groups (p > 0.05), no significant differences in duration of visual loss across the groups with visual losses (p > 0.05), and no significant differences in age of onset of visual loss across the groups with visual losses (p > 0.05). Hearing for all participants was normal or near-normal, as indicated by pure-tone-average better-ear hearing thresholds ≤25 dB HL across 0.5, 1, 2, 4, 6, and 8 kHz30. The study and methods followed the tenets of the Declaration of Helsinki, and informed consent was obtained from participants after the nature and possible consequences of participation were explained. Approval for the experiments was given by the Anglia Ruskin University Ethics Panel.
Apparatus and data acquisition
Testing took place in a quiet room. Stimuli were presented using Sennheiser HD219 headphones. The stimuli were generated using methods described in our previous studies16,17,31,32,33. A virtual room measuring 35 m (length) x 30 m (width) x 10 m (height), that was either anechoic (where only sound level information about distance was available24,28,34) or reverberant (where both level and echoic direct-to-reverberant energy ratio [DRR] distance cues were available33,35,36), was generated using an image-source model (ISM)37. The reverberation time T60 (the time taken for the sound level to fall by 60 dB) was 700 ms, as used in previous studies16,35. The ISM produced a room impulse response (RIR) between a virtual auditory source and a receiver positioned a set virtual distance away. Convolution of the RIR and an auditory stimulus generated a virtual sample of the stimulus heard in the virtual room at the simulated distance. Stimuli were simulated at 1 m height, at 0° elevation and 0° azimuth relative to the front of the head. The participant was in the near-left corner of the room, facing forwards at 30° relative to the longer wall (for a schematic of the simulated room, see Fig. 1).
Participants heard speech, music, and broadband noise stimuli, as used in our previous work16,31. Speech stimuli had a duration of 1.5 s, were sampled at 22.05 kHz, and were single sentences spoken at a conversational level by a male, selected randomly from the Bench–Kowal–Bamford corpus16,31,38,39. Music stimuli had a duration of 7.3 s, were sampled at 22.05 kHz, and played by a jazz trio of piano, bass and drums16,31,40. Broadband (0.6–12 kHz) noise stimuli had a duration of 90 ms and a rise/fall time of 10 ms, and were sampled at 44.1 kHz10,16,31,32.
Virtual distances of the sound source were 1.22, 1.72, 2.44, 3.45, 4.88, 6.90, 9.75 and 13.79 m, chosen to match those used in previous work16,28,31. Sounds were presented at 65 dB SPL (unweighted) for a simulated distance of 1 m and the level decreased as the virtual distance was increased. Stimuli were spatially rendered by convolving the direct sound component with a non-individualized head-related transfer function (HRTF) using publicly available measurements41. These HRTFs were used in previous studies of auditory distance judgments16,31,32,42.
Procedures
Participants wore blindfolds throughout testing8,43, and were instructed to imagine that they were seated in a rectangular room of a certain size. They were told that sounds would be played from loudspeakers from a range of distances and they would be required to estimate by verbal report the distance of each sound in meters and centimeters. After hearing a series of sounds, they would be requested to estimate the room size by reporting the length, width, and height of the room in meters and centimeters.
Single sounds were presented in a pseudo-random order at the various simulated distances. At the end of each block of trials (80 stimulus presentations; see below), the participant gave an estimate of the room size. All responses were recorded by the experimenter. Participants did not receive training, were not given feedback, and were not limited in their response time.
For each block of trials, the stimulus type (speech, music, or noise) and room condition (anechoic or reverberant) were held constant. There were 10 repetitions for each of the 8 simulated distances for each block and 3 stimulus types x 2 room conditions = 6 blocks, with 480 trials in total. Data collection occurred in a single session lasting approximately 1 hour and 40 minutes, during which the blocks were presented in a random order.
Results
Distance estimates
Figure 2 shows scatter plots of signed error values for judged distance against best eye visual acuity, for a subset of the tested distances of 1.22, 3.45, and 13.79 m. Pearson correlation (r) values are reported in the bottom-right corner of each panel and are shown for all distances used in Table 1. Correlations were significant in all cases, with the exception of the distance of 1.22 m for the noise conditions, and 1.72 m for the reverberant noise condition.
