Abstract
The development of a Pd(ii)-catalysed enantioselective fluorination of C(sp3)−H bonds would offer a new approach to making chiral organofluorines. However, such a strategy is particularly challenging because of the difficulty in differentiating prochiral C(sp3)−H bonds through Pd(ii)-insertion, as well as the sluggish reductive elimination involving Pd−F bonds. Here, we report the development of a Pd(ii)-catalysed enantioselective C(sp3)−H fluorination using a chiral transient directing group strategy. In this work, a bulky, amino amide transient directing group was developed to control the stereochemistry of the C−H insertion step and selectively promote the C(sp3)−F reductive elimination pathway from the Pd(iv)–F intermediate. Stereochemical analysis revealed that while the desired C(sp3)−F formation proceeds via an inner-sphere pathway with retention of configuration, the undesired C(sp3)−O formation occurs through an SN2-type mechanism. Elucidation of the dual mechanism allows us to rationalize the profound ligand effect on controlling reductive elimination selectivity from high-valent Pd species.
This is a preview of subscription content, access via your institution
Access options
Access Nature and 54 other Nature Portfolio journals
Get Nature+, our best-value online-access subscription
$29.99 / 30 days
cancel any time
Subscribe to this journal
Receive 12 print issues and online access
$259.00 per year
only $21.58 per issue
Buy this article
- Purchase on Springer Link
- Instant access to full article PDF
Prices may be subject to local taxes which are calculated during checkout




Similar content being viewed by others
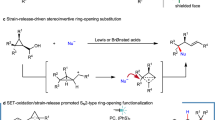
References
O’Hagan, D. Understanding organofluorine chemistry. An introduction to the C–F bond. Chem. Soc. Rev. 37, 308–319 (2008).
Purser, S., Moore, P. R., Swallow, S. & Gouverneur, V. Fluorine in medicinal chemistry. Chem. Soc. Rev. 37, 320–330 (2008).
Fujiwara, T. & O’Hagan, D. Successful fluorine-containing herbicide agrochemicals. J. Fluor. Chem. 167, 16–29 (2014).
Wang, J. et al. Fluorine in pharmaceutical industry: fluorine-containing drugs introduced to the market in the last decade (2001–2011). Chem. Rev. 114, 2432–2506 (2014).
Furuya, T., Kamlet, A. S. & Ritter, T. Catalysis for fluorination and trifluoromethylation. Nature 473, 470–477 (2011).
Hollingworth, C. & Gouverneur, V. Transition metal catalysis and nucleophilic fluorination. Chem. Commun. 48, 2929–2942 (2012).
Sather, A. C. & Buchwald, S. L. The evolution of Pd0/PdII-catalyzed aromatic fluorination. Acc. Chem. Res. 49, 2146–2157 (2016).
Yang, X., Wu, T., Phipps, R. J. & Toste, F. D. Advances in catalytic enantioselective fluorination, mono-, di-, and trifluoromethylation, and trifluoromethylthiolation reactions. Chem. Rev. 115, 826–870 (2015).
Hintermann, L. & Togni, A. Catalytic enantioselective fluorination of β-ketoesters. Angew. Chem. Int. Ed. 39, 4359–4362 (2000).
Marigo, M., Fielenbach, D., Braunton, A., Kjærsgaard, A. & Jørgensen, K. A. Enantioselective formation of stereogenic carbon–fluorine centers by a simple catalytic method. Angew. Chem. Int. Ed. 44, 3703–3706 (2005).
Beeson, T. D. & MacMillan, D. W. C. Enantioselective organocatalytic α-fluorination of aldehydes. J. Am. Chem. Soc. 127, 8826–8828 (2005).
Rauniyar, V., Lackner, A. D., Hamilton, G. L. & Toste, F. D. Asymmetric electrophilic fluorination using an anionic chiral phase-transfer catalyst. Science 334, 1681–1684 (2011).
Talbot, E. P. A., Fernandes, T. d. A., McKenna, J. M. & Toste, F. D. Asymmetric palladium-catalyzed directed intermolecular fluoroarylation of styrenes. J. Am. Chem. Soc. 136, 4101–4104 (2014).
Cochrane, N. A., Nguyen, H. & Gagné, M. R. Catalytic enantioselective cyclization and C3-fluorination of polyenes. J. Am. Chem. Soc. 135, 628–631 (2013).
Kalow, J. A. & Doyle, A. G. Enantioselective ring opening of epoxides by fluoride anion promoted by a cooperative dual-catalyst system. J. Am. Chem. Soc. 132, 3268–3269 (2010).
Katcher, M. H. & Doyle, A. G. Palladium-catalyzed asymmetric synthesis of allylic fluorides. J. Am. Chem. Soc. 132, 17402–17404 (2010).
Zhang, Q., Stockdale, D. P., Mixdorf, J. C., Topczewski, J. J. & Nguyen, H. M. Iridium-catalyzed enantioselective fluorination of racemic, secondary allylic trichloroacetimidates. J. Am. Chem. Soc. 137, 11912–11915 (2015).
Hull, K. L., Anani, W. Q. & Sanford, M. S. Palladium-catalyzed fluorination of carbon−hydrogen bonds. J. Am. Chem. Soc. 128, 7134–7135 (2006).
Braun, M.-G. & Doyle, A. G. Palladium-catalyzed allylic C–H fluorination. J. Am. Chem. Soc. 135, 12990–12993 (2013).
Zhang, Q., Yin, X.-S., Chen, K., Zhang, S.-Q. & Shi, B.-F. Stereoselective synthesis of chiral β-fluoro α-amino acids via Pd(ii)-catalyzed fluorination of unactivated methylene C(sp 3)–H bonds: scope and mechanistic studies. J. Am. Chem. Soc. 137, 8219–8226 (2015).
Zhu, R.-Y. et al. Ligand-enabled stereoselective β-C(sp 3)–H fluorination: synthesis of unnatural enantiopure anti-β-fluoro-α-amino acids. J. Am. Chem. Soc. 137, 7067–7070 (2015).
Rueda-Becerril, M. et al. Fluorine transfer to alkyl radicals. J. Am. Chem. Soc. 134, 4026–4029 (2012).
Bloom, S. et al. A polycomponent metal‐catalyzed aliphatic, allylic, and benzylic fluorination. Angew. Chem. Int. Ed. 51, 10580–10583 (2012).
Liu, W. et al. Oxidative aliphatic C–H fluorination with fluoride ion catalyzed by a manganese porphyrin. Science 337, 1322–1325 (2012).
Xia, J.-B., Zhu, C. & Chen, C. Visible light-promoted metal-free C–H activation: diarylketone-catalyzed selective benzylic mono- and difluorination. J. Am. Chem. Soc. 135, 17494–17500 (2013).
Chatalova-Sazepin, C., Hemelaere, R., Paquin, J.-F. & Sammis, G. M. Recent advances in radical fluorination. Synthesis 47, 2554–2569 (2015).
Huang, X. et al. Late stage benzylic C–H fluorination with [18F]fluoride for PET imaging. J. Am. Chem. Soc. 136, 6842–6845 (2014).
Zhang, F.-L., Hong, K., Li, T.-J., Park, H. & Yu, J.-Q. Functionalization of C(sp 3)–H bonds using a transient directing group. Science 351, 252–256 (2016).
Engle, K. M., Mei, T.-S., Wang, X. & Yu, J.-Q. Bystanding F+ oxidants enable selective reductive elimination from high-valent metal centers in catalysis. Angew. Chem. Int. Ed. 50, 1478–1491 (2011).
Yahav, A., Goldberg, I. & Vigalok, A. Synthesis of the elusive (R3P)2MF2 (M = Pd, Pt) complexes. J. Am. Chem. Soc. 125, 13634–13635 (2003).
Rosewall, C. F., Sibbald, P. A., Liskin, D. V. & Michael, F. E. Palladium-catalyzed carboamination of alkenes promoted by N-fluorobenzenesulfonimide via C−H activation of arenes. J. Am. Chem. Soc. 131, 9488–9489 (2009).
Pérez-Temprano, M. H., Racowski, J. M., Kampf, J. W. & Sanford, M. S. Competition between sp 3-C–N vs. sp 3-C–F reductive elimination from PdIV complexes. J. Am. Chem. Soc. 136, 4097–4100 (2014).
Camasso, N. M., Pérez-Temprano, M. H. & Sanford, M. S. C(sp 3)–O bond-forming reductive elimination from PdIV with diverse oxygen nucleophiles. J. Am. Chem. Soc. 136, 12771–12775 (2014).
Sibbald, P. A., Rosewall, C. F., Swartz, R. D. & Michael, F. E. Mechanism of N-fluorobenzenesulfonimide promoted diamination and carboamination reactions: divergent reactivity of a Pd(iv) species. J. Am. Chem. Soc. 131, 15945–15951 (2009).
Wang, X., Mei, T.-S. & Yu, J.-Q. Versatile Pd(OTf)2·2H2O-catalyzed ortho-fluorination using NMP as a promoter. J. Am. Chem. Soc. 131, 7520–7521 (2009).
Chan, K. S. L., Wasa, M., Wang, X. & Yu, J.-Q. Palladium(ii)-catalyzed selective monofluorination of benzoic acids using a practical auxiliary: a weak-coordination approach. Angew. Chem. Int. Ed. 50, 9081–9084 (2011).
He, G., Lu, G., Guo, Z., Liu, P. & Chen, G. Benzazetidine synthesis via palladium-catalysed intramolecular C−H amination. Nat. Chem. 8, 1131–1136 (2016).
Katcher, M. H., Norrby, P.-O. & Doyle, A. G. Mechanistic investigations of palladium-catalyzed allylic fluorination. Organometallics 33, 2121–2133 (2014).
Racowski, J. M. & Sanford, M. S. Carbon-heteroatom bond-forming reductive elimination from palladium(iv) complexes. Top. Organomet. Chem. 35, 61–84.
Chen, K., Zhang, S.-Q., Jiang, H.-Z., Xu, J.-W. & Shi, B.-F. Practical synthesis of anti-β-hydroxy-α-amino acids by PdII-catalyzed sequential C(sp 3)–H functionalization. Chem. Eur. J. 21, 3264–3270 (2015).
Canty, A. J. et al. Computational study of C(sp 3)–O bond formation at a PdIV centre. Dalton Trans. 46, 3742–3748 (2017).
Geier, M. J., Dadkhah Aseman, M. & Gagné, M. R. Anion-dependent switch in C–X reductive elimination diastereoselectivity. Organometallics 33, 4353–4356 (2014).
Furuya, T. et al. Mechanism of C−F reductive elimination from palladium(iv) fluorides. J. Am. Chem. Soc. 132, 3793–3807 (2010).
Racowski, J. M., Gary, J. B. & Sanford, M. S. Carbon(sp 3)–fluorine bond-forming reductive elimination from palladium(iv) complexes. Angew. Chem. Int. Ed. 51, 3414–3417 (2012).
Kaspi, A. W., Yahav-Levi, A., Goldberg, I. & Vigalok, A. Xenon difluoride induced aryl iodide reductive elimination: a simple access to difluoropalladium(ii) complexes. Inorg. Chem. 47, 5–7 (2008).
Zhao, S.-B., Becker, J. J. & Gagné, M. R. Steric crowding makes challenging Csp 3–F reductive eliminations feasible. Organometallics 30, 3926–3929 (2011).
McMurtrey, K. B., Racowski, J. M. & Sanford, M. S. Pd-catalyzed C–H fluorination with nucleophilic fluoride. Org. Lett. 14, 4094–4097 (2012).
Hynes, J. B. & Campbell, J. P. Synthesis of 2-aminoquinazolines from ortho-fluorobenzaldehydes. J. Heterocycl. Chem. 34, 385–387 (1997).
Niu, X. et al. An efficient one-pot synthesis of 1,2,4-triazoloquinoxalines. Tetrahedron 70, 4657–4660 (2014).
Acknowledgements
The authors acknowledge financial support from The Scripps Research Institute, the National Institutes of Health (NIGMS, 2R01GM084019) and Shanghai RAAS Blood Products Co. H.P. thanks the Korea Foundation for Advanced Studies and Eli Lilly for graduate fellowship.
Author information
Authors and Affiliations
Contributions
H.P. developed the enantioselective fluorination reaction. H.P. and K.H. expanded the substrate scope. P.V. conducted the computational studies. J.-Q.Y. conceived and supervised the project.
Corresponding author
Ethics declarations
Competing interests
The authors declare no competing interests.
Additional information
Publisher’s note: Springer Nature remains neutral with regard to jurisdictional claims in published maps and institutional affiliations.
Supplementary information
Supplementary information
Supplementary experimental data, synthetic procedures and chemical compound characterization data
Crystallographic data
CIF for compound 5a; CCDC reference: 1556389
Crystallographic data
CIF for compound 6; CCDC reference: 1556390
Crystallographic data
CIF for compound 8; CCDC reference: 1577327
Rights and permissions
About this article
Cite this article
Park, H., Verma, P., Hong, K. et al. Controlling Pd(iv) reductive elimination pathways enables Pd(ii)-catalysed enantioselective C(sp3)−H fluorination. Nature Chem 10, 755–762 (2018). https://doi.org/10.1038/s41557-018-0048-1
Received:
Accepted:
Published:
Issue Date:
DOI: https://doi.org/10.1038/s41557-018-0048-1
This article is cited by
-
Transition metal-catalysed directed C–H functionalization with nucleophiles
Nature Synthesis (2022)
-
Unconventional mechanism and selectivity of the Pd-catalyzed C–H bond lactonization in aromatic carboxylic acid
Nature Communications (2022)
-
Contemporary synthetic strategies in organofluorine chemistry
Nature Reviews Methods Primers (2021)
-
C–C bond activation enabled by dyotropic rearrangement of Pd(iv) species
Nature Chemistry (2021)
-
Transient directing ligands for selective metal-catalysed C–H activation
Nature Reviews Chemistry (2021)