Abstract
Despite decades of research, our understanding of the processes controlling late-stage atherosclerotic plaque stability remains poor. A prevailing hypothesis is that reducing inflammation may improve advanced plaque stability, as recently tested in the Canakinumab Anti-inflammatory Thrombosis Outcome Study (CANTOS) trial, in which post-myocardial infarction subjects were treated with an IL-1β antibody. Here, we performed intervention studies in which smooth muscle cell (SMC) lineage-tracing Apoe-/- mice with advanced atherosclerosis were treated with anti-IL-1β or IgG control antibodies. Surprisingly, we found that IL-1β antibody treatment between 18 and 26 weeks of Western diet feeding induced a marked reduction in SMC and collagen content, but increased macrophage numbers in the fibrous cap. Moreover, although IL-1β antibody treatment had no effect on lesion size, it completely inhibited beneficial outward remodeling. We also found that SMC-specific knockout of Il1r1 (encoding IL-1 receptor type 1) resulted in smaller lesions nearly devoid of SMCs and lacking a fibrous cap, whereas macrophage-selective loss of IL-1R1 had no effect on lesion size or composition. Taken together, these results show that IL-1β has multiple beneficial effects in late-stage murine atherosclerosis, including promotion of outward remodeling and formation and maintenance of an SMC- and collagen-rich fibrous cap.
This is a preview of subscription content, access via your institution
Access options
Access Nature and 54 other Nature Portfolio journals
Get Nature+, our best-value online-access subscription
$29.99 / 30 days
cancel any time
Subscribe to this journal
Receive 12 print issues and online access
$209.00 per year
only $17.42 per issue
Buy this article
- Purchase on Springer Link
- Instant access to full article PDF
Prices may be subject to local taxes which are calculated during checkout






Similar content being viewed by others
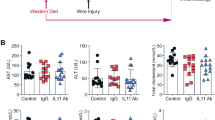
References
Yahagi, K. et al. Pathophysiology of native coronary, vein graft, and in-stent atherosclerosis. Nat. Rev. Cardiol. 13, 79–98 (2016).
Kolodgie, F. D. et al. Pathologic assessment of the vulnerable human coronary plaque. Heart 90, 1385–1391 (2004).
Virmani, R., Kolodgie, F. D., Burke, A. P., Farb, A. & Schwartz, S. M. Lessons from sudden coronary death: A comprehensive morphological classification scheme for atherosclerotic lesions. Arterioscler. Thromb. Vasc. Biol. 20, 1262–1275 (2000).
Davies, M. J., Richardson, P. D., Woolf, N., Katz, D. R. & Mann, J. Risk of thrombosis in human atherosclerotic plaques: Role of extracellular lipid, macrophage, and smooth muscle cell content. Br. Heart J. 69, 377–381 (1993).
Gomez, D. & Owens, G. K. Smooth muscle cell phenotypic switching in atherosclerosis. Cardiovasc. Res. 95, 156–164 (2012).
Cherepanova, O. A. et al. Activation of the pluripotency factor OCT4 in smooth muscle cells is atheroprotective. Nat. Med. 22, 657–665 (2016).
Shankman, L. S. et al. KLF4-dependent phenotypic modulation of smooth muscle cells has a key role in atherosclerotic plaque pathogenesis. Nat. Med. 21, 628–637 (2015).
Feil, S. et al. Transdifferentiation of vascular smooth muscle cells to macrophage-like cells during atherogenesis. Circ. Res. 115, 662–667 (2014).
Sui, Y. et al. IKKbeta links vascular inflammation to obesity and atherosclerosis. J. Exp. Med. 211, 869–886 (2014).
Tabas, I. & Glass, C. K. Anti-inflammatory therapy in chronic disease: Challenges and opportunities. Science 339, 166–172 (2013).
Libby, P., Tabas, I., Fredman, G. & Fisher, E. A. Inflammation and its resolution as determinants of acute coronary syndromes. Circ. Res. 114, 1867–1879 (2014).
Hansson, G. K., Libby, P. & Tabas, I. Inflammation and plaque vulnerability. J. Intern. Med. 278, 483–493 (2015).
Kirii, H. et al. Lack of interleukin-1beta decreases the severity of atherosclerosis in ApoE-deficient mice. Arterioscler. Thromb. Vasc. Biol. 23, 656–660 (2003).
Chi, H., Messas, E., Levine, R. A., Graves, D. T. & Amar, S. Interleukin-1 receptor signaling mediates atherosclerosis associated with bacterial exposure and/or a high-fat diet in a murine apolipoprotein E heterozygote model: Pharmacotherapeutic implications. Circulation 110, 1678–1685 (2004).
Isoda, K. et al. Lack of interleukin-1 receptor antagonist modulates plaque composition in apolipoprotein E-deficient mice. Arterioscler. Thromb. Vasc. Biol. 24, 1068–1073 (2004).
Bhaskar, V. et al. Monoclonal antibodies targeting IL-1 beta reduce biomarkers of atherosclerosis in vitro and inhibit atherosclerotic plaque formation in Apolipoprotein E-deficient mice. Atherosclerosis 216, 313–320 (2011).
Ridker, P. M. et al. Effects of interleukin-1beta inhibition with canakinumab on hemoglobin A1c, lipids, C-reactive protein, interleukin-6, and fibrinogen: A phase IIb randomized, placebo-controlled trial. Circulation 126, 2739–2748 (2012).
Ridker, P. M., Thuren, T., Zalewski, A. & Libby, P. Interleukin-1beta inhibition and the prevention of recurrent cardiovascular events: Rationale and design of the Canakinumab Anti-inflammatory Thrombosis Outcomes Study (CANTOS). Am. Heart J. 162, 597–605 (2011).
Ridker, P. M. et al. Antiinflammatory therapy with canakinumab for atherosclerotic disease. N. Engl. J. Med. 377, 1119–1131 (2017).
Ridker, P. M. et al. Relationship of C-reactive protein reduction to cardiovascular event reduction following treatment with canakinumab: A secondary analysis from the CANTOS randomised controlled trial. Lancet 391, 319–328 (2018).
Chamberlain, J. et al. Interleukin-1 regulates multiple atherogenic mechanisms in response to fat feeding. PLoS One 4, e5073 (2009).
Shemesh, S. et al. Interleukin-1 receptor type-1 in non-hematopoietic cells is the target for the pro-atherogenic effects of interleukin-1 in apoE-deficient mice. Atherosclerosis 222, 329–336 (2012).
Alexander, M. R., Murgai, M., Moehle, C. W. & Owens, G. K. Interleukin-1beta modulates smooth muscle cell phenotype to a distinct inflammatory state relative to PDGF-DD via NF-kappaB-dependent mechanisms. Physiol. Genom. 44, 417–429 (2012).
Libby, P., Warner, S. J. & Friedman, G. B. Interleukin 1: A mitogen for human vascular smooth muscle cells that induces the release of growth-inhibitory prostanoids. J. Clin. Invest. 81, 487–498 (1988).
Loppnow, H. & Libby, P. Proliferating or interleukin 1-activated human vascular smooth muscle cells secrete copious interleukin 6. J. Clin. Invest. 85, 731–738 (1990).
Baylis, R. A., Gomez, D. & Owens, G. K. Shifting the focus of preclinical, murine atherosclerosis studies from prevention to late-stage intervention. Circ. Res. 120, 775–777 (2017).
Gomez, D., Shankman, L. S., Nguyen, A. T. & Owens, G. K. Detection of histone modifications at specific gene loci in single cells in histological sections. Nat. Methods 10, 171–177 (2013).
Mombaerts, P. et al. RAG-1-deficient mice have no mature B and T lymphocytes. Cell 68, 869–877 (1992).
Amento, E. P., Ehsani, N., Palmer, H. & Libby, P. Cytokines and growth factors positively and negatively regulate interstitial collagen gene expression in human vascular smooth muscle cells. Arterioscler. Thromb. 11, 1223–1230 (1991).
Alexander, M. R. et al. Genetic inactivation of IL-1 signaling enhances atherosclerotic plaque instability and reduces outward vessel remodeling in advanced atherosclerosis in mice. J. Clin. Invest. 122, 70–79 (2012).
Tacke, F. et al. Immature monocytes acquire antigens from other cells in the bone marrow and present them to T cells after maturing in the periphery. J. Exp. Med. 203, 583–597 (2006).
Potteaux, S. et al. Suppressed monocyte recruitment drives macrophage removal from atherosclerotic plaques of Apoe-/- mice during disease regression. J. Clin. Invest. 121, 2025–2036 (2011).
Haka, A. S., Potteaux, S., Fraser, H., Randolph, G. J. & Maxfield, F. R. Quantitative analysis of monocyte subpopulations in murine atherosclerotic plaques by multiphoton microscopy. PLoS One 7, e44823 (2012).
Abdulaal, W. H. et al. Characterization of a conditional interleukin-1 receptor 1 mouse mutant using the Cre/LoxP system. Eur. J. Immunol. 46, 912–918 (2016).
Clausen, B. E., Burkhardt, C., Reith, W., Renkawitz, R. & Forster, I. Conditional gene targeting in macrophages and granulocytes using LysMcre mice. Transgenic Res. 8, 265–277 (1999).
Vadiveloo, P. K., Stanton, H. R., Cochran, F. W. & Hamilton, J. A. Interleukin-4 inhibits human smooth muscle cell proliferation. Artery 21, 161–181 (1994).
Hawker, K. M., Johnson, P. R., Hughes, J. M. & Black, J. L. Interleukin-4 inhibits mitogen-induced proliferation of human airway smooth muscle cells in culture. Am. J. Physiol. 275, L469–477 (1998).
Jenkins, S. J. et al. Local macrophage proliferation, rather than recruitment from the blood, is a signature of TH2 inflammation. Science 332, 1284–1288 (2011).
Jenkins, S. J. et al. IL-4 directly signals tissue-resident macrophages to proliferate beyond homeostatic levels controlled by CSF-1. J. Exp. Med. 210, 2477–2491 (2013).
Sica, A. & Mantovani, A. Macrophage plasticity and polarization: In vivo veritas. J. Clin. Invest. 122, 787–795 (2012).
Zhao, X. N., Li, Y. N. & Wang, Y. T. Interleukin-4 regulates macrophage polarization via the MAPK signaling pathway to protect against atherosclerosis. Genet. Mol. Res. 15, gmr.15017348 (2016).
Tabas, I. Macrophage death and defective inflammation resolution in atherosclerosis. Nat. Rev. Immunol. 10, 36–46 (2010).
Cai, B. et al. MerTK cleavage limits proresolving mediator biosynthesis and exacerbates tissue inflammation. Proc. Natl Acad. Sci. USA 113, 6526–6531 (2016).
van Gils, J. M. et al. The neuroimmune guidance cue netrin-1 promotes atherosclerosis by inhibiting the emigration of macrophages from plaques. Nat. Immunol. 13, 136–143 (2012).
Wanschel, A. et al. Neuroimmune guidance cue Semaphorin 3E is expressed in atherosclerotic plaques and regulates macrophage retention. Arterioscler. Thromb. Vasc. Biol. 33, 886–893 (2013).
Robbins, C. S. et al. Local proliferation dominates lesional macrophage accumulation in atherosclerosis. Nat. Med. 19, 1166–1172 (2013).
Duewell, P. et al. NLRP3 inflammasomes are required for atherogenesis and activated by cholesterol crystals. Nature 464, 1357–1361 (2010).
Baylis, R. A., Gomez, D., Mallat, Z., Pasterkamp, G. & Owens, G. K. The CANTOS trial: One important step for clinical cardiology but a giant leap for vascular biology. Arterioscler. Thromb. Vasc. Biol. 37, e174–e177 (2017).
Harrington, R. A. Targeting inflammation in coronary artery disease. N. Engl. J. Med. 377, 1197–1198 (2017).
Ibanez, B. & Fuster, V. CANTOS: A gigantic proof-of-concept trial. Circ. Res. 121, 1320–1322 (2017).
Nov, O. et al. Interleukin-1beta regulates fat-liver crosstalk in obesity by auto-paracrine modulation of adipose tissue inflammation and expandability. PLoS One 8, e53626 (2013).
Howard, C. et al. Safety and tolerability of canakinumab, an IL-1beta inhibitor, in type 2 diabetes mellitus patients: A pooled analysis of three randomised double-blind studies. Cardiovasc. Diabetol. 13, 94 (2014).
Everett, B. M. et al. Anti-inflammatory therapy with canakinumab for the prevention and management of diabetes. J. Am. Coll. Cardiol. 71, 2402–2404 (2018).
Topol, E. J. Failing the public health—Rofecoxib, Merck, and the FDA. N. Engl. J. Med. 351, 1707–1709 (2004).
Morton, A. C. et al. The effect of interleukin-1 receptor antagonist therapy on markers of inflammation in non-ST elevation acute coronary syndromes: The MRC-ILA Heart Study. Eur. Heart J. 36, 377–384 (2015).
