Abstract
Current clinical therapies targeting receptor tyrosine kinases including focal adhesion kinase (FAK) have had limited or no effect on esophageal squamous cell carcinoma (ESCC). Unlike esophageal adenocarcinomas, ESCC acquire glucose in excess of their anabolic need. We recently reported that glucose-induced growth factor-independent proliferation requires the phosphorylation of FAKHis58. Here, we confirm His58 phosphorylation in FAK immunoprecipitates of glucose-stimulated, serum-starved ESCC cells using antibodies specific for 3-phosphohistidine and mass spectrometry. We also confirm a role for the histidine kinase, NME1, in glucose-induced FAKpoHis58 and ESCC cell proliferation, correlating with increased levels of NME1 in ESCC tumors versus normal esophageal tissues. Unbiased screening identified glucose-induced retinoblastoma transcriptional corepressor 1 (RB1) binding to FAK, mediated through a “LxCxE” RB1-binding motif in FAK’s FERM domain. Importantly, in the absence of growth factors, glucose increased FAK scaffolding of RB1 in the cytoplasm, correlating with increased ESCC G1→S phase transition. Our data strongly suggest that this glucose-mediated mitogenic pathway is novel and represents a unique targetable opportunity in ESCC.
This is a preview of subscription content, access via your institution
Access options
Subscribe to this journal
Receive 50 print issues and online access
$259.00 per year
only $5.18 per issue
Buy this article
- Purchase on Springer Link
- Instant access to full article PDF
Prices may be subject to local taxes which are calculated during checkout







Similar content being viewed by others
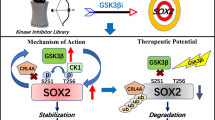
Data availability
All data generated or analyzed during this study are included in this published article and its supplementary information files.
References
Callahan R, Hurvitz S. Human epidermal growth factor receptor-2-positive breast cancer: current management of early, advanced, and recurrent disease. Curr Opin Obstet Gynecol. 2011;23:37–43.
Engelman JA, Janne PA. Mechanisms of acquired resistance to epidermal growth factor receptor tyrosine kinase inhibitors in non-small cell lung cancer. Clin Cancer Res. 2008;14:2895–9.
Kassouf W, Dinney CP, Brown G, McConkey DJ, Diehl AJ, Bar-Eli M, et al. Uncoupling between epidermal growth factor receptor and downstream signals defines resistance to the antiproliferative effect of Gefitinib in bladder cancer cells. Cancer Res. 2005;65:10524–35.
Sforza V, Martinelli E, Ciardiello F, Gambardella V, Napolitano S, Martini G, et al. Mechanisms of resistance to anti-epidermal growth factor receptor inhibitors in metastatic colorectal cancer. World J Gastroenterol. 2016;22:6345–61.
Yamaoka T, Ohba M, Ohmori T. Molecular-targeted therapies for epidermal growth factor receptor and its resistance mechanisms. Int J Mol Sci. 2017;18:2420 (1-22).
Zhu J, Thompson CB. Metabolic regulation of cell growth and proliferation. Nat Rev Mol Cell Biol. 2019;20:436–50.
Hotta M, Minamimoto R, Yamada K, Nohara K, Soma D, Nakajima K, et al. Efficacy of 4’-[methyl-11C] thiothymidine PET/CT before and after neoadjuvant therapy for predicting therapeutic responses in patients with esophageal cancer: a pilot study. EJNMMI Res. 2019;9:10.
Mantziari S, Pomoni A, Prior JO, Winiker M, Allemann P, Demartines N, et al. (18)F- FDG PET/CT-derived parameters predict clinical stage and prognosis of esophageal cancer. BMC Med Imaging. 2020;20:7.
Schreurs LM, Janssens AC, Groen H, Fockens P, van Dullemen HM, van Berge Henegouwen MI, et al. Value of EUS in determining curative resectability in reference to CT and FDG-PET: the optimal sequence in preoperative staging of esophageal cancer? Ann Surg Oncol. 2016;23:1021–8.
