Abstract
Tomato (Solanum lycopersicum) is a major crop plant and a model system for fruit development. Solanum is one of the largest angiosperm genera1 and includes annual and perennial plants from diverse habitats. Here we present a high-quality genome sequence of domesticated tomato, a draft sequence of its closest wild relative, Solanum pimpinellifolium2, and compare them to each other and to the potato genome (Solanum tuberosum). The two tomato genomes show only 0.6% nucleotide divergence and signs of recent admixture, but show more than 8% divergence from potato, with nine large and several smaller inversions. In contrast to Arabidopsis, but similar to soybean, tomato and potato small RNAs map predominantly to gene-rich chromosomal regions, including gene promoters. The Solanum lineage has experienced two consecutive genome triplications: one that is ancient and shared with rosids, and a more recent one. These triplications set the stage for the neofunctionalization of genes controlling fruit characteristics, such as colour and fleshiness.
Similar content being viewed by others
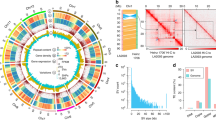
Main
The genome of the inbred tomato cultivar ‘Heinz 1706’ was sequenced and assembled using a combination of Sanger and ‘next generation’ technologies (Supplementary Information section 1). The predicted genome size is approximately 900 megabases (Mb), consistent with previous estimates3, of which 760 Mb were assembled in 91 scaffolds aligned to the 12 tomato chromosomes, with most gaps restricted to pericentromeric regions (Fig. 1A and Supplementary Fig. 1). Base accuracy is approximately one substitution error per 29.4 kilobases (kb) and one indel error per 6.4 kb. The scaffolds were linked with two bacterial artificial chromosome (BAC)-based physical maps and anchored/oriented using a high-density genetic map, introgression line mapping and BAC fluorescence in situ hybridization (FISH).
A, Multi-dimensional topography of tomato chromosome 1 (chromosomes 2–12 are shown in Supplementary Fig. 1). a, Left: contrast-reversed, 4′,6-diamidino-2-phenylindole (DAPI)-stained pachytene chromosome; centre and right: FISH signals for repeat sequences on diagrammatic pachytene chromosomes (purple, TGR1; blue, TGR4; red, telomere repeat; green, Cot 100 DNA (including most repeats)). b, Frequency distribution of recombination nodules (RNs) representing crossovers on 249 chromosomes. Red stars mark 5 cM intervals starting from the end of the short arm (top). Scale is in micrometres. c, FISH-based locations of selected BACs (horizontal blue lines on left). d, Kazusa F2-2000 linkage map. Blue lines to the left connect linkage map markers on the BAC-FISH map (c), and to the right to heat maps (e) and the DNA pseudomolecule (f). e, From left to right: linkage map distance (cM/Mb, turquoise), repeated sequences (% nucleotides per 500 kb, purple), genes (% nucleotides per 500 kb, blue), chloroplast insertions; RNA-Seq reads from leaves and breaker fruits of S. lycopersicum and S. pimpinellifolium (number of reads per 500 kb, green and red, respectively), microRNA genes (transcripts per million per 500 kb, black), small RNAs (thin horizontal black and red lines, sum of hits-normalized abundances). Horizontal grey lines represent gaps in the pseudomolecule (f). f, DNA pseudomolecule consisting of nine scaffolds. Unsequenced gaps (approximately 9.8 Mb, Supplementary Table 13) are indicated by white horizontal lines. Tomato genes identified by map-based cloning (Supplementary Table 14) are indicated on the right. For more details, see legend to Supplementary Fig. 1. B, Syntenic relationships in the Solanaceae. COSII-based comparative maps of potato, aubergine (eggplant), pepper and Nicotiana with respect to the tomato genome (Supplementary Information section 4.5 and Supplementary Fig. 14). Each tomato chromosome is assigned a different colour and orthologous chromosome segment(s) in other species are shown in the same colour. White dots indicate approximate centromere locations. Each black arrow indicates an inversion relative to tomato and ‘+1’ indicates a minimum of one inversion. Each black bar beside a chromosome indicates translocation breakpoints relative to tomato. Chromosome lengths are not to scale, but segments within chromosomes are. C, Tomato–potato syntenic relationships dot plot of tomato (T) and potato (P) genomic sequences based on collinear blocks (Supplementary Information section 4.1). Red and blue dots represent gene pairs with statistically significant high and low ω (Ka/Ks) in collinear blocks, which average Ks ≤ 0.5, respectively. Green and magenta dots represent genes in collinear blocks which average 0.5 < Ks ≤ 1.5 and Ks > 1.5, respectively. Yellow dots represent all other gene pairs. Blocks circled in red are examples of pan-eudicot triplication. Inserts represent schematic drawings of BAC-FISH patterns of cytologically demonstrated chromosome inversions (also in Supplementary Fig. 15).
The genome of S. pimpinellifolium LA1589 was sequenced and assembled de novo using Illumina short reads, yielding a 739 Mb draft genome (Supplementary Information section 3). Estimated divergence between the wild and domesticated genomes is 0.6% (5.4 million single nucleotide polymorphisms (SNPs) distributed along the chromosomes (Fig. 1A and Supplementary Fig. 1)).
Tomato chromosomes consist of pericentric heterochromatin and distal euchromatin, with repeats concentrated within and around centromeres, in chromomeres and at telomeres (Fig. 1A and Supplementary Fig. 1). Substantially higher densities of recombination, genes and transcripts are observed in euchromatin, whereas chloroplast insertions (Supplementary Information sections 1.22 and 1.23) and conserved microRNA (miRNA) genes (Supplementary Information section 2.9) are more evenly distributed throughout the genome. The genome is highly syntenic with those of other economically important Solanaceae (Fig. 1B). Compared to the genomes of Arabidopsis4 and Sorghum5, tomato has fewer high-copy, full-length long terminal repeat (LTR) retrotransposons with older average insertion ages (2.8 versus 0.8 million years (Myr) ago) and fewer high-frequency k-mers (Supplementary Information section 2.10). This supports previous findings that the tomato genome is unusual among angiosperms by being largely comprised of low-copy DNA6,7.
The pipeline used to annotate the tomato and potato8 genomes is described in Supplementary Information section 2. It predicted 34,727 and 35,004 protein-coding genes, respectively. Of these, 30,855 and 32,988, respectively, are supported by RNA sequencing (RNA-Seq) data, and 31,741 and 32,056, respectively, show high similarity to Arabidopsis genes (Supplementary Information section 2.1). Chromosomal organization of genes, transcripts, repeats and small RNAs (sRNAs) is very similar in the two species (Supplementary Figs 2–4). The protein-coding genes of tomato, potato, Arabidopsis, rice and grape were clustered into 23,208 gene groups (≥2 members), of which 8,615 are common to all five genomes, 1,727 are confined to eudicots (tomato, potato, grape and Arabidopsis), and 727 are confined to plants with fleshy fruits (tomato, potato and grape) (Supplementary Information section 5.1 and Supplementary Fig. 5). Relative expression of all tomato genes was determined by replicated strand-specific Illumina RNA-Seq of root, leaf, flower (two stages) and fruit (six stages) in addition to leaf and fruit (three stages) of S. pimpinellifolium (Supplementary Table 1).
sRNA sequencing data supported the prediction of 96 conserved miRNA genes in tomato and 120 in potato, a number consistent with other plant species (Fig. 1A, Supplementary Figs 1 and 3 and Supplementary Information section 2.9). Among the 34 miRNA families identified, 10 are highly conserved in plants and similarly represented in the two species, whereas other, less conserved families are more abundant in potato. Several miRNAs, predicted to target Toll interleukin receptor, nucleotide-binding site and leucine-rich repeat (TIR-NBS-LRR) genes, seemed to be preferentially or exclusively expressed in potato (Supplementary Information section 2.9).
Comparative genomic studies are reported in Supplementary Information section 4. Sequence alignment of 71 Mb of euchromatic tomato genomic DNA to their potato8 counterparts revealed 8.7% nucleotide divergence (Supplementary Information section 4.1). Intergenic and repeat-rich heterochromatic sequences showed more than 30% nucleotide divergence, consistent with the high sequence diversity in these regions among potato genotypes8. Alignment of tomato–potato orthologous regions confirmed nine large inversions known from cytological or genetic studies and several smaller ones (Fig. 1C). The exact number of small inversions is difficult to determine due to the lack of orientation of most potato scaffolds.
A total of 18,320 clearly orthologous tomato–potato gene pairs were identified. Of these, 138 (0.75%) had significantly higher than average non-synonymous (Ka) versus synonymous (Ks) nucleotide substitution rate ratios (ω), indicating diversifying selection, whereas 147 (0.80%) had significantly lower than average ω, indicating purifying selection (Supplementary Table 2). The proportions of high and low ω between sorghum and maize (Zea mays) are 0.70% and 1.19%, respectively, after 11.9 Myr of divergence9, indicating that diversifying selection may have been stronger in tomato–potato. The highest densities of low-ω genes are found in collinear blocks with average Ks > 1.5, tracing to a genome triplication shared with grape (see below) (Fig. 1C, Supplementary Fig. 6 and Supplementary Table 3). These genes, which have been preserved in paleo-duplicated locations for more than 100 Myr10,11, are more constrained than ‘average’ genes and are enriched for transcription factors and genes otherwise related to gene regulation (Supplementary Tables 3 and 4).
Sequence comparison of 31,760 Heinz 1706 genes with >5× S. pimpinellifolium read coverage in over 90% of their coding regions revealed 7,378 identical genes and 11,753 with only synonymous changes. The remaining 12,629 genes had non-synonymous changes, including gains and losses of stop codons with potential consequences for gene function (Supplementary Tables 5–7). Several pericentric regions, predicted to contain genes, are absent or polymorphic in the broader S. pimpinellifolium germplasm (Supplementary Table 8 and Supplementary Fig. 7). Within cultivated germplasm, particularly among the small-fruited cherry tomatoes, several chromosomal segments are more closely related to S. pimpinellifolium than to Heinz 1706 (Supplementary Figs 8 and 9), supporting previous observations on recent admixture of these gene pools due to breeding12. Heinz 1706 itself has been reported to carry introgressions from S. pimpinellifolium13, traces of which are detectable on chromosomes 4, 9, 11 and 12 (Supplementary Table 9).
Comparison of the tomato and grape genomes supports the hypothesis that a whole-genome triplication affecting the rosid lineage occurred in a common eudicot ancestor11 (Fig. 2a). The distribution of Ks between corresponding gene pairs in duplicated blocks suggests that one polyploidization in the solanaceous lineage preceded the rosid–asterid (tomato–grape) divergence (Supplementary Fig. 10).
a, Speciation and polyploidization in eudicot lineages. Confirmed whole-genome duplications and triplications are shown with annotated circles, including ‘T’ (this paper) and previously discovered events α, β, γ10,11,14. Dashed circles represent one or more suspected polyploidies reported in previous publications that need further support from genome assemblies27,28. Grey branches indicate unpublished genomes. Black and red error bars bracket indicate the likely timings of divergence of major asterid lineages and of ‘T’, respectively. The post-‘T’ subgenomes, designated T1, T2, and T3, are further detailed in Supplementary Fig. 10. b, On the basis of alignments of multiple tomato genome segments to single grape genome segments, the tomato genome is partitioned into three non-overlapping ‘subgenomes’ (T1, T2, T3), each represented by one axis in the three-dimensional plot. The ancestral gene order of each subgenome is inferred according to orthologous grape regions, with tomato chromosomal affinities shown by red (inner) bars. Segments tracing to pan-eudicot triplication (γ) are shown by green (outer) bars with colours representing the seven putative pre-γ eudicot ancestral chromosomes10, also coded a–g.
Comparison with the grape genome also reveals a more recent triplication in tomato and potato. Whereas few individual tomato/potato genes remain triplicated (Supplementary Tables 10 and 11), 73% of tomato gene models are in blocks that are orthologous to one grape region, collectively covering 84% of the grape gene space. Among these grape genomic regions, 22.5% have one orthologous region in tomato, 39.9% have two, and 21.6% have three, indicating that a whole-genome triplication occurred in the Solanum lineage, followed by widespread gene loss. This triplication, also evident in potato (Supplementary Fig. 11), is estimated at 71 (±19.4) Myr on the basis of the Ks of paralogous genes (Supplementary Fig. 10), and therefore predates the ∼7.3 Myr tomato–potato divergence. On the basis of alignments to single grape genome segments, the tomato genome can be partitioned into three non-overlapping ‘subgenomes’ (Fig. 2b). The number of euasterid lineages that have experienced the recent triplication remains unclear and awaits complete euasterid I and II genome sequences. Ks distributions show that euasterids I and II, and indeed the rosid–asterid lineages, all diverged from common ancestry at or near the pan-eudicot triplication (Fig. 2a), suggesting that this event may have contributed to the formation of major eudicot lineages in a short period of several million years14, partially explaining the explosive radiation of angiosperm plants on Earth15.
Fleshy fruits (Supplementary Fig. 12) are an important means of attracting vertebrate frugivores for seed dispersal16. Combined orthology and synteny analyses indicate that both genome triplications added new gene family members that mediate important fruit-specific functions (Fig. 3). These include transcription factors and enzymes necessary for ethylene biosynthesis (RIN, CNR, ACS) and perception (ETR3/NR, ETR4)17, red light photoreceptors influencing fruit quality (PHYB1/PHYB2) and ethylene- and light-regulated genes mediating lycopene biosynthesis (PSY1/PSY2). Several cytochrome P450 subfamilies associated with toxic alkaloid biosynthesis show contraction or complete loss in tomato and the extant genes show negligible expression in ripe fruits (Supplementary Information section 5.4).
The genes shown represent a fruit ripening control network regulated by transcription factors (MADS-RIN, CNR) necessary for production of the ripening hormone ethylene, the production of which is regulated by ACC synthase (ACS). Ethylene interacts with ethylene receptors (ETRs) to drive expression changes in output genes, including phytoene synthase (PSY), the rate-limiting step in carotenoid biosynthesis. Light, acting through phytochromes, controls fruit pigmentation through an ethylene-independent pathway. Paralogous gene pairs with different physiological roles (MADS1/RIN, PHYB1/PHYB2, ACS2/ACS6, ETR3/ETR4, PSY1/PSY2), were generated during the eudicot (γ, black circle) or the more recent Solanum (T, red circle) triplications. Complete dendrograms of the respective protein families are shown in Supplementary Figs 16 and 17.
Fruit texture has profound agronomic and sensory importance and is controlled in part by cell wall structure and composition18. More than 50 genes showing differential expression during fruit development and ripening encode proteins involved in modification of cell wall architecture (Fig. 4a and Supplementary Information section 5.7). For example, a family of xyloglucan endotransglucosylase/hydrolases (XTHs) has expanded both in the recent whole-genome triplication and through tandem duplication. One of the triplicated members, XTH10, shows differential loss between tomato and potato (Fig. 4a and Supplementary Table 12), suggesting genetically driven specialization in the remodelling of fruit cell walls.
a, Xyloglucan transglucosylase/hydrolases (XTHs) differentially expressed between mature green and ripe fruits (Supplementary Information section 5.7). These XTH genes and many others are expressed in ripening fruits and are linked with the Solanum triplication, marked with a red circle on the phylogenetic tree. Red lines on the tree denote paralogues derived from the Solanum triplication, and blue lines are tandem duplications. b, Developmentally regulated accumulation of sRNAs mapping to the promoter region of a fruit-regulated cell wall gene (pectin acetylesterase, Solyc08g005800). Variation of abundance of sRNAs (left) and messenger RNA expression levels from the corresponding gene (right) over a tomato fruit developmental series (T1, bud; T2, flower; T3, fruit 1–3 mm; T4, fruit 5–7 mm; T5, fruit 11–13 mm; T6, fruit mature green; T7, breaker; T8, breaker + 3 days; T9, breaker + 7 days). The promoter regions are grouped in 100-nucleotide windows. For each window the size class distribution of sRNAs is shown (red, 21; green, 22; orange, 23; blue, 24). The height of the box corresponding to the first time point shows the cumulative sRNA abundance in log scale. The height of the following boxes is proportional to the log offset fold change (offset = 20) relative to the first time point. The expression profile of the mRNA is shown in log2 scale. The horizontal black line represents 1 kb of the promoter region. 0 to 12 represent arbitrary units of gene expression.
Similar to soybean and potato and in contrast to Arabidopsis, tomato sRNAs map preferentially to euchromatin (Supplementary Fig. 2). sRNAs from tomato flowers and fruits19 map to 8,416 gene promoters. Differential expression of sRNAs during fruit development is apparent for 2,687 promoters, including those of cell-wall-related genes (Fig. 4b) and occurs preferentially at key developmental transitions (for example, flower to fruit, fruit growth to fruit ripening, Supplementary Information section 2.8).
The genome sequences of tomato, S. pimpinellifolium and potato provide a starting point for comparing gene family evolution and sub-functionalization in the Solanaceae. A striking example is the SELF PRUNING (SP) gene family, which includes the homologue of Arabidopsis FT, encoding the mobile flowering signal florigen20 and its antagonist SP, encoding the orthologue of TFL1. Nearly a century ago, a spontaneous mutation in SP spawned the ‘determinate’ varieties that now dominate the tomato mechanical harvesting industry21. The genome sequence has revealed that the SP family has expanded in the Solanum lineage compared to Arabidopsis, driven by the Solanum triplication and tandem duplication (Supplementary Fig. 13). In potato, SP3D and SP6A control flowering and tuberization, respectively22, whereas SP3D in tomato, known as SINGLE FLOWER TRUSS, similarly controls flowering, but also drives heterosis for fruit yield in an epistatic relationship with SP23,24,25. Interestingly, SP6A in S. lycopersicum is inactivated by a premature stop codon, but remains functionally intact in S. pimpinellifolium. Thus, allelic variation in a subset of SP family genes has played a major role in the generation of both shared and species-specific variation in solanaceous agricultural traits.
The genome sequences of tomato and S. pimpinellifolium also provide a basis for understanding the bottlenecks that have narrowed tomato genetic diversity: the domestication of S. pimpinellifolium in the Americas, the export of a small number of genotypes to Europe in the 16th century, and the intensive breeding that followed. Charles Rick pioneered the use of trait introgression from wild tomato relatives to increase genetic diversity of cultivated tomatoes26. Introgression lines exist for seven wild tomato species, including S. pimpinellifolium, in the background of cultivated tomato. The genome sequences presented here and the availability of millions of SNPs will allow breeders to revisit this rich trait reservoir and identify domestication genes, providing biological knowledge and empowering biodiversity-based breeding.
Methods Summary
A total of 21 gigabases (Gb) of Roche/454 Titanium shotgun and mate pair reads and 3.3 Gb of Sanger paired-end reads, including ∼200,000 BAC and fosmid end sequence pairs, were generated from the ‘Heinz 1706’ inbred line (Supplementary Information sections 1.1–1.7), assembled using both Newbler and CABOG and integrated into a single assembly (Supplementary Information sections 1.17 and 1.18). The scaffolds were anchored using two BAC-based physical maps, one high density genetic map, overgo hybridization and genome-wide BAC FISH (Supplementary Information sections 1.8–1.16 and 1.19). Over 99.9% of BAC/fosmid end pairs mapped consistently on the assembly and over 98% of EST sequences could be aligned to the assembly (Supplementary Information section 1.20). Chloroplast genome insertions in the nuclear genome were validated using a mate pair method and the flanking regions were identified (Supplementary Information sections 1.22–1.24). Annotation was carried out using a pipeline based on EuGene that integrates de novo gene prediction, RNA-Seq alignment and rich function annotation (Supplementary Information section 2). To facilitate interspecies comparison, the potato genome was re-annotated using the same pipeline. LTR retrotransposons were detected de novo with the LTR-STRUC program and dated by the sequence divergence between left and right solo LTR (Supplementary Information section 2.10). The genome of S. pimpinellifolium was sequenced to ×40 depth using Illumina paired end reads and assembled using ABySS (Supplementary Information section 3). The tomato and potato genomes were aligned using LASTZ (Supplementary Information section 4.1). Identification of triplicated regions was done using BLASTP, in-house-generated scripts and three-way comparisons between tomato, potato and S. pimpinellifolium using MCSCAN (Supplementary Information sections 4.2–4.4). Specific gene families/groups (genes for ascorbate, carotenoid and jasmonate biosynthesis, cytochrome P450s, genes controlling cell wall architecture, hormonal and transcriptional regulators, resistance genes) were subjected to expert curation/analysis (Supplementary Information section 5). PHYML and MEGA were used to reconstruct phylogenetic trees and MCSCAN was used to infer gene collinearity (Supplementary Information section 5.2).
Accession codes
Primary accessions
GenBank/EMBL/DDBJ
Data deposits
The genomic data generated by the whole project are available in GenBank as accession number AEKE00000000, and the individual chromosome sequences as numbersCM001064–CM001075. TheRNA-Seq data are available in the Sequence Read Archive under accession number SRA049915, GSE33507, SRA050797 and SRA048144. Further information on data access can be found in Supplementary Information section 2.2.
References
Frodin, D. G. History and concepts of big plant genera. Taxon 53, 753–776 (2004)
Peralta, I. E., Spooner, D. M. & Knapp, S. Taxonomy of tomatoes: a revision of wild tomatoes (Solanum section Lycopersicon) and their outgroup relatives in sections Juglandifolia and Lycopersicoides . Syst. Bot. Monogr. 84, 1–186 (2008)
Michaelson, M. J., Price, H. J., Ellison, J. R. & Johnston, J. S. Comparison of plant DNA contents determined by Feulgen microspectrophotometry and laser flow cytometry. Am. J. Bot. 78, 183–188 (1991)
The Arabidopsis Genome Initiative. Analysis of the genome sequence of the flowering plant Arabidopsis thaliana . Nature 408, 796–815 (2000)
Paterson, A. H. et al. The Sorghum bicolor genome and the diversification of grasses. Nature 457, 551–556 (2009)
Zamir, D. & Tanksley, S. D. Tomato genome is comprised largely of fast-evolving, low copy-number sequences. Mol. Gen. Genet. 213, 254–261 (1988)
Peterson, D. G., Pearson, W. R. & Stack, S. M. Characterization of the tomato (Lycopersicon esculentum) genome using in vitro and in situ DNA reassociation. Genome 41, 346–356 (1998)
Xu, X. et al. Genome sequence and analysis of the tuber crop potato. Nature 475, 189–195 (2011)
Swigoňová, Z. et al. Close split of sorghum and maize genome progenitors. Genome Res. 14, 1916–1923 (2004)
Jaillon, O. et al. The grapevine genome sequence suggests ancestral hexaploidization in major angiosperm phyla. Nature 449, 463–467 (2007)
Tang, H. et al. Synteny and collinearity in plant genomes. Science 320, 486–488 (2008)
Ranc, N., Munos, S., Santoni, S. & Causse, M. A clarified position for Solanum lycopersicum var. cerasiforme in the evolutionary history of tomatoes (solanaceae). BMC Plant Biol. 8, 130 (2008)
Ozminkowski, R. Pedigree of variety Heinz 1706. Rep. Tomato Genet. Coop. 54, 26 (2004)
Moore, M. J., Soltis, P. S., Bell, C. D., Burleigh, J. G. & Soltis, D. E. Phylogenetic analysis of 83 plastid genes further resolves the early diversification of eudicots. Proc. Natl Acad. Sci. USA 107, 4623–4628 (2010)
Stockey, R. A., Graham, S. W. & Crane, P. R. Introduction to the Darwin special issue: the abominable mystery. Am. J. Bot. 96, 3–4 (2009)
Howe, H. F. & Smallwood, J. Ecology of seed dispersal. Annu. Rev. Ecol. Syst. 13, 201–228 (1982)
Klee, H. J. & Giovannoni, J. J. Genetics and control of tomato fruit ripening and quality attributes. Annu. Rev. Genet. 45, 41–59 (2011)
Vicente, A. R., Saladie, M., Rose, J. K. C. & Labavitch, J. M. The linkage between cell wall metabolism and fruit softening: looking to the future. J. Sci. Food Agric. 87, 1435–1448 (2007)
Mohorianu, I. et al. Profiling of short RNAs during fleshy fruit development reveals stage-specific sRNAome expression patterns. Plant J. 67, 232–246 (2011)
Corbesier, L. et al. FT protein movement contributes to long-distance signaling in floral induction of Arabidopsis . Science 316, 1030–1033 (2007)
Rick, C. M. The tomato. Sci. Am. 239, 76–87 (1978)
Navarro, C. et al. Control of flowering and storage organ formation in potato by FLOWERING LOCUS T. Nature 478, 119–122 (2011)
Lifschitz, E. et al. The tomato FT ortholog triggers systemic signals that regulate growth and flowering and substitute for diverse environmental stimuli. Proc. Natl Acad. Sci. USA 103, 6398–6403 (2006)
Krieger, U., Lippman, Z. B. & Zamir, D. The flowering gene SINGLE FLOWER TRUSS drives heterosis for yield in tomato. Nature Genet. 42, 459–463 (2010)
Pnueli, L. et al. The SELF-PRUNING gene of tomato regulates vegetative to reproductive switching of sympodial meristems and is the ortholog of CEN and TFL1 . Development 125, 1979–1989 (1998)
Rick, C. M. Hybridization between Lycopersicon esculentum and Solanum pennellii: phylogenetic and cytogenetic significance. Proc. Natl Acad. Sci. USA 46, 78–82 (1960)
Barker, M. S. et al. Multiple paleopolyploidizations during the evolution of the Compositae reveal parallel patterns of duplicate gene retention after millions of years. Mol. Biol. Evol. 25, 2445–2455 (2008)
Aagaard, J. E., Willis, J. H. & Phillips, P. C. Relaxed selection among duplicate floral regulatory genes in Lamiales. J. Mol. Evol. 63, 493–503 (2006)
Acknowledgements
This work was supported by: Argentina: INTA and CONICET. Belgium: Flemish Institute for Biotechnology and Ghent University. China: The State Key Laboratory of Plant Genomics, Institute of Genetics and Developmental Biology, Chinese Academy of Sciences; Ministry of Science and Technology (2006AA10A116, 2004CB720405, 2006CB101907, 2007DFB30080) Ministry of Agriculture (‘948’ Program: 2007-Z5); National Natural Science Foundation (36171319); Postdoctoral Science Foundation (20070420446). European Union: FP6 Integrated Project EU-SOL PL 016214. France: Institute National de la Recherche Agronomique and Agence Nationale de la Recherche. Germany: the Max Planck Society. India: Department of Biotechnology, Government of India; Indian Council of Agricultural Research. Italy: Ministry of Research (FIRB-SOL, FIRB-Parallelomics, ItaLyco and GenoPOM projects); Ministry of Agriculture (Agronanotech and Biomassval projects); FILAS foundation; ENEA; CNR-ENEA project L. 191/2009. Japan: Kazusa DNA Research Institute Foundation and National Institute of Vegetable and Tea Science. Korea: KRIBB Basic Research Fund and Crop Functional Genomics Research Center (CFGC), MEST. Netherlands: Centre for BioSystems Genomics, Netherlands Organization for Scientific Research. Spain: Fundación Genoma España; Cajamar; FEPEX; Fundación Séneca; ICIA; IFAPA; Fundación Manrique de Lara; Instituto Nacional de Bioinformatica. UK: BBSRC grant BB/C509731/1; DEFRA; SEERAD. USA: NSF (DBI-0116076; DBI-0421634; DBI-0606595; IOS-0923312; DBI-0820612; DBI-0605659; DEB-0316614; DBI 0849896 and MCB 1021718); USDA (2007-02773 and 2007-35300-19739); USDA-ARS. We acknowledge the Potato Genome Sequencing Consortium for sharing data before publication; potato RNA-Seq data was provided by C. R. Buell from the NSF-funded Potato Genome Sequence and Annotation project; tomato RNA-Seq data by the USDA-funded SolCAP project, N. Sinha and J. Maloof; the Amplicon Express team for BAC pooling services; construction of the Whole Genome Profiling (WGP) physical map was supported by EnzaZaden, RijkZwaan, Vilmorin & Cie, and Takii & Co. Keygene N.V. owns patents and patent applications covering its AFLP and Whole Genome Profiling technologies; AFLP and Keygene are registered trademarks of Keygene N.V. The following individuals are also acknowledged for their contribution to the work described: J. Park, B. Wang, C. Niu, D. Liu, F. Cojutti, S. Pescarolo, A. Zambon, G. Xiao, J. Chen, J. Shi, L. Zhang, L. Zeng, M. Caccamo, D. Bolser, D. Martin, M. Gonzalez, P. A. Bedinger, P. A. Covey, P. Pachori, R. R. Pousada, S. Hakim, S. Sims, V. Cahais, W. Long, X. Zhou, Y. Lu, W. Haso, C. Lai, S. Lepp, C. Peluso, H. Teramu, H. De Jong, R. Lizarralde, E. R. May and Z. Li. M. Zabeau is thanked for his support and encouragement and S. van den Brink for her secretarial support. We dedicate this work to the late C. Rick who pioneered tomato genetics, collection of wild germplasm and the distribution of seed and knowledge.
Author information
Authors and Affiliations
Consortia
Contributions
For full details of author contributions, please see the Supplementary Information.
Corresponding authors
Ethics declarations
Competing interests
The author declare no competing financial interests.
Supplementary information
Supplementary Information
This file contains Supplementary Methods, Supplementary Results, Supplementary Figures 1-56 and additional references –see Contents for details. (PDF 16885 kb)
Supplementary Tables
This zipped excel file contains Supplementary Tables 1-78. (ZIP 29428 kb)
Supplementary Tables
This zipped archive contains Supplementary HTM Tables 1-269. The tables can be opened by any web browser and contain additional text that can be visualized by placing the mouse over the image. (ZIP 250 kb)
Rights and permissions
This article is distributed under the terms of the Creative Commons Attribution-Non-Commercial-Share Alike licence (http://creativecommons.org/licenses/by-nc-sa/3.0/).
About this article
Cite this article
The Tomato Genome Consortium. The tomato genome sequence provides insights into fleshy fruit evolution. Nature 485, 635–641 (2012). https://doi.org/10.1038/nature11119
Received:
Accepted:
Published:
Issue Date:
DOI: https://doi.org/10.1038/nature11119
This article is cited by
-
Optimized protocols for protoplast isolation, transfection, and regeneration in the Solanum genus for the CRISPR/Cas-mediated transgene-free genome editing
Applied Biological Chemistry (2024)
-
Chromosome level genome assembly of endangered medicinal plant Anisodus tanguticus
Scientific Data (2024)
-
Genetics and evolutionary insights from Solanaceae genome sequences
Plant Systematics and Evolution (2024)
-
Comparative performance and transcriptomics of tomato (Solanum lycopersicum) in response to Bombus terrestris (Hymenoptera, Apidae) and 2,4-dichlorophenoxyacetic acid
Arthropod-Plant Interactions (2024)
-
Transcriptional and epigenetic changes during tomato yellow leaf curl virus infection in tomato
BMC Plant Biology (2023)
Comments
By submitting a comment you agree to abide by our Terms and Community Guidelines. If you find something abusive or that does not comply with our terms or guidelines please flag it as inappropriate.