Individual judged distance signed error values plotted against best eye visual acuity. Rows of panels from top to bottom show results for simulated anechoic and reverberant rooms for speech, music, and noise. The left, middle, and right panels show results for a subset of simulated distances of 1.22, 3.45 and 13.79 m, respectively. LogMAR = 0 represents best visual acuity and 4 the worst. Linear fits to the data are shown by dotted lines. Perfect performance is indicated by the long-dashed lines. Pearson r values are reported in the bottom-right corner of each panel (in this and subsequent figures and Table 1, ns = non-significant, * = p<0.05, ** = p<0.01).
The top panels of Fig. 3 show judged auditory distance plotted as a function of source distance for each condition, on log-log co-ordinates. Geometric mean estimates (following previous work28) are indicated by open symbols for sighted participants and filled symbols for VI participants. Consistent with the literature7, the sighted group judged sound source distance most accurately for the closest sounds and least accurately for farther sounds. On average, the severe VI group showed the opposite pattern, judging sound source distance most accurately for farther sounds and least accurately for closer sounds. All VI groups estimated the sound source distances to be farther than did the sighted controls. Estimated distance systematically increased with severity of visual loss, with the severe VI group on average judging sounds to be twice as far away as for the sighted controls. For statistical comparison purposes, and similar to the procedure used by Voss et al.44 for analyzing spatial estimates, the distances tested were grouped into near (1.22–1.72 m), middle (2.44–4.88 m), and far (6.90–13.79 m) distances. Near space was previously described by Zahorik, et al.45 as less than 1.9 m from the participant, and it includes the region where tactile feedback might be used to calibrate audition as an alternative to visual feedback. Grouping the distances also allowed us to compare the results to previous work showing that absolute judgments of distance for speech and music were relatively accurate at near and middle distances and less accurate at far distances16,29. A mixed-model ANOVA on the logarithms of the estimated distances with distance, reverberation time (i.e. anechoic vs. reverberant virtual room) and stimulus as within-subjects factors and visual status as a between-subjects factor showed main effects of visual status (F(3, 52) = 6.6, p < 0.001), distance (F(2, 104) = 303.5, p < 0.001), and stimulus (F(2, 104) = 60.0, p < 0.001), and significant interactions between stimulus and visual status (F(6, 104) = 7.61, p < 0.001), distance and visual status (F(6, 104) = 4.81, p < 0.001), stimulus and distance (F(4, 208) = 34.9, p < 0.001), stimulus and reverberation time (F(2, 104) = 9.9, p < 0.001), stimulus reverberation time and visual status (F(6, 104) = 2.7, p < 0.05), and stimulus and reverberation time and distance (F(4, 208) = 2.9, p < 0.05). No other main effects or interactions were significant (all p > 0.05). Post hoc tests with Bonferroni correction showed that in all conditions the severe VI group judged sounds to be significantly farther than the sighted group, except for the speech and noise stimuli at near distances. The mid-range VI group judged sounds to be significantly farther than the sighted group in all conditions for near and middle distances only, except for the reverberant music stimuli, and the anechoic music stimuli at near distance only. The mild VI group judged sounds to be significantly farther than the sighted group for anechoic music and noise stimuli at near and middle distances only.
Judged auditory distance plotted as a function of virtual sound source distance (top panels), and veridical perceived distances (distances at which the perceived distance most accurately matched the simulated distance) for each condition (bottom panel). In the top panels, geometric mean estimates are indicated by open (sighted participants), and closed grey (mild VI), red (mid-range VI), and blue (severe VI) symbols. The left, middle and right panels show results for speech, music and noise stimuli, respectively. The upper and lower panels show results for the reverberant and anechoic rooms, respectively. Perfect performance is indicated by the dashed lines. Error bars represent ±1 standard error across participants, and are not shown when smaller than the symbol size. The lower panel shows veridical perceived distances in each condition for each group; values were obtained from the intercept between lines of best fit for each condition (not shown) and the line for perfect performance in the top panels.
The bottom panel of Fig. 3 shows veridical perceived distances, which are the distances at which the perceived distance matched the simulated distance, for each stimulus condition and severity. Values were obtained from the intercept between lines of best fit for each condition (not shown) and the lines showing perfect performance in the top panels. Smaller values of veridical distance indicate that the distances at which the reported distance and simulated distance matched were nearer to the participant. Veridical perceived distances increased as severity of visual loss increased, with the farthest veridical perceived distances occurring for the group with severe visual impairment in anechoic and reverberant music conditions. These results show that groups with severe visual losses judged farther sound distances most accurately than sighted participants, whereas the opposite was the case for close sounds.
Room size estimates
Figure 4 shows individual room size estimates plotted against best eye visual acuity. r values are shown in the bottom-right corner of each panel. The room size was consistently underestimated. Correlations between room size estimates and best eye visual acuity were positive and significant for all conditions (p < 0.05), indicating that participants with greater visual losses made larger room size estimates.
Individual judged room size estimates plotted against best eye visual acuity. Rows of panels from top to bottom show results for speech, music, and noise. The left and right columns show results for anechoic and reverberant simulated rooms, respectively. Linear fits to the data are shown by dotted lines. Veridical room size is indicated by the long-dashed lines (room size = 10500 m3). r values are shown in the bottom-right corner of each panel.
Discussion
The results show that the greater the severity of visual loss, the larger the auditory judgments of distance and room size, with the largest perceived distances and room sizes associated with the most severe visual impairment. On average the group with severe visual losses judged sounds to be twice as far away, and rooms to be three times larger, than sighted controls. For normally sighted participants, auditory space is assumed to be calibrated primarily by vision46, which is considerably more accurate than audition47,48,49. Internal representations of auditory space are constantly updated using motor and visual feedback to align auditory and visual representations of space50. This leads to the prediction that altering the accuracy of the visual signal will affect how auditory space is calibrated. The results demonstrate that full blindness is not necessary for judged auditory distance and room size to be affected by visual loss, and that changes in auditory perception are systematic and related to the severity of visual loss. The current results support the hypothesis that crossmodal calibration is dependent on the magnitude of the sensory loss. Consistent with this idea, the visually impaired participants, who lacked an intact visual signal to calibrate auditory space accurately, perceived sound sources as being at greater distances than sighted controls and judged the room to be larger. As a consequence, visually impaired individuals show a deficit in accuracy for judging the distance of closer sounds. However, it is in this region of space that accurate judgments of distance are required to guide rapid motor responses in order to react to auditory events, particularly threatening or interesting sounds51. For example, the increase in perceived distance of a sound source would make it harder for somebody with visual loss to plan a safe path through a busy street to avoid collisions and falls.
On average, normally sighted controls made more veridical judgments for stimuli that were near 1 m, and systematically underestimated the distance of sound sources that were farther away, agreeing with previous research conducted in real and virtual rooms7,45. Consistent with previous work16, estimates by normally sighted controls tended to be more veridical for speech and music than for noise stimuli, for which judgments tended to be most accurate for sounds at near and middle distances. For speech stimuli, normally sighted controls were most accurate for near and middle distances, and less accurate for far distances, as observed in a previous study29. However, it is possible that the relatively long durations of the speech and music stimuli, which were chosen to match those used in previous work, may have affected the results. This needs further examination. Similar performance was found for anechoic and reverberant conditions, suggesting that the presence of the DRR cue did not affect spatial judgments for all groups of participants. This may be due to the comparatively short room reverberation time used in the study (700 ms), as previous studies have shown that increasing the room reverberation time increases perceived distance judgments24 and room size estimates25,26 for normally sighted participants. This also needs further examination.
The current study investigated how judged auditory distance and room size were affected by visual loss when both auditory level and DRR cues were present (reverberant condition) and when the level cue in isolation was available (anechoic condition). Although level is generally the dominant cue used for making distance judgments, DRR has been shown to be as effective as level when making distance judgments in highly reverberant environments for farther source distances for normally sighted participants only33. Also, fully blind participants often develop enhanced abilities to process echoes32,52. Further work is needed to establish whether severity of visual loss affects the ability to process the DRR cue in isolation. By making DRR the only useful distance cue, for example by equalizing or roving the overall stimulus level, it would be possible to examine whether the severity of visual loss is correlated with the ability to process reverberation information when making distance judgments.
Another area for future work regards the relevance of the onset of visual deprivation and the duration of visual loss for crossmodal plasticity and calibration. Full visual loss in early life during a critical period of development is likely to affect the spatial abilities of the remaining senses53. This is the basis of the crossmodal calibration hypothesis, that loss of vision at an early age affects the spatial calibration of the remaining senses47. Investigations into the influence of the age of onset of visual loss on audition have generally been confined to testing participants with full blindness54. Further work is needed to establish how the onset of visual loss affects the spatial processing abilities of individuals with partial visual losses.
Absolute judgments of auditory distance require well-calibrated mapping between internal spatial representations and the distance to external sound-producing objects7. Auditory representations of distance are likely to be relatively coarse compared to visual representations16,55,56, and the calibration of internal auditory spatial maps is generally thought to be realized using visual signals, due to the greater accuracy of visual spatial information than auditory information46. Tactile feedback in peripersonal space might be used to calibrate audition as an alternative to vision11, and audiomotor feedback from the systematic changes in sound cues arising from the movement of the head and body has also been suggested as a means to calibrate internal representations of sound azimuth in the absence of vision12. In contrast to the relatively accurate localization performance for azimuth observed in blind individuals9,42, the current results for partially sighted participants are consistent with previous work reporting that absolute distance accuracy is worse for blind than for sighted controls in peripersonal space16,17,57,58. Taken together, these results suggest that if vision is absent or degraded, tactile feedback is insufficient to calibrate auditory distance in peripersonal space, corresponding to “near” space in the current results. The ability to judge the distance of a single, static sound source is relatively poor compared to the ability to judge the azimuth of a sound in normally sighted individuals59 as well as blind individuals5,16. Although it is unclear why tactile and audiomotor feedback appear to be sufficient to calibrate internal representations of sound azimuth but not distance, one possible explanation is that small changes in sound azimuth cues are easier to detect than small changes in auditory distance cues, rendering azimuth cues but not distance cues amenable to calibration by tactile or audiomotor feedback. However, further investigation is needed. The current results suggest that partial visual loss affects the calibration of internal representations of auditory distance, and that the magnitude of change in the internal spatial representations of distance stemming from visual loss is dependent on the severity of visual loss.
A potential limitation of the experiment concerns the choice of response measure (verbally stating an estimated distance). The use of this measure depends on the participants’ ability to judge distance in standard measurement units. This raises the possibility that differences between groups may not be related to auditory perception, but rather to the ability to accurately estimate distance. It is possible that the outcomes would have differed if a more objective and potentially less biased spatial measure was used60,61. However, we have previously shown that visual loss does not affect distance judgments using a non-auditory and non-visual task, in which blind participants and sighted controls were required to walk over various distances (2, 5, or 10 m). Performance was similar across groups, indicating that the general ability to estimate distances was similar for sighted and fully blind participants16. These results suggest that visual loss does not affect the ability to estimate distance. Measurements such as walking to the perceived location of the sound source have been used as an alternative to reporting perceived distance49. Loomis et al.49 reported that judgments of auditory distance by normally sighted participants made using verbal report or walking responses were generally concordant, with less variability associated with the walking responses, suggesting that the findings of the current study would likely be similar if walking responses instead of verbal responses were used to measure perceived auditory distance.
The literature indicates reasonable agreement between subjective and objective measures of auditory perception for partially sighted participants22, suggesting that changes in audition shown under laboratory conditions can reflect judgments in real-life situations. Thus, the effects of degree of visual loss on distance and room size judgments shown here would probably be associated with differences in the ability to use sound effectively when navigating through the world. A key open question regarding the effect of sensory deprivation on the remaining senses is how residual vision may affect the development of cross-modal plasticity54. For individuals with remaining pattern vision, like those who took part in the current study, competitive visual inputs may affect the degree to which cross-modal recruitment occurs. The current results suggest that changes in auditory distance and room size perception are systematically related to the severity of visual impairment, raising the possibility that cross-modal recruitment may also be proportional to the severity of visual impairment. This remains to be investigated.
Data availability
The datasets generated during and analyzed during the current study are available from the corresponding author on reasonable request.
References
Bourne, R. R. et al. Magnitude, temporal trends, and projections of the global prevalence of blindness and distance and near vision impairment: A systematic review and meta-analysis. Lancet Glob. Health 5, e888–e897 (2017).
Voss, P., Collignon, O., Lassonde, M. & Lepore, F. Adaptation to sensory loss. Wiley Interdiscip. Rev. Cogn. Sci. 1, 308–328 (2010).
Kolarik, A. J., Cirstea, S., Pardhan, S. & Moore, B. C. J. A summary of research investigating echolocation abilities of blind and sighted humans. Hear. Res. 310, 60–68 (2014).
Thaler, L. & Goodale, M. A. Echolocation in humans: An overview. Wiley Interdiscip. Rev. Cogn. Sci. 7, 382–393 (2016).
Voss, P. Auditory spatial perception without vision. Front. Psychol. 7, 1960 (2016).
Collignon, O., Voss, P., Lassonde, M. & Lepore, F. Cross-modal plasticity for the spatial processing of sounds in visually deprived subjects. Exp. Brain. Res. 192, 343–358 (2009).
Kolarik, A. J., Moore, B. C. J., Zahorik, P., Cirstea, S. & Pardhan, S. Auditory distance perception in humans: A review of cues, development, neuronal bases and effects of sensory loss. Atten. Percept. Psychophys. 78, 373–395 (2016).
Kolarik, A. J., Scarfe, A. C., Moore, B. C. J. & Pardhan, S. Blindness enhances auditory obstacle circumvention: Assessing echolocation, sensory substitution, and visual-based navigation. PloS one 12, e0175750 (2017).
Lessard, N., Pare, M., Lepore, F. & Lassonde, M. Early-blind human subjects localize sound sources better than sighted subjects. Nature 395, 278–280 (1998).
Voss, P. et al. Early- and late-onset blind individuals show supra-normal auditory abilities in far-space. Curr. Biol. 14, 1734–1738 (2004).
Zwiers, M., Van Opstal, A. & Cruysberg, J. A spatial hearing deficit in early-blind humans. J. Neurosci. 21, 141–145 (2001).
Lewald, J. Vertical sound localization in blind humans. Neuropsychologia 40, 1868–1872 (2002).
Vercillo, T., Tonelli, A. & Gori, M. Early visual deprivation prompts the use of body-centered frames of reference for auditory localization. Cognition 170, 263–269 (2018).
Pascual-Leone, A., Amedi, A., Fregni, F. & Merabet, L. B. The plastic human brain cortex. Annu. Rev. Neurosci. 28, 377–401 (2005).
Amedi, A., Merabet, L. B., Bermpohl, F. & Pascual-Leone, A. The occipital cortex in the blind: Lessons about plasticity and vision. Curr. Dir. Psychol. Sci. 14, 306–311 (2005).
Kolarik, A. J., Pardhan, S., Cirstea, S. & Moore, B. C. J. Auditory spatial representations of the world are compressed in blind humans. Exp. Brain. Res. 235, 597–606 (2017).
Kolarik, A. J., Cirstea, S., Pardhan, S. & Moore, B. C. J. An assessment of virtual auditory distance judgements among blind and sighted listeners. Proc. Mtgs. Acoust. 19, 050043 (2013).
Axelrod, S. Effects of early blindness. New York: American Foundation for the Blind (1959).
Dufour, A. & Gérard, Y. Improved auditory spatial sensitivity in near-sighted subjects. Cognit. Brain. Res. 10, 159–165 (2000).
Després, O., Candas, V. & Dufour, A. Auditory compensation in myopic humans: involvement of binaural, monaural, or echo cues? Brain Res. 1041, 56–65 (2005).
Després, O., Candas, V. & Dufour, A. The extent of visual deficit and auditory spatial compensation: Evidence from self-positioning from auditory cues. Cognit. Brain. Res. 23, 444–447 (2005).
Kolarik, A. J. et al. Partial visual loss affects self-reports of hearing abilities measured using a modified version of the Speech, Spatial, and Qualities of Hearing Questionnaire. Front. Psychol. 8, 561 (2017).
Audira. What our hearing does for us, <audira.org.uk/en/modernising-attitudes-to-hearing-care/item/17-what-our-hearing-does-for-us> (2018).
Mershon, D. H. & King, L. E. Intensity and reverberation as factors in the auditory perception of egocentric distance. Atten. Percept. Psychophys. 18, 409–415 (1975).
Mershon, D. H., Ballenger, W. L., Little, A. D., McMurtry, P. L. & Buchanan, J. L. Effects of room reflectance and background noise on perceived auditory distance. Perception 18, 403–416 (1989).
Etchemendy, P. E. et al. Auditory environmental context affects visual distance perception. Sci. Rep. 7, 7189 (2017).
Gardner, M. B. Distance estimation of 0° or apparent 0°‐oriented speech signals in anechoic space. J. Acoust. Soc. Am. 45, 47–53 (1969).
Zahorik, P. Assessing auditory distance perception using virtual acoustics. J. Acoust. Soc. Am. 111, 1832–1846 (2002).
Brungart, D. S. & Scott, K. R. The effects of production and presentation level on the auditory distance perception of speech. J. Acoust. Soc. Am. 110, 425–440 (2001).
British Society of Audiology. Pure-tone air-conduction and bone-conduction threshold audiometry with and without masking. (British Society of Audiology, 2011).
Kolarik, A. J., Pardhan, S., Cirstea, S. & Moore, B. C. J. Using acoustic information to perceive room size: Effects of blindness, room reverberation time, and stimulus. Perception 42, 985–990 (2013).
Kolarik, A. J., Cirstea, S. & Pardhan, S. Evidence for enhanced discrimination of virtual auditory distance among blind listeners using level and direct-to-reverberant cues. Exp. Brain. Res. 224, 623–633 (2013).
Kolarik, A. J., Cirstea, S. & Pardhan, S. Discrimination of virtual auditory distance using level and direct-to-reverberant ratio cues. J. Acoust. Soc. Am. 134, 3395–3398 (2013).
Coleman, P. D. An analysis of cues to auditory depth perception in free space. Psychol. Bull. 60, 302–315 (1963).
Zahorik, P. Direct-to-reverberant energy ratio sensitivity. J. Acoust. Soc. Am. 112, 2110–2117 (2002).
Bronkhorst, A. W. & Houtgast, T. Auditory distance perception in rooms. Nature 397, 517–520 (1999).
Lehmann, E. A. & Johansson, A. M. Prediction of energy decay in room impulse responses simulated with an image-source model. J. Acoust. Soc. Am. 124, 269–277 (2008).
Akeroyd, M. A., Gatehouse, S. & Blaschke, J. The detection of differences in the cues to distance by elderly hearing-impaired listeners. J. Acoust. Soc. Am. 121, 1077–1089 (2007).
Bench, J., Kowal, A. & Bamford, J. The BKB (Bamford-Kowal-Bench) sentence lists for partially-hearing children. Br. J. Audiol. 13, 108–112 (1979).
Moore, B. C. J., Füllgrabe, C. & Stone, M. A. Determination of preferred parameters for multichannel compression using individually fitted simulated hearing aids and paired comparisons. Ear. Hear. 32, 556–568 (2011).
Gardner, W. G. & Martin, K. D. HRTF measurements of a KEMAR. J. Acoust. Soc. Am. 97, 3907–3908 (1995).
Voss, P., Lepore, F., Gougoux, F. & Zatorre, R. J. Relevance of spectral cues for auditory spatial processing in the occipital cortex of the blind. Front. Psychol. 2, 48 (2011).
Kolarik, A. J., Scarfe, A. C., Moore, B. C. J. & Pardhan, S. Echoic sensory substitution information in a single obstacle circumvention task. PLOS ONE 11, e0160872 (2016).
Voss, P., Tabry, V. & Zatorre, R. J. Trade-off in the sound localization abilities of early blind individuals between the horizontal and vertical planes. J. Neurosci. 35, 6051–6056 (2015).
Zahorik, P., Brungart, D. S. & Bronkhorst, A. W. Auditory distance perception in humans: A summary of past and present research. Acta Acust. United Ac. 91, 409–420 (2005).
Gori, M., Sandini, G., Martinoli, C. & Burr, D. C. Impairment of auditory spatial localization in congenitally blind human subjects. Brain 137, 288–293 (2014).
Gori, M., Sandini, G., Martinoli, C. & Burr, D. Poor haptic orientation discrimination in nonsighted children may reflect disruption of cross-sensory calibration. Curr. Biol. 20, 223–225 (2010).
Da Silva, J. A. Scales for perceived egocentric distance in a large open field: Comparison of three psychophysical methods. Am. J. Psychol. 98, 119–144 (1985).
Loomis, J. M., Klatzky, R. L., Philbeck, J. W. & Golledge, R. G. Assessing auditory distance perception using perceptually directed action. Atten. Percept. Psychophys. 60, 966–980 (1998).
Lewald, J. Exceptional ability of blind humans to hear sound motion: Implications for the emergence of auditory space. Neuropsychologia 51, 181–186 (2013).
Serino, A., Canzoneri, E. & Avenanti, A. Fronto-parietal areas necessary for a multisensory representation of peripersonal space in humans: An rTMS study. J. Cognitive Neurosci. 23, 2956–2967 (2011).
