Key Points
-
Arabidopsis thaliana is the model system of choice for plant molecular geneticists. Forward genetic screens in Arabidopsis offer a powerful means for the functional characterization of various aspects of the plant life cycle. • Morphological and brute force biochemical screens have been highly successful in identifying the genes that are involved in many aspects of plant metabolism and development.
-
The success of genetic screens depends on the appropriate genetic background, and an easy and tight procedure to identify mutants of interest.
-
Unbiased modifier screens often yield fundamental new insights into complex developmental processes.
-
Engineered genetic backgrounds often allow the isolation of mutants using selection rather than screening procedures.
-
Use of reporter genes allows the specific tailoring of genetic backgrounds for mutagenesis. This allows the construction of an easily scorable phenotype for nearly any hidden aspect of the plant's life cycle.
-
Insertional mutagenesis offers an efficient way to screen for gametophytic mutants by screening for a non-Mendelian segregation ratio distortion.
-
Taking advantage of natural variation bears great potential to gain insights into plant growth and development in an evolutionary context.
Abstract
Molecular genetic studies rely on well-characterized organisms that can be easily manipulated. Arabidopsis thaliana — the model system of choice for plant biologists — allows efficient analysis of plant function, combining classical genetics with molecular biology. Although the complete sequence of the Arabidopsis genome allows the rapid discovery of the molecular basis of a characterized mutant, functional characterization of the Arabidopsis genome depends on well-designed forward genetic screens, which remain a powerful strategy to identify genes that are involved in many aspects of the plant life cycle.
This is a preview of subscription content, access via your institution
Access options
Subscribe to this journal
Receive 12 print issues and online access
$189.00 per year
only $15.75 per issue
Buy this article
- Purchase on Springer Link
- Instant access to full article PDF
Prices may be subject to local taxes which are calculated during checkout






Similar content being viewed by others
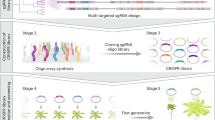
References
Rédei, G.P. in Methods in Arabidopsis Research (eds Koncz, C., Chua, N.-H & Schell, J.) 1–15 (World Scientific, Singapore, 1992).
Laibach, F. Arabidopsis thaliana (L.) Heynh. als Objekt für genetische und entwicklungsphysiologische Untersuchungen. Bot. Archiv. 44, 439–455 (1943).
The Arabidopsis Genome Initiative. Analysis of the genome sequence of the flowering plant Arabidopsis thaliana. Nature 408, 796–815 (2000).
Leutwiler, L. S., Hough-Evans, B. R. & Meyerowitz, E. M. The DNA of Arabidopsis thaliana. Mol. Gen. Genet. 194, 15–23 (1984).
Pruitt, R. E. & Meyerowitz, E. M. Characterization of the genome of Arabidopsis thaliana. J. Mol. Biol. 187, 169–183 (1986).
Meyerowitz, E. M. & Pruitt, R. E. Arabidopsis thaliana and plant molecular genetics. Science 229, 1214–1218 (1985).
Meyerowitz, E. M. Arabidopsis, a useful weed. Cell 56, 263–269 (1989).
Meinke, D. W., Cherry, J. M., Dean, C., Rounsley, S. D. & Koornneef, M. Arabidopsis thaliana: a model plant for genome analysis. Science 282, 679–682 (1998). | PubMed |
Röbbelen, G. Untersuchungen an strahleninduzierten Blattformmutanten von Arabidopsis thaliana (L.). Heynh. Z. Ind. Abst. Vererb.-Lehre 88, 189–252 (1957).
McKelvie, A. D. A list of mutant genes in Arabidopsis thaliana (L.). Heynh. Radiat. Bot. 1, 233–241 (1962).
Koornneef, M. et al. Linkage map of Arabidopsis thaliana. J. Hered. 74, 265–272 (1983).
Müller, A. J. Embryonentest zum Nachweis rezessiver Letalfaktoren bei Arabidopsis thaliana. Biol. Zentralbl. 82, 133–163 (1963).
Meinke, D. W. & Sussex, I. M. Embryo-lethal mutants of Arabidopsis thaliana: a model system for genetic analysis of plant embryo development. Dev. Biol. 72, 50–61 (1979).
Mayer, U., Torres-Ruiz, R. A., Berleth, T., Miséra, S. & Jürgens, G. Mutations affecting body organisation in the Arabidopsis embryo. Nature 353, 402–407 (1991).An extensive screen for seedling phenotypes that identified many genes involved in pattern formation during Arabidopsis embryogenesis.
Maher, E. P. & Martindale, S. J. Mutants of Arabidopsis thaliana with altered responses to auxins and gravity. Biochem. Genet. 18, 1041–1053 (1980).
Koornneef, M., Dellaert, L. W. & Van der Veen, J. H. EMS- and radiation-induced mutation frequencies at individual loci in Arabidopsis thaliana (L.) Heynh. Mutat. Res. 93, 109–123 (1982).
Koornneef, M., Reuling, G. & Karssen, C. M. The isolation and characterization of abscisic acid insensitive mutants of Arabidopsis thaliana. Physiol. Plant. 61, 377–383 (1984).
Bleecker, A. B., Estelle, M. A., Somerville, C. & Kende, H. Insensitivity to ethylene conferred by a dominant mutation in Arabidopsis thaliana. Science 241, 1086–1089 (1988).
Guzman, P. & Ecker, J. R. Exploiting the triple response of Arabidopsis to identify ethylene-related mutants. Plant Cell 2, 513–523 (1990).
Li, S. & Rédei, G. P. Estimation of mutation rates in autogamous diploids. Radiat. Bot. 9, 125–131 (1969).
Browse, J., McCourt, P. & Somerville, C. R. A mutant of Arabidopsis lacking a chloroplast-specific lipid. Science 227, 763–765 (1985).A pioneering example of a brute force biochemical screen that led to the genetic analysis of lipid biosynthesis in plants.
James, D. W. & Dooner, H. K. Isolation of EMS-induced mutants in Arabidopsis altered in seed fatty acid desaturation. Theor. Appl. Genet. 80, 241–245 (1990).
Lemieux, B., Miquel, M., Somerville, C. & Browse, J. Mutants of Arabidopsis with alterations in seed lipid fatty acid composition. Theor. Appl. Genet. 80, 234–240 (1990).
Quail, P. H. The phytochrome family: dissection of functional roles and signalling pathways among family members. Phil. Trans. R. Soc. Lond. B 353, 1399–1403 (1998).
Glazebrook, J., Rogers, E. E. & Aushubel, F. M. Use of Arabidopsis for genetic dissection of plant defense responses. Annu. Rev. Genet. 31, 547–569 (1997).A comprehensive review on the genetic dissection of plant–pathogen interactions in Arabidopsis.
Mittelsten-Scheid, O., Afsar, K. & Paszkowski, J. Release of epigenetic gene silencing by trans-acting mutations in Arabidopsis. Proc. Natl Acad. Sci. USA 95, 632–637 (1998).The first example of a mutagenesis screen for mutants that reactivate a silenced transgene.
Moore, J. M., Vielle-Calzada, J.-P., Gagliano, W. & Grossniklaus, U. Genetic characterization of hadad, a mutant disrupting female gametogenesis in Arabidopsis thaliana. Cold Spring Harb. Symp. Quant. Biol. 62, 35–47 (1997).
Bartee, L., Malagnac, F. & Bender, J. Arabidopsis cmt3 chromomethylase mutations block non-CG methylation and silencing of an endogenous gene. Genes Dev. 15, 1753–1758 (2001).An example of how characteristics of endogenous genes can be used to screen for a highly specific mutant phenotype.
Forsburg, S. L. The art and design of genetic screens: yeast. Nature Rev. Genet. 2, 659–668 (2001).
Thomas, J. H. Thinking about genetic redundancy. Trends Genet. 9, 395–399 (1993).
Eshed, Y., Baum, S. F. & Bowman, J. L. Distinct mechanisms promote polarity establishment in carpels of Arabidopsis. Cell 99, 199–209 (1999).Illustrates the power of an unbiased modifier screen, which led to the identification of genes that act in different pathways that are involved in establishing polarity in Arabidopsis carpels.
Bowman, J. L. & Smyth, D. R. CRABS CLAW, a gene that regulates carpel and nectary development in Arabidopsis, encodes a novel protein with zinc finger and helix–loop–helix domains. Development 126, 2387–2396 (1999).
Sawa, S. et al. FILAMENTOUS FLOWER, a meristem and organ identity gene of Arabidopsis, encodes a protein with a zinc finger and HMG-related domains. Genes Dev. 13, 1079–1088 (1999).
Siegfried, K. R. et al. Members of the YABBY gene family are required to establish polarity in lateral organs. Development 126, 4117–4128 (1999).
Ogas, J., Kaufmann, S., Henderson, J. & Somerville, C. PICKLE is a CHD3 chromatin-remodeling factor that regulates the transition from embryonic to vegetative development in Arabidopsis. Proc. Natl Acad Sci. USA 96, 13839–13844 (1999).
Eshed, Y., Baum, S. F., Perea, J. V. & Bowman, J. L. Establishment of polarity in lateral organs of plants. Curr. Biol. 11, 1251–1260 (2001).
Telfer, A. & Poethig R. S. HASTY: a gene that regulates the timing of shoot maturation in Arabidopsis thaliana. Development 125, 1889–1898 (1998).
Vongs, A., Kakutani, T., Martienssen, R. A. & Richards, E. J. Arabidopsis thaliana DNA methylation mutants. Science 260, 1926–1928 (1993).This paper describes a brute force Southern-blot-based screen for mutants that affect DNA methylation at centromeric sequences.
Dehio, C. & Schell, J. Identification of plant genetic loci involved in a posttranscriptional mechanism for meiotically reversible transgene silencing. Proc. Natl Acad. Sci. USA 91, 5538–5542 (1994).
Kakutani, T., Munakata, K., Richards, E. J. & Hirochika, H. Meiotically and mitotically stable inheritance of DNA hypomethylation induced by ddm1 mutation of Arabidopsis thaliana. Genetics 151, 831–838 (1999).
Jeddeloh, J. A., Stokes, T. L. & Richards, E. J. Maintenance of genomic methylation requires a SWI2/SNF2-like protein. Nature Genet. 22, 94–97 (1999).
Bartee, L. & Bender, J. Two Arabidopsis methylation-deficiency mutations confer only partial effects on a methylated endogenous gene family. Nucleic Acids Res. 29, 2127–2134 (2001).
Bender, J. & Fink, G. R. Epigenetic control of an endogenous gene family is revealed by a novel blue fluorescent mutant of Arabidopsis. Cell 83, 725–734 (1995).
Lindroth, A. M. et al. Requirement of CHROMOMETHYLASE3 for maintenance of CpXpG methylation. Science 292, 2077–2080 (2001).Reports the use of a sensitized genetic background in a screen to investigate DNA methylation.
Jacobsen, S. E., Sakai, H., Finnegan, J., Cao, X. & Meyerowitz, E. M. Ectopic hypermethylation of flower-specific genes in Arabidopsis. Curr. Biol. 10, 179–186 (2000).
Jacobsen, S. E. & Meyerowitz, E. M. Hypermethylated SUPERMAN epigenetic alleles in Arabidopsis. Science 277, 1100–1103 (1997).
Millar, A. J., Straume, M., Chory, J., Chua, N.-H. & Kay, S. A. Circadian clock mutants in Arabidopsis identified by luciferase imaging. Science 267, 1161–1164 (1995).
Meier, C. et al. Gibberellin response mutants identified by luciferase imaging. Plant J. 25, 509–519 (2001).
Bowling, S. A. et al. A mutation in Arabidopsis that leads to constitutive expression of systemic acquired resistance. Plant Cell 6, 1845–1857 (1994).
Xiong, L. et al. The Arabidopsis LOS5/ABA3 locus encodes a molybdenum cofactor sulfurase and modulates cold stress- and osmotic stress-responsive gene expression. Plant Cell 13, 2063–2083 (2001).
Johnson, M. A., Perez-Amador, M. A., Lidder, P. & Green, P. J. Mutants of Arabidopsis defective in a sequence-specific mRNA degradation pathway. Proc. Natl Acad. Sci. USA 97, 13991–13996 (2000).An example that illustrates how precisely a background can be tailored for mutagenesis to screen for mutants that affect a specific biochemical pathway.
McClure, B. A. & Guilfoyle, T. J. Rapid redistribution of auxin-regulated RNAs during gravitropism. Science 243, 91–93 (1989).
Napoli, C., Lemieux, C. & Jorgensen, R. Introduction of a chimeric chalonce synthase gene into petunia results in reversible co-suppression of homologous genes in trans. Plant Cell 2, 279–289 (1990).
Matzke, M., Matzke, A. J. M. & Kooter, J. M. RNA: guiding gene silencing. Science 293, 1080–1083 (2001).A recent short review that summarizes the role of RNA in post-transcriptional and transcriptional gene silencing.
Dalmay, T., Hamilton, A., Rudd, S., Angell, S. & Baulcombe, D. C. An RNA-dependent RNA polymerase gene in Arabidopsis is required for posttranscriptional gene silencing mediated by a transgene but not by a virus. Cell 26, 543–553 (2000). | PubMed |
Mourrain, P. et al. Arabidopsis SGS2 and SGS3 genes are required for post-transcriptional gene silencing and natural virus resistance. Cell 101, 533–542 (2000).
Amedeo, P., Habu, Y., Afsar, K., Scheid, O. M. & Paszkowski, J. Disruption of the plant gene MOM releases transcriptional silencing of methylated genes. Nature 11, 203–206 (2000).
Cogoni, C. & Macino, G. Gene silencing in Neurospora crassa requires a protein homologous to RNA-dependent RNA polymerase. Nature 399, 166–169 (1999).
Smardon, A. et al. EGO-1 is related to RNA-directed RNA polymerase and functions in germ-line development and RNA interference in C. elegans. Curr. Biol. 10, 169–178 (2000).
Chen, Y.-C. S. & McCormick, S. sidecar pollen, an Arabidopsis male gametophytic mutant with aberrant cell divisions during pollen development. Development 122, 3243–3253 (1996).
Park, S. K., Howden, R. & Twell, D. The Arabidopsis thaliana gametophytic mutation gemini pollen1 disrupts microspore polarity, division asymmetry and pollen cell fate. Development 125, 3789–3799 (1998).
Johnson, S. A. & McCormick, S. Pollen germinates precociously in the anthers of raring-to-go, an Arabidopsis gametophytic mutant. Plant Physiol. 126, 685–695 (2001).
Grini, P. E. et al. Isolation of ethyl methanesulfonate-induced gametophytic mutants in Arabidopsis thaliana by a segregation distortion assay using the multimarker chromosome 1. Genetics 151, 849–863 (1999).
Howden, R. et al. Selection of T-DNA tagged male and female gametophytic mutants by segregation distortion in Arabidopsis. Genetics 149, 621–631 (1998).This paper gives a detailed description of the principle of segregation ratio distortion that is used to identify male and female gametophytic mutants.
Christensen, C. A., Subramanian, S. & Drews, G. N. Identification of gametophytic mutations affecting female gametophyte development in Arabidopsis. Dev. Biol. 202, 136–151 (1998).
Bonhomme, S. et al. T-DNA mediated disruption of essential gametophytic genes in Arabidopsis is unexpectedly rare and cannot be inferred from segregation distortion alone. Mol. Gen. Genet. 260, 444–452 (1998).
Desfeux, C., Clough, S. J. & Bent, A. F. Female reproductive tissues are the primary target of Agrobacterium-mediated transformation by the Arabidopsis floral-dip method. Plant Physiol. 123, 895–904 (2000).
Bechtold, N. et al. The maternal chromosome set is the target of the T-DNA in the in planta transformation of Arabidopsis thaliana. Genetics 155, 1875–1887 (2000).
Laibach, F. Ueber sommer- und winter-annuelle Rassen von Arabidopsis thaliana (L.) Heynh. Ein Beitrag zur Aetiologie der Blütenbildung. Beitr. Biol. Pflanz. 28, 173–210 (1951).
Kugler, I. Untersuchungen über das Keimverhalten einiger Rassen von Arabidopsis thaliana (L.) Heynh. Ein Beitrag zum Problem der Lichtkeimung. Beitr. Biol. Pflanz. 28, 211–243 (1951).
Bevan, M. & Murphy, G. The small, the large and the wild: the value of comparison in plant genomics. Trends Genet. 15, 211–214 (1999).
Mitchell-Olds, T. The molecular basis of quantitative genetic variations in natural populations. Trends Ecol. Evol. 10, 324–328 (1995).
Alonso-Blanco, C. & Koornneef, M. Naturally occuring variation in Arabidopsis: an underexploited resource for plant genetics. Trends Plant Sci. 5, 22–29 (2000).A comprehensive review on the potential of taking advantage of natural variation that occurs in the Arabidopsis gene pool.
Swarup, K. et al. Natural allelic variation identifies new genes in the Arabidopsis circadian system. Plant J. 20, 67–77 (1999).
Johanson, U. et al. Molecular analysis of FRIGIDA, a major determinant of natural variation in Arabidopsis flowering time. Science 290, 344–347 (2000).This paper illustrates how the analysis of naturally occurring variation can identify new functional alleles and reveal important information about the evolution and function of known genes.
Simpson, G. G., Gendall, A. R. & Dean, C. When to switch to flowering. Annu. Rev. Cell Dev. Biol. 99, 519–550 (1999).
Burn, J. E., Smyth, D. R., Peacock, W. J. & Dennis, E. S. Genes confering late flowering in Arabidopsis thaliana. Genetica 90, 145–157 (1993).
Sussman, M. R., Amasino, R. M., Young, J. C., Krysan, P. J. & Austin-Philipps, S. The Arabidopsis knockout facility at the University of Wisconsin–Madison. Plant Physiol. 124, 1465–1467 (2000).
Colbert, T. et al. High-throughput screening for induced point mutations. Plant Physiol. 126, 480–484 (2001).
Laibach, F. in Reports from the 1st International Conference on Arabidopsis Research (ed. Röbbelen, G.) 14–18 (University of Göttingen, Germany, 1965).
De Buck, S., Jacobs, A., Van Montagu, M. & Depicker, A. The DNA sequences of T-DNA junctions suggest that complex T-DNA loci are formed by a recombination process resembling T-DNA integration. Plant J. 20, 295–304 (1999).
Dooner, H. K. & Belachew, A. Transposition pattern of the maize element Ac from the bz-m2(Ac) allele. Genetics 122, 447–457 (1989).
Aarts, M. G. M., Dirkse, W. M., Stiekema, W. J. & Pereira, A. Transposon tagging of a male sterility gene in Arabidopsis. Nature 363, 715–717 (1993).
Bancroft, I. & Dean, C. Transpositon pattern of the maize element Ds in Arabidopsis thaliana. Genetics 134, 1221–1229 (1993).
Long, D. et al. The maize transposable element system Ac/Ds as a mutagen in Arabidopsis. Proc. Natl Acad. Sci. USA 90, 10370–10374 (1993).
Sundaresan, V. et al. Patterns of gene action in plant development revealed by enhancer trap and gene trap transposable elements. Genes Dev. 15, 1797–1810 (1995). | PubMed |
Müller, A. J. in Induction of Mutations and the Mutation Process (eds Veleminsky, J. & Gichner, T.) 46–52 (Czech. Acad. Sci., Prague, 1965).
Rédei, G. P. & Koncz, C. in Methods in Arabidopsis Research (eds Koncz, C., Chua, N.-H. & Schell, J.) 16–82 (World Scientific, Singapore, 1992).
Weigel, D. & Meyerowitz, E. M. The ABCs of floral homeotic genes. Cell 78, 203–209 (1994).
Acknowledgements
We thank M. Curtis and three anonymous reviewers for helpful comments on the manuscript, J.-P. Vielle-Calzada, J. Bowman and N. Huck for providing images, and J.-J. Pittet for help with original artwork. D.P. was supported by a Fellowship of the Roche Research Foundation and U.G. is a Searle Scholar. Support in U.G.'s lab comes from the Kanton of Zürich, the Swiss National Science Foundation, Novartis, and grants from the Bundesamt für Bildung und Wissenschaft as part of the APOTOOL and EXOTIC Projects in Framework V of the European Union.
Author information
Authors and Affiliations
Corresponding author
Related links
Glossary
- FLORAL DIPPING
-
A T-DNA transformation method in which plants are simply dipped into an Agrobacterium suspension.
- OVULE
-
The organ that develops into a seed after fertilization.
- ENHANCER DETECTION
-
A method originally developed in Drosophila that allows the identification of genes on the basis of their expression patterns. Engineered insertion elements carry a reporter gene construct under a minimal promoter that can respond to cis-acting regulatory elements near the insertion site.
- GENE TRAPPING
-
A modification of enhancer detection first used in mice. The minimal promoter driving the reporter gene is replaced by splice acceptor sites such that expression depends on integration within a gene.
- ACCESSION
-
A sample of a plant variety collected at a specific location and time. The terms ecotype, wild type and accession are not uniformly used in the Arabidopsis field and often cause confusion. The term accession is probably the most appropriate way to describe the Arabidopsis laboratory lines collected initially from the wild.
- GAMETOPHYTE
-
The plant generation that produces the gametes and usually has a reduced chromosome number.
- PHYTOHORMONE
-
A plant hormone that controls, or regulates, germination, growth, metabolism or other physiological functions.
- CARPEL
-
A leaf-like structure that encloses the ovules and is the defining character of flowering plants. In some species, multiple carpels might be present in a compound structure, called an ovary.
- PLACENTA
-
The tissue in the female reproductive organ of a plant that produces the ovules.
- NECTARY
-
The nectar-secreting gland in a flower.
- MERISTEMATIC CELL
-
Totipotent, undifferentiated cells in the meristems.
- CpNpG METHYLATION
-
In contrast to animals, in which 5-methylcytosine that is found in the sequence CpG is the main site of DNA methylation, both CpG and CpNpG (where N = A, T, C or G) are methylated in plants.
- STAMEN
-
The male, pollen-bearing organ of the flower.
- GYNOECIUM
-
The seed-bearing organ of the flower, which consists of the ovary, stigma (a sticky surface to which pollen grains attach and germinate) and style (which connects the stigma to the ovary).
- CO-SUPPRESSION
-
Silencing of an endogenous gene due to the presence of a homologous transgene or virus.
- EPIALLELES
-
Epigenetic variant that can confer a phenotype similar to a mutant variant of the same locus, but that has no associated changes at the DNA level.
- QUELLING
-
Specifically used to describe transgene-induced silencing in Neurospora crassa.
- RNA INTERFERENCE
-
(RNAi). The process by which double-stranded RNA specifically silences the expression of homologous genes through degradation of their cognate mRNA.
- SPOROPHYTE
-
The multicellular diploid form in plants that undergo alternation of generations. The sporophyte results from a union of haploid gametes and meiotically produces haploid spores that grow into the gametophyte generation.
- CHALAZA
-
The region of the ovule that surrounds the proximal pole of the female gametophyte and that gives rise to the integuments (protective coats) of the ovule.
- MICROPYLE
-
An opening at the distal pole of the ovule through which the pollen tube enters to effect double fertilization.
- EPISTATIC
-
When one gene masks the expression of another. If mutant a gives phenotype A and mutant b gives phenotype B, and if the double mutant ab gives phenotype A and not B, then gene a is epistatic to gene b.
- RECOMBINANT INBRED LINE
-
Homozygous plant line obtained after crossing two parental accessions and subsequent inbreeding over several generations.
- QUANTITATIVE TRAIT LOCUS
-
A genetic locus identified through the statistical analysis of complex traits (such as plant height or body weight). These traits are typically affected by more than one gene, and also by the environment.
- NEAR-ISOGENIC LINE
-
Homozygous plant lines obtained after crossing two parental accessions, then repeatedly backcrossing the progeny to one of the parents. Near-isogenic lines differ from the parental line only in a small genomic region.
- ECOTYPE
-
A subdivision of a species that survives as a distinct population through environmental selection and reproductive isolation.
Rights and permissions
About this article
Cite this article
Page, D., Grossniklaus, U. The art and design of genetic screens: Arabidopsis thaliana. Nat Rev Genet 3, 124–136 (2002). https://doi.org/10.1038/nrg730
Issue Date:
DOI: https://doi.org/10.1038/nrg730
This article is cited by
-
Mutant divergence in sunflower induced through gamma radiation
Journal of Crop Science and Biotechnology (2023)
-
Effectiveness and efficiency of Monosodium Glutamate as a potential mutagen inducing polyploidy in Drimia indica (Roxb.) Jessop
Genetic Resources and Crop Evolution (2022)
-
The genome-wide rate and spectrum of EMS-induced heritable mutations in the microcrustacean Daphnia: on the prospect of forward genetics
Heredity (2021)
-
Can genetic engineering-based methods for gene function identification be eclipsed by genome editing in plants? A comparison of methodologies
Molecular Genetics and Genomics (2021)
-
Development of male and female gametophytes and embryogenesis in the Arabidopsis thaliana
Biologia (2021)