Lim CH, Park YJ, Shin M, Cho YS, Choi JY, Lee KH, et al. Tumor SUVs on 18F-FDG PET/CT and aggressive pathological features in esophageal squamous cell carcinoma. Clin Nucl Med. 2020;45:e128–e133.
Gillies RS, Middleton MR, Maynard ND, Bradley KM, Gleeson FV. Additional benefit of (1)(8)F-fluorodeoxyglucose integrated positron emission tomography/computed tomography in the staging of oesophageal cancer. Eur Radiol. 2011;21:274–80.
Furukawa T, Hamai Y, Hihara J, Emi M, Yamakita I, Ibuki Y, et al. Clinical significance of FDG-PET to predict pathologic tumor invasion and lymph node metastasis of superficial esophageal squamous cell carcinoma. Ann Surg Oncol. 2016;23:4086–92.
Yap WK, Chang YC, Hsieh CH, Chao YK, Chen CC, Shih MC, et al. Favorable versus unfavorable prognostic groups by post-chemoradiation FDG-PET imaging in node-positive esophageal squamous cell carcinoma patients treated with definitive chemoradiotherapy. Eur J Nucl Med Mol imaging. 2018;45:689–98.
Hamai Y, Emi M, Ibuki Y, Murakami Y, Nishibuchi I, Nagata Y, et al. Predictions of pathological features and recurrence based on FDG-PET findings of esophageal squamous cell carcinoma after trimodal therapy. Ann surgical Oncol. 2020;27:4422–30.
Goodwin J, Neugent ML, Lee SY, Choe JH, Choi H, Jenkins DMR, et al. The distinct metabolic phenotype of lung squamous cell carcinoma defines selective vulnerability to glycolytic inhibition. Nat Commun. 2017;8:15503.
Vander Heiden MG, Locasale JW, Swanson KD, Sharfi H, Heffron GJ, Amador-Noguez D, et al. Evidence for an alternative glycolytic pathway in rapidly proliferating cells. Science. 2010;329:1492–9.
He X, Cheng X, Ding J, Xiong M, Chen B, Cao G. Hyperglycemia induces miR-26-5p down-regulation to overexpress PFKFB3 and accelerate epithelial-mesenchymal transition in gastric cancer. Bioengineered. 2022;13:2902–17.
Ghosh A, Shieh JJ, Pan CJ, Chou JY. Histidine 167 is the phosphate acceptor in glucose-6-phosphatase-beta forming a phosphohistidine enzyme intermediate during catalysis. J Biol Chem. 2004;279:12479–83.
Kalagiri R, Hunter T. The many ways that nature has exploited the unusual structural and chemical properties of phosphohistidine for use in proteins. Biochemical J. 2021;478:3575–96.
Adam K, Lesperance J, Hunter T, Zage PE. The potential functional roles of NME1 histidine kinase activity in neuroblastoma pathogenesis. Int J Mol Sci. 2020;21:3319 (1-19).
Hindupur SK, Colombi M, Fuhs SR, Matter MS, Guri Y, Adam K, et al. The protein histidine phosphatase LHPP is a tumour suppressor. Nature. 2018;555:678–82.
Zhang J, Gelman IH, Katsuta E, Liang Y, Wang X, Li J, et al. Glucose drives growth factor-independent esophageal cancer proliferation via phosphohistidine-focal adhesion kinase signaling. Cell Mol Gastroenterol Hepatol. 2019;8:37–60.
Zhang J, Gao Q, Zhou Y, Dier U, Hempel N, Hochwald SN. Focal adhesion kinase-promoted tumor glucose metabolism is associated with a shift of mitochondrial respiration to glycolysis. Oncogene. 2016;35:1926–42.
Lee BY, Timpson P, Horvath LG, Daly RJ. FAK signaling in human cancer as a target for therapeutics. Pharmacol therapeutics. 2015;146:132–49.
Schober M, Fuchs E. Tumor-initiating stem cells of squamous cell carcinomas and their control by TGF-beta and integrin/focal adhesion kