Abstract
Many patients with end-stage heart disease die because of the scarcity of donor hearts. A total artificial heart (TAH), an implantable machine that replaces the heart, has so far been successfully used in over 1,700 patients as a temporary life-saving technology for bridging to heart transplantation. However, after more than six decades of research on TAHs, a TAH that is suitable for destination therapy is not yet available. High complication rates, bulky devices, poor durability, poor biocompatibility and low patient quality of life are some of the major drawbacks of current TAH devices that must be addressed before TAHs can be used as a destination therapy. Quickly emerging innovations in battery technology, wireless energy transmission, biocompatible materials and soft robotics are providing a promising opportunity for TAH development and might help to solve the drawbacks of current TAHs. In this Review, we describe the milestones in the history of TAH research and reflect on lessons learned during TAH development. We summarize the differences in the working mechanisms of these devices, discuss the next generation of TAHs and highlight emerging technologies that will promote TAH development in the coming decade. Finally, we present current challenges and future perspectives for the field.
Key points
-
After decades of research on total artificial hearts, only two devices are clinically available as a bridge to transplantation therapy; a total artificial heart suitable for destination therapy has not yet been developed.
-
Currently available total artificial hearts have major drawbacks, including bulkiness, limited durability, poor biocompatibility, high complication rates and low quality of life for the recipients.
-
We are on the verge of an era in total artificial heart development in which rapidly evolving technologies from different fields will lead to new approaches in total artificial heart design and development.
-
More powerful and more compact batteries and transcutaneous energy transfer systems will omit the need for percutaneous cables and will improve the quality of life of the recipients of a total artificial heart.
-
With the rise of soft robotic technologies and smart biomaterials, completely soft total artificial hearts might soon be developed and are likely to have fewer biocompatibility issues than current devices.
This is a preview of subscription content, access via your institution
Access options
Access Nature and 54 other Nature Portfolio journals
Get Nature+, our best-value online-access subscription
$29.99 / 30 days
cancel any time
Subscribe to this journal
Receive 12 print issues and online access
$209.00 per year
only $17.42 per issue
Buy this article
- Purchase on Springer Link
- Instant access to full article PDF
Prices may be subject to local taxes which are calculated during checkout
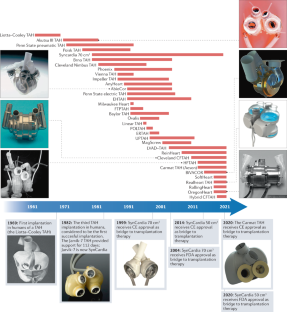


Similar content being viewed by others
References
Metra, M. & Teerlink, J. R. Heart failure. Lancet 390, 1981–1995 (2017).
Heidenreich, P. A. et al. Forecasting the impact of heart failure in the United States: a policy statement from the American Heart Association. Circ. Heart Fail. 6, 606–619 (2013).
Colvin, M. et al. OPTN/SRTR 2018 annual data report: heart. Am. J. Transpl. 20, 340–426 (2020).
Molina, E. J. et al. The Society of Thoracic Surgeons Intermacs 2020 annual report. Ann. Thorac. Surg. 111, 778–792 (2021).
Han, J. J., Acker, M. A. & Atluri, P. Left ventricular assist devices. Circulation 138, 2841–2851 (2018).
Gurvits, G. E. & Fradkov, E. Bleeding with the artificial heart: gastrointestinal hemorrhage in CF-LVAD patients. World J. Gastroenterol. 23, 3945–3953 (2017).
Galand, V. et al. Predictors and clinical impact of late ventricular arrhythmias in patients with continuous-flow left ventricular assist devices. JACC Clin. Electrophysiol. 4, 1166–1175 (2018).
Ross, D. W. et al. Left ventricular assist devices and the kidney. Clin. J. Am. Soc. Nephrol. 13, 348–355 (2018).
Aissaoui, N. et al. Understanding left ventricular assist devices. Blood Purif. 46, 292–300 (2018).
National Heart Lung and Blood Institute. What Is Total Artificial Heart? Total Artificial Heart https://www.nhlbi.nih.gov/health-topics/total-artificial-heart (NIH, 2020).
Cooley, D. A. et al. Orthotopic cardiac prosthesis for two-staged cardiac replacement. Am. J. Cardiol. 24, 723–730 (1969).
US National Library of Medicine. SynCardia 70cc TAH-t for Destination Therapy (DT) (RA-540). ClinicalTrials.gov https://ClinicalTrials.gov/show/NCT02232659 (2021).
Akutsu, T. & Kolff, W. J. Permanent substitutes for valves and hearts. ASAIO J. 4, 230–234 (1958).
Houston, C. S., Akutsu, T. & Kolff, W. J. Pendulum type of artificial heart within the chest: preliminiary report. Am. Heart J. 59, 723–730 (1960).
Seidel, W., Akutsu, T., Mirkovitch, V., Brown, F. & Kolff, W. J. Air-driven artificial hearts inside the chest. Trans. Am. Soc. Artif. Intern. Organs 7, 378–387 (1961).
Liotta, D. et al. Artificial heart in the chest: preliminary report. Trans. Am. Soc. Artif. Intern. Organs 7, 318–322 (1961).
Atsumi, K. et al. Artificial heart incorporated in the chest. Trans. Am. Soc. Artif. Intern. Organs 9, 292–298 (1963).
Pierce, W. S. et al. Total heart replacement by a single intrathoracic blood pump. J. Surg. Res. 5, 387–394 (1965).
Nosé, Y., Tretbar, L. L., SenGupta, A., Topaz, S. R. & Kolff, W. J. An artificial heart inside the chest. J. Thorac. Cardiovasc. Surg. 50, 792–799 (1965).
Cohn, W. E., Timms, D. L. & Frazier, O. H. Total artificial hearts: past, present, and future. Nat. Rev. Cardiol. 12, 609–617 (2015).
Curran, W. J. Law-medicine notes. The first mechanical heart transplant: informed consent and experimentation. N. Engl. J. Med. 291, 1015–1016 (1974).
Morris, D. T. & Couves, C. M. Experiences with a sac-type artificial heart. Can. Med. Assoc. J. 105, 483–487 (1971).
Kwan-Gett, C. S., Van Kampen, K. R., Kawai, J., Eastwood, N. & Kolff, W. J. Results of total artificial heart implantation in calves. J. Thorac. Cardiovasc. Surg. 62, 880–889 (1971).
Kawai, J. et al. Implantation of a total artificial heart in calves under hypothermia with 10 day survival. J. Thorac. Cardiovasc. Surg. 64, 45–60 (1972).
Hastings, W. L. et al. A retrospective study of nine calves surviving five months on the pneumatic total artificial heart. Trans. Am. Soc. Artif. Intern. Organs 27, 71–76 (1981).
Fukumasu, H., Iwaya, F., Olsen, D. B., Lawson, J. H. & Kolff, W. J. Surgical implantation of the Jarvik-5 total artificial heart in a calf. Trans. Am. Soc. Artif. Intern. Organs 25, 232–238 (1979).
Akutsu, T., Takagi, H. & Takano, H. Total artificial hearts with built-in valves. Trans. Am. Soc. Artif. Intern. Organs 16, 392–397 (1970).
Honda, T. et al. One 25 day survivor with total artificial heart. J. Thorac. Cardiovasc. Surg. 69, 92–101 (1975).
Nakazono, M. et al. A case report of 17 days survival with an implanted artificial heart in a calf. Jpn Heart J. 15, 485–497 (1974).
Kasai, S. et al. Survival for 145 days with a total artificial heart. J. Thorac. Cardiovasc. Surg. 73, 637–646 (1977).
Kennedy, J. H. et al. Development of an orthotopic cardiac prosthesis. J. Thorac. Cardiovasc. Surg. 65, 673–683 (1973).
Backman, D. K., Donovan, F. M., Sandquist, G., Kessler, T. & Kolff, W. J. The design and evaluation of ventricles for the aec artificial heart nuclear power source. ASAIO J. 19, 542–552 (1973).
Smith, L. et al. Development on the implantation of a total nuclear-powered artificial heart system. ASAIO J. 20, 732–735 (1974).
Urzua, J., Sudilovsky, O., Panke, T., Kiraly, R. J. & Nosé, Y. Preliminary report: anatomic constraints for the implantation of an artificial heart. J. Surg. Res. 17, 262–268 (1974).
Cooley, D. A., Akutsu, T., Norman, J. C., Serrato, M. A. & Frazier, O. H. Total artificial heart in two-staged cardiac transplantation. Cardiovasc. Dis. 8, 305–319 (1981).
DeVries, W. C. et al. Clinical use of the total artificial heart. N. Engl. J. Med. 310, 273–278 (1984).
Vasků, J. & Urbánek, P. Constructional and functional characteristics of recent total artificial heart models TNS Brno VII, VIII, and IX. Artif. Organs 19, 535–543 (1995).
Davis, P. K., Pae, W. E. Jr & Pierce, W. S. Toward an implantable artificial heart. Experimental and clinical experience at The Pennsylvania State University. Invest. Radiol. 24, 81–87 (1989).
Hsu, C. H. Fuzzy logic automatic control of the Phoenix-7 total artificial heart. J. Artif. Organs 7, 69–76 (2004).
Hsu, C. H. In vivo and clinical study of Phoenix-7 total artificial heart. Biomed. Eng. Appl. Basis Commun. 13, 133–139 (2001).
Shumakov, V. I. et al. Use of an ellipsoid artificial heart. Artif. Organs 11, 16–19 (1987).
Shumakov, V. I. et al. New design of an orthotopic fluorosiloxane rubber heart prosthesis. Biomed. Eng. 10, 223–224 (1976).
Nawrat, Z. & Malota, Z. The analysis of driving mode influence on energy dissipation in pneumatic artificial heart chambers. Artif. Organs 22, 898–904 (1998).
Rokitansky, A. et al. The new small Viennese total artificial heart: experimental and first clinical experiences. Artif. Organs 15, 129–135 (1991).
Homma, A. et al. Development of an electrohydraulic total artificial heart system: improvement of pump unit. Electron. Commun. Jpn. 93, 34–46 (2010).
Ford, B. J. A new generation of cardiology: the AbioCor implantable replacement heart. Air Med. J. 22, 26–30 (2003).
Dowling, R. D. et al. The AbioCor implantable replacement heart. Ann. Thorac. Surg. 75, S93–S99 (2003).
Smith, P. A., Cohn, W. E. & Frazier, O. H. in Mechanical Circulatory and Respiratory Support (eds Gregory, S. D., Stevens, M. C. & Fraser, J. F.) Ch. 7, 221–244 (Academic, 2018).
Spiliopoulos, S., Dimitriou, A. M., Guersoy, D., Koerfer, R. & Tenderich, G. Expanding applicability of total artificial heart therapy: the 50-cc SynCardia total artificial heart. Ann. Thorac. Surg. 100, e55–e57 (2015).
Cohrs, N. H. et al. A soft total artificial heart-first concept evaluation on a hybrid mock circulation. Artif. Organs 41, 948–958 (2017).
Stepanenko, A. & Kaufmann, F. A novel total artificial heart: search for haemocompatibility. Lancet 386, 1517–1519 (2015).
CARMAT. Artificial hearts: devices from France’s Carmat to go on sale in Europe. YouTube https://www.youtube.com/watch?v=j9T2HnSfVME (2021).
US Food and Drug Administration. SynCardia Temporary Cardio West Total Artificial Heart (TAH-T). AccessData https://www.accessdata.fda.gov/scripts/cdrh/cfdocs/cfpma/pma.cfm?ID=P030011 (2004).
EC Design-Examination Certificate. SynCardia temporary Total Artificial Heart (TAH-t) and external drivers. SynCardia http://syncardia.com/wp-content/uploads/2018/02/CE-665479-Design-Examination-26-May-2017.pdf (2017).
SynCardia. SynCardia 70cc total artificial heart. SynCardia https://syncardia.com/clinicians/our-products/see-all-our-products/ (2021).
US National Library of Medicine. Carmat TAH early feasibility study. ClinicalTrials.gov https://clinicaltrials.gov/ct2/show/NCT04117295 (2022).
Guex, L. G. et al. Increased longevity and pumping performance of an injection molded soft total artificial heart. Soft Robot. 23, 23 (2020).
Mihaylov, D., Verkerke, G. J. & Rakhorst, G. Mechanical circulatory support systems — a review. Technol. Health Care 8, 251–266 (2000).
Min, B. G. et al. A moving-actuator type electromechanical total artificial heart — Part II: circular type and animal experiment. IEEE Trans. Biomed. Eng. 37, 1195–1200 (1990).
Min, B. G. et al. A moving-actuator type electromechanical total artificial heart — Part I: linear type and mock circulation experiments. IEEE Trans. Biomed. Eng. 37, 1186–1194 (1990).
Ahn, J. M., Kang, D. W., Kim, H. C. & Min, B. G. In vivo performance evaluation of a transcutaneous energy and information transmission system for the total artificial heart. ASAIO J. 39, M208–M212 (1993).
Ohashi, Y., de Andrade, A. & Nosé, Y. Hemolysis in an electromechanical driven pulsatile total artificial heart. Artif. Organs 27, 1089–1093 (2003).
Takatani, S. et al. One piece ultracompact totally implantable electromechanical total artificial heart for permanent use. ASAIO J. 48, 538–545 (2002).
Irié, H. et al. Initial in vivo tests of an electrohydraulic actuated total artificial heart. ASAIO J. 38, M497–M500 (1992).
Fukamachi, K. et al. Anatomic fitting studies of a total artificial heart in heart transplant recipients. Critical dimensions and prediction of fit. ASAIO J. 42, M337–M342 (1996).
Sueshiro, M., Fukunaga, S., Hirai, S., Sueda, T. & Matsuura, Y. Eccentric roller type total artificial heart designed for implantation. Artif. Organs 22, 451–457 (1998).
Kobayashi, M. et al. In vitro evaluation of linear motor-driven total artificial heart. Artif. Organs 20, 1320–1324 (1996).
Yamada, H., Yamaguchi, M., Kobayashi, K., Matsuura, Y. & Takano, H. Development and test of a linear motor-driven total artificial heart. IEEE Eng. Med. Biol. Mag. 14, 84–90 (1995).
Weber, S. et al. MagScrew TAH: an update. ASAIO J. 51, xxxvi–xlvi (2005).
Gao, H. et al. In vitro assessment of the Milwaukee heart and right to left balance. ASAIO J. 38, M722–M725 (1992).
Sauer, I. M., Frank, J., Spiegelberg, A. & Bücherl, E. S. Ovalis TAH: development and in vitro testing of a new electromechanical energy converter for a total artificial heart. ASAIO J. 46, 744–748 (2000).
Mehta, S. M. et al. Testing of a 50 cc stroke volume completely implantable artificial heart: expanding chronic mechanical circulatory support to women, adolescents, and small stature men. ASAIO J. 46, 779–782 (2000).
Szabó, Z. et al. Scandinavian real heart (SRH) 11 implantation as total artificial heart (TAH)-experimental update. J. Clin. Exp. Cardiol. 9, 2 (2018).
Sonntag, S. J. et al. Virtual implantations to transition from porcine to bovine animal models for a total artificial heart. Artif. Organs 44, 384–393 (2020).
Pelletier, B. et al. System overview of the fully implantable destination therapy — ReinHeart total artificial heart. Eur. J. Cardiothorac. Surg. 47, 80–86 (2015).
Tozzi, P. et al. An original valveless artificial heart providing pulsatile flow tested in mock circulatory loops. Int. J. Artif. Organs 40, 683–689 (2017).
Kirklin, J. K. et al. Eighth annual INTERMACS report: special focus on framing the impact of adverse events. J. Heart Lung Transpl. 36, 1080–1086 (2017).
Potapov, E. V., Kaufmann, F., Müller, M., Mulzer, J. & Falk, V. Longest ongoing support (13 years) with magnetically levitated left ventricular assist device. ASAIO J. 66, e121–e122 (2020).
Yang, M. in Technology and Therapy Management 99–100 (Springer, 2020).
Healy, A. H. et al. Physiologic effects of continuous-flow left ventricular assist devices. J. Surg. Res. 202, 363–371 (2016).
Bhimaraj, A., Uribe, C. & Suarez, E. E. Physiological impact of continuous flow on end-organ function: clinical implications in the current era of left ventricular assist devices. Methodist. Debakey Cardiovasc. J. 11, 12–17 (2015).
Isoyama, T. et al. New version of flow-transformed pulsatile total artificial heart with no electrical switching valve. Artif. Organs 19, 694–696 (1995).
Abe, Y. et al. Results of animal experiments with the fourth model of the undulation pump total artificial heart. Artif. Organs 35, 781–790 (2011).
Qian, K. X., Ru, W. M., Zeng, P. & Yuan, H. Y. A novel impeller TAH using magnetic bearings for load reduction. J. Med. Eng. Technol. 26, 214–216 (2002).
Feng, J. et al. New continuous-flow total artificial heart and vascular permeability. J. Surg. Res. 199, 296–305 (2015).
Lebreton, G., Mastroianni, C., Amour, J. & Leprince, P. Implantation of two HVADs used as a total artificial heart: a new approach. Ann. Thorac. Surg. 107, e165–e167 (2019).
Mulvihill, M. S. et al. Usefulness of two centrifugal ventricular assist devices in a total artificial heart configuration: a preliminary report. J. Heart Lung Transpl. 36, 1266–1268 (2017).
Cohn, W. E. et al. Eight-year experience with a continuous-flow total artificial heart in calves. ASAIO J. 60, 25–30 (2014).
Pirk, J. et al. Total artificial heart support with two continuous-flow ventricular assist devices in a patient with an infiltrating cardiac sarcoma. ASAIO J. 59, 178–180 (2013).
Frazier, O. H. & Cohn, W. E. Continuous-flow total heart replacement device implanted in a 55-year-old man with end-stage heart failure and severe amyloidosis. Tex. Heart Inst. J. 39, 542–546 (2012).
Strueber, M., Schmitto, J. D., Kutschka, I. & Haverich, A. Placement of two implantable centrifugal pumps to serve as a total artificial heart after cardiectomy. J. Thorac. Cardiovasc. Surg. 143, 507–509 (2012).
Frazier, O. H., Cohn, W. E., Tuzun, E., Winkler, J. A. & Gregoric, I. D. Continuous-flow total artificial heart supports long-term survival of a calf. Tex. Heart Inst. J. 36, 568–574 (2009).
Baldwin, A. C., Gemmato, C. J., Cohn, W. E. & Frazier, O. H. Feasibility of long-term continuous flow total heart replacement in calves. Int. J. Artif. Organs 45, 44–51 (2021).
Daneshmand, M. A., Bishawi, M., Milano, C. A. & Schroder, J. N. The HeartMate 6. ASAIO J. 66, e46–e49 (2020).
Kleinheyer, M. et al. Rapid speed modulation of a rotary total artificial heart impeller. Artif. Organs 40, 824–833 (2016).
Fukamachi, K. et al. Generating pulsatility by pump speed modulation with continuous-flow total artificial heart in awake calves. J. Artif. Organs 20, 381–385 (2017).
Miyamoto, T. et al. Analysis of Cleveland clinic continuous-flow total artificial heart performance using the virtual mock loop: comparison with an in vivo study. Artif. Organs 44, 375–383 (2020).
Abe, Y. et al. Animal experiments of the helical flow total artificial heart. Artif. Organs 39, 670–680 (2015).
Fox, C. et al. Hybrid continuous-flow total artificial heart. Artif. Organs 42, 500–509 (2018).
Glynn, J. et al. The OregonHeart total artificial heart: design and performance on a mock circulatory loop. Artif. Organs 41, 904–910 (2017).
Jurney, P. L. et al. Characterization of a pulsatile rotary total artificial heart. Artif. Organs 45, 135–142 (2021).
Franklin, D. L., Van Citters, R. L. & Rushmer, R. F. Balance between right and left ventricular output. Circ. Res. 10, 17–26 (1962).
Ley, S., Kreitner, K. F., Morgenstern, I., Thelen, M. & Kauczor, H. U. Bronchopulmonary shunts in patients with chronic thromboembolic pulmonary hypertension: evaluation with helical CT and MR imaging. Am. J. Roentgenol. 179, 1209–1215 (2002).
Baile, E. M., Ling, H., Heyworth, J. R., Hogg, J. C. & Pare, P. D. Bronchopulmonary anastomotic and noncoronary collateral blood flow in humans during cardiopulmonary bypass. Chest 87, 749–754 (1985).
Bhunia, S. K. & Kung, R. T. Indirect bronchial shunt flow measurements in AbioCor implantable replacement heart recipients. ASAIO J. 50, 211–214 (2004).
Nestler, F. et al. Investigation of the inherent left-right flow balancing of rotary total artificial hearts by means of a resistance box. Artif. Organs 44, 584–593 (2020).
Latrémouille, C. et al. A bioprosthetic total artificial heart for end-stage heart failure: results from a pilot study. J. Heart Lung Transpl. 37, 33–37 (2018).
Slepian, M. J. et al. The Syncardia™ total artificial heart: in vivo, in vitro, and computational modeling studies. J. Biomech. 46, 266–275 (2013).
Crosby, J. R. et al. Physiological characterization of the SynCardia total artificial heart in a mock circulation system. ASAIO J. 61, 274–281 (2015).
Diedrich, M. et al. Experimental investigation of right–left flow balance concepts for a total artificial heart. Artif. Organs 45, 364–372 (2021).
Horvath, D. et al. Mechanism of self-regulation and in vivo performance of the cleveland clinic continuous-flow total artificial heart. Artif. Organs 41, 411–417 (2017).
Kung, R. T. et al. Progress in the development of the ABIOMED total artificial heart. ASAIO J. 41, M245–M248 (1995).
Harasaki, H. et al. Progress in Cleveland Clinic — Nimbus total artificial heart development. ASAIO J. 40, M494–M498 (1994).
Kim, H. C., Khanwilkar, P. S., Bearnson, G. B. & Olsen, D. B. Development of a microcontroller-based automatic control system for the electrohydraulic total artificial heart. IEEE Trans. Biomed. Eng. 44, 77–89 (1997).
Snyder, A. J. et al. An electrically powered total artificial heart. Over 1 year survival in the calf. ASAIO J. 38, M707–M712 (1992).
Abe, Y. et al. Third model of the undulation pump total artificial heart. ASAIO J. 49, 123–127 (2003).
Dowling, R. D. et al. Initial experience with the AbioCor implantable replacement heart at the University of Louisville. ASAIO J. 46, 579–581 (2000).
Latrémouille, C. et al. Animal studies with the Carmat bioprosthetic total artificial heart. Eur. J. Cardiothorac. Surg. 47, e172–e179 (2015).
Vasků, J. et al. A comparative study of a group of eight calves, surviving longer than 1 month with the total artificial heart. Artif. Organs 7, 470–478 (1983).
Vasků, J., Urbánek, P., Dostál, M. & Vasků, J. The applicability of experimental experience with the total artificial heart to its clinical use. Int. J. Artif. Organs 15, 307–311 (1992).
Copeland, J. G. et al. The total artificial heart as a bridge to transplantation. A report of two cases. JAMA 256, 2991–2995 (1986).
Shumakov, V. et al. Clinical indications for the use of the “Poisk-IOM” total artificial heart: the experience of 13 implantations in humans. Artif. Organs 15, 372–375 (1991).
Wei, J. et al. Successful use of Phoenix-7 total artificial heart. Transpl. Proc. 30, 3403–3404 (1998).
Trubel, W. et al. Clinical total artificial heart bridging: Viennese strategy and experiences. Artif. Organs 13, 470–475 (1989).
Frazier, O. H. et al. The total artificial heart: where we stand. Cardiology 101, 117–121 (2004).
Alaeddine, M., Ploutz, M., Arabia, F. A. & Velez, D. A. Implantation of total artificial heart in a 10-year-old after support with a temporary perventricular assist device. J. Thorac. Cardiovasc. Surg. 159, e227–e229 (2020).
SynCardia. Turkish man becomes world’s longest supported syncardia temporary total artificial heart patient. SynCardia https://syncardia.com/news/turkish-man-becomes-worlds-longest-supported-syncardia-temporary-total-artificial-heart-patient/ (2017).
David, C. H. et al. A heart transplant after total artificial heart support: initial and long-term results. Eur. J. Cardiothorac. Surg. 58, 1175–1181 (2020).
Carrier, M. et al. Outcomes after heart transplantation and total artificial heart implantation: a multicenter study. J. Heart Lung Transplant. 28, 28 (2020).
Hulman, M., Artemiou, P., Hudec, V., Olejarova, I. & Goncalvesova, E. SynCardia, total artificial heart, as a bridge to transplant. Bratisl. Lek. Listy 120, 325–330 (2019).
Nguyen, A. et al. Experience with the SynCardia total artificial heart in a Canadian centre. Can. J. Surg. 60, 375–379 (2017).
Kirsch, M. E. et al. SynCardia temporary total artificial heart as bridge to transplantation: current results at La Pitié hospital. Ann. Thorac. Surg. 95, 1640–1646 (2013).
Copeland, J. G. et al. Experience with more than 100 total artificial heart implants. J. Thorac. Cardiovasc. Surg. 143, 727–734 (2012).
Roussel, J. C. et al. CardioWest (Jarvik) total artificial heart: a single-center experience with 42 patients. Ann. Thorac. Surg. 87, 124–130 (2009).
El-Banayosy, A. et al. CardioWest total artificial heart: bad Oeynhausen experience. Ann. Thorac. Surg. 80, 548–552 (2005).
Thanavaro, K. L., Tang, D. G., Kasirajan, V. & Shah, K. B. Clinical indications for implantation of the total artificial heart. ASAIO J. 60, 594–596 (2014).
Carmat. Carmat outlines commercial and development plan for its total artificial heart. Carmat https://www.carmatsa.com/carmat-content/uploads/2021/01/pr_carmat_conference_06-01-21.pdf (2021).
Netuka, I. et al. Initial bridge to transplant experience with a bioprosthetic autoregulated artificial heart. J. Heart Lung Transpl. 39, 1491–1493 (2020).
Unthan, K. et al. Design and evaluation of a fully implantable control unit for blood pumps. Biomed. Res. Int. 2015, 257848 (2015).
Kluin, J. et al. In situ heart valve tissue engineering using a bioresorbable elastomeric implant — from material design to 12 months follow-up in sheep. Biomaterials 125, 101–117 (2017).
Zilla, P., Deutsch, M., Bezuidenhout, D., Davies, N. H. & Pennel, T. Progressive reinvention or destination lost? Half a century of cardiovascular tissue engineering. Front. Cardiovasc. Med. 7, 159 (2020).
Chen, Z. et al. Device-induced platelet dysfunction in mechanically assisted circulation increases the risks of thrombosis and bleeding. Artif. Organs 43, 745–755 (2019).
Zaiser, A. S. et al. Adverse events of percutaneous microaxial left ventricular assist devices-a retrospective, single-centre cohort study. J. Clin. Med. 10, 3710 (2021).
HybridHeart. The development of a soft biocompatible artificial heart. HybridHeart https://www.hybridheart.eu/ (2021).
Kohll, A. X. et al. Long-term performance of a pneumatically actuated soft pump manufactured by rubber compression molding. Soft Robot. 6, 206–213 (2019).
Roche, E. T. et al. Soft robotic sleeve supports heart function. Sci. Transl. Med. 9, eaaf3925 (2017).
Banerjee, H., Tse, Z. T. H. & Ren, H. Soft robotics with compliance and adaptation for biomedical applications and forthcoming challenges. Int. J. Robot. Autom. 33, 69–80 (2018).
Greatrex, N., Kleinheyer, M., Nestler, F. & Timms, D. The Maglev heart. IEEE Spectr. 56, 22–29 (2019).
Parnis, S. M. et al. Chronic in vivo evaluation of an electrohydraulic total artificial heart. ASAIO J. 40, M489–M493 (1994).
Dowling, R. D., Etoch, S. W., Stevens, K. A., Johnson, A. C. & Gray, L. A. Jr Current status of the AbioCor implantable replacement heart. Ann. Thorac. Surg. 71, S147–S149 (2001).
Takatani, S. et al. Totally implantable total artificial heart and ventricular assist device with multipurpose miniature electromechanical energy system. Artif. Organs 18, 80–92 (1994).
Takatani, S. et al. Left and right pump output control in one-piece electromechanical total artificial heart. Artif. Organs 17, 176–184 (1993).
Cohn, W. E. et al. Pulsatile outflow in cows supported long-term with the BiVACOR rotary TAH. J. Heart Lung Transplant. 36, S14 (2017).
Dostál, M. et al. Hematological and biochemical studies in calves living over 100 days with the polymethylmethacrylate total artificial heart TNS Brno II. Int. J. Artif. Organs 9, 39–48 (1986).
Vasků, J. et al. Recent efforts in artificial heart research in Czechoslovakia. ASAIO Trans. 35, 805–811 (1989).
Smadja, D. M. et al. The Carmat bioprosthetic total artificial heart is associated with early hemostatic recovery and no acquired von Willebrand syndrome in calves. J. Cardiothorac. Vasc. Anesth. 31, 1595–1602 (2017).
Karimov, J. H. et al. First report of 90-day support of two calves with a continuous-flow total artificial heart. J. Thorac. Cardiovasc. Surg. 150, 687–693.e681 (2015).
Taenaka, Y. et al. Development and evaluation of components for a totally implantable artificial heart system. ASAIO J. 40, M314–M318 (1994).
Taenaka, Y. et al. An electrohydraulic total artificial heart with a separately placed actuator. ASAIO Trans. 36, M242–M245 (1990).
Tatsumi, E. et al. A blood pump with an interatrial shunt for use as an electrohydraulic total artificial heart. ASAIO J. 38, M425–M430 (1992).
Tatsumi, E. et al. The National Cardiovascular Center electrohydraulic total artificial heart and ventricular assist device systems: current status of development. ASAIO J. 49, 243–249 (2003).
Tatsumi, E. et al. Current status of development and in vivo evaluation of the National Cardiovascular Center electrohydraulic total artificial heart system. J. Artif. Organs 3, 62–69 (2000).
Doi, K. et al. In vivo studies of the MagScrew total artificial heart in calves. ASAIO J. 48, 222–225 (2002).
Kuroda, H. et al. Postoperative pulmonary complications in calves after implantation of an electric total artificial heart. ASAIO J. 44, M613–M618 (1998).
Pierce, W. S. et al. An electric artificial heart for clinical use. Ann. Surg. 212, 339–343 (1990).
Snyder, A. J. et al. In vivo testing of a completely implanted total artificial heart system. ASAIO J. 39, M177–M184 (1993).
Shaffer, L. J. et al. Total artificial heart implantation in calves with pump on an angled port design. Trans. Am. Soc. Artif. Intern. Organs 25, 254–259 (1979).
Abe, Y. et al. Development of mechanical circulatory support devices at the University of Tokyo. J. Artif. Organs 10, 60–70 (2007).
Mochizuki, S. et al. Results of animal experiments using an undulation pump total artificial heart: analysis of 10 day and 19 day survival. ASAIO J. 46, 500–504 (2000).
Wampler, R. et al. Performance of a novel shuttling total artificial heart on a on a mock circulatory loop. J. Heart Lung Transplant. 36, S56–S57 (2017).
Cooley, D. The total artificial heart. Nat. Med. 9, 108–111 (2003).
Emmanuel, S. et al. Anatomical human fitting of the BiVACOR total artificial heart. Artif. Organs 46, 50–56 (2022).
Mohacsi, P. & Leprince, P. The Carmat total artificial heart. Eur. J. Cardiothorac. Surg. 46, 933–934 (2014).
Pieper, I. L. et al. Evaluation of the novel total artificial heart Realheart in a pilot human fitting study. Artif. Organs 44, 174–177 (2020).
Yu, L. S. et al. A compact and noise free electrohydraulic total artificial heart. ASAIO J. 39, M386–M391 (1993).
Rosenberg, G. et al. Dynamic in vitro and in vivo performance of a permanent total artificial heart. Artif. Organs 22, 87–94 (1998).
Marieb, E. N. & Hoehn, K. in The Heart 679–681 (Pearson, 2013).
Acknowledgements
The authors are grateful to L. C. van Laake (AMOLF, Netherlands), D. Zrinscak (Scuola Superiore Sant’Anna, Italy) and A. Henseler (evos GmbH, Germany) for their contributions and discussions; D. van Urk (Amsterdam UMC, The Netherlands) for assisting with screening and selecting articles; T. Azami (Amsterdam UMC, The Netherlands) and C. M. van de Beek (Amsterdam UMC, The Netherlands) for assistance with data extraction; C. E. J. M. Limpens (Amsterdam UMC, The Netherlands) and A. Malekzadeh (Amsterdam UMC, The Netherlands) for their help with the literature search; and ReinHeart TAH GmbH, Germany for providing additional data upon request. The authors’ work is part of the HybridHeart project and is funded by the European Union Horizon 2020 research and innovation programme under grant agreement no. 767195.
Author information
Authors and Affiliations
Contributions
A.V. and M.A. did the major literature search and wrote the first draft. All of the authors contributed to the discussion of content and reviewed and edited the manuscript before submission.
Corresponding author
Ethics declarations
Competing interests
A.V., M.A., H.K., J.T.B.O. and J.K. are part of the HybridHeart consortium. The other authors declare no competing interests.
Peer review
Peer review information
Nature Reviews Cardiology thanks the anonymous reviewer(s) for their contribution to the peer review of this work.
Additional information
Publisher’s note
Springer Nature remains neutral with regard to jurisdictional claims in published maps and institutional affiliations.
Glossary
- Driveline
-
Percutaneous cable that transmits electrical power from an external driver to the internally implanted device such as a TAH or LVAD.
- Transcutaneous energy transfer (TET) system
-
A wireless power delivery system that uses magnetic fields to transfer power across the skin without the need for direct electrical connectivity.
- Bronchial shunt
-
The physiological passage of oxygenated blood from the aorta to the bronchial circulation. This blood returns directly to the left atrium, thereby bypassing the right side of the heart.
- Frank–Starling mechanism
-
This law states that the stroke volume of the heart increases in response to an increase in the volume of blood in the ventricles before contraction (the end diastolic volume), when all other factors remain constant.
- Preload
-
The filling pressure of the ventricle at the end of diastole, which is determined by the atrial pressure.
- Stator
-
The stationary part of a rotary machine or device.
- Investigational device exemption
-
Type of FDA approval that allows the investigational device to be used in a clinical study in order to collect safety and efficacy data.
- Afterload
-
The amount of pressure that the ventricle needs to exert to eject the blood during ventricular contraction.
- Biofunctionalization
-
The modification of a material to add a biological function, such as replace or repair, while at the same time being accepted by the host organism.
Rights and permissions
About this article
Cite this article
Vis, A., Arfaee, M., Khambati, H. et al. The ongoing quest for the first total artificial heart as destination therapy. Nat Rev Cardiol 19, 813–828 (2022). https://doi.org/10.1038/s41569-022-00723-8
Accepted:
Published:
Issue Date:
DOI: https://doi.org/10.1038/s41569-022-00723-8
This article is cited by
-
Stem Cell Therapy, Artificial Heart or Xenotransplantation: What will be New “Regenerative” Strategies in Heart Failure during the Next Decade?
Stem Cell Reviews and Reports (2023)