Dufour, A., Després, O. & Candas, V. Enhanced sensitivity to echo cues in blind subjects. Exp. Brain. Res. 165, 515–519 (2005).
Thinus-Blanc, C. & Gaunet, F. Representation of space in blind persons: Vision as a spatial sense? Psychol. Bull. 121, 20–42 (1997).
Voss, P. Brain (re) organization following visual loss. Wiley Interdiscip. Rev. Cogn. Sci. 10, e1468 (2019).
Milne, J. L., Goodale, M. A. & Thaler, L. The role of head movements in the discrimination of 2-D shape by blind echolocation experts. Atten. Percept. Psychophys. 76, 1828–1837 (2014).
Kolarik, A. J., Scarfe, A. C., Moore, B. C. J. & Pardhan, S. An assessment of auditory-guided locomotion in an obstacle circumvention task. Exp. Brain. Res. 234, 1725–1735 (2016).
Wanet, M. & Veraart, C. Processing of auditory information by the blind in spatial localization tasks. Atten. Percept. Psychophys. 38, 91–96 (1985).
Macé, M. J. M., Dramas, F. & Jouffrais, C. in Computers Helping People with Special Needs: 13th International Conference, ICCHP 2012 (eds Miesenberger, K., Karshmer, A., Penaz, P. & Zagler, W. W.) 636-643 (Springer-Verlag, 2012).
Middlebrooks, J. C. & Green, D. M. Sound localization by human listeners. Annu. Rev. Psychol. 42, 135–159 (1991).
Cattaneo, Z., Fantino, M., Tinti, C., Silvanto, J. & Vecchi, T. Crossmodal interaction between the mental number line and peripersonal haptic space representation in sighted and blind individuals. Atten. Percept. Psychophys. 72, 885–890 (2010).
Pasqualotto, A., Taya, S. & Proulx, M. J. Sensory deprivation: Visual experience alters the mental number line. Behav Brain Res 261, 110–113 (2014).
Acknowledgements
This research was supported by the Vision and Eye Research Institute, School of Medicine at Anglia Ruskin University. We thank Ms. Saranya, who helped in the data collection.
Author information
Authors and Affiliations
Contributions
A.J.K., B.C.J.M., S.C. and S.P. designed the study. A.J.K. wrote the manuscript draft. S.P. coordinated the study. A.J.K., S.G. and R.R. carried out data collection. A.J.K. and S.C. analysed data. All authors revised the manuscript draft and gave final approval of the manuscript draft.
Corresponding author
Ethics declarations
Competing interests
The authors declare no competing interests.
Additional information
Publisher’s note Springer Nature remains neutral with regard to jurisdictional claims in published maps and institutional affiliations.
Supplementary information
Rights and permissions
Open Access This article is licensed under a Creative Commons Attribution 4.0 International License, which permits use, sharing, adaptation, distribution and reproduction in any medium or format, as long as you give appropriate credit to the original author(s) and the source, provide a link to the Creative Commons license, and indicate if changes were made. The images or other third party material in this article are included in the article’s Creative Commons license, unless indicated otherwise in a credit line to the material. If material is not included in the article’s Creative Commons license and your intended use is not permitted by statutory regulation or exceeds the permitted use, you will need to obtain permission directly from the copyright holder. To view a copy of this license, visit http://creativecommons.org/licenses/by/4.0/.
About this article
Cite this article
Kolarik, A.J., Raman, R., Moore, B.C.J. et al. The accuracy of auditory spatial judgments in the visually impaired is dependent on sound source distance. Sci Rep 10, 7169 (2020). https://doi.org/10.1038/s41598-020-64306-8
Received:
Accepted:
Published:
DOI: https://doi.org/10.1038/s41598-020-64306-8
This article is cited by
-
Development and validation of a questionnaire for assessing visual and auditory spatial localization abilities in dual sensory impairment
Scientific Reports (2024)
-
Experimental Approaches to Studies of the Localization of Sound Sources by Distance
Neuroscience and Behavioral Physiology (2024)
-
Early visual deprivation disrupts the mental representation of numbers in visually impaired children
Scientific Reports (2022)
-
Partial visual loss disrupts the relationship between judged room size and sound source distance
Experimental Brain Research (2022)
Comments
By submitting a comment you agree to abide by our Terms and Community Guidelines. If you find something abusive or that does not comply with our terms or guidelines please flag it as inappropriate.