Interleukin 1 Genetics Consortium. Cardiometabolic effects of genetic upregulation of the interleukin 1 receptor antagonist: a Mendelian randomisation analysis. Lancet Diabetes Endocrinol. 3, 243–253 (2015).
Wirth, A. et al. G12-G13-LARG-mediated signaling in vascular smooth muscle is required for salt-induced hypertension. Nat. Med. 14, 64–68 (2008).
Dobin, A. et al. STAR: Ultrafast universal RNA-seq aligner. Bioinformatics 29, 15–21 (2013).
Liao, Y., Smyth, G. K. & Shi, W. featureCounts: An efficient general purpose program for assigning sequence reads to genomic features. Bioinformatics 30, 923–930 (2014).
Love, M. I., Huber, W. & Anders, S. Moderated estimation of fold change and dispersion for RNA-seq data with DESeq2. Genome Biol. 15, 550 (2014).
Luo, W., Friedman, M. S., Shedden, K., Hankenson, K. D. & Woolf, P. J. GAGE: Generally applicable gene set enrichment for pathway analysis. BMC Bioinforma. 10, 161 (2009).
Acknowledgements
We thank the Owens laboratory members for their input. We thank P. Libby, M. Nahrendorf, and P. Swirski for their constructive discussion during the project completion. We thank E. Greene for her assistance in generating the SMC-specific Il1r1 knockout mice, A. Nguyen for performing retro-orbital injections, and M. McCanna for technical support. We thank the University of Pittsburgh Center for Biologic Imaging for their assistance with confocal microscopy. This work was supported by NIH grants R01 HL121008, R01 HL132904, and R01 HL136314 to G.K.O. D.G. was supported by Scientific Development Grant 15SDG25860021 from the American Heart Association. R.A.B. was supported by NIH grant F30 HL136188. B.G.D. was supported by a Predoctoral Fellowship from the American Heart Association (14PRE20380659). C.S.H. was supported by K22HL117917. The generation of the IL1R1fl/fl mice was funded by FP7/EU Project MUGEN (MUGEN LSHG-CT-2005-005203) to W.M. and by MRC research (G0801296) to E.P.
Author information
Authors and Affiliations
Contributions
D.G. and G.K.O. originally conceived of and designed the experiments. D.G. performed experiments, analyzed data, performed statistical analysis, and wrote the manuscript. R.A.B. designed and performed experiments, analyzed data, and contributed to manuscript writing. B.G.D. performed staining and data analysis. A.A.C.N. performed staining and data analysis of necrotic core and Ter119 data. G.F.A. analyzed the RNA-seq dataset. S.M. performed staining and analyzed data. C.S.H. performed calcification staining. A.W., W.M., S.E.F., and E.P. provided Il1r1fl/fl mice. G.J.R. helped with the monocyte trafficking assay. H.G. provided the IL-1β antibody and the IgG control and helped in experimental design. G.K.O. supervised the project. All co-authors read the manuscript.
Corresponding author
Ethics declarations
Competing interests
H.G. is a full-time employee of Novartis Pharma AG, Basel.
Additional information
Publisher’s note: Springer Nature remains neutral with regard to jurisdictional claims in published maps and institutional affiliations.
Supplementary information
Supplementary Text and Figures
Supplementary Figures 1–12 and Supplementary Table 1
Rights and permissions
About this article
Cite this article
Gomez, D., Baylis, R.A., Durgin, B.G. et al. Interleukin-1β has atheroprotective effects in advanced atherosclerotic lesions of mice. Nat Med 24, 1418–1429 (2018). https://doi.org/10.1038/s41591-018-0124-5
Received:
Accepted:
Published:
Issue Date:
DOI: https://doi.org/10.1038/s41591-018-0124-5
This article is cited by
-
Suppression of IL-1β promotes beneficial accumulation of fibroblast-like cells in atherosclerotic plaques in clonal hematopoiesis
Nature Cardiovascular Research (2024)
-
Cholesterol lowering depletes atherosclerotic lesions of smooth muscle cell-derived fibromyocytes and chondromyocytes
Nature Cardiovascular Research (2024)
-
Expansion of fibroblast-like cells may explain the CANTOS meta-analysis findings for patients with clonal hematopoiesis
Nature Cardiovascular Research (2024)
-
Programmed death of macrophages in atherosclerosis: mechanisms and therapeutic targets
Nature Reviews Cardiology (2024)
-
Identification of key pyroptosis-related genes and microimmune environment among peripheral arterial beds in atherosclerotic arteries
Scientific Reports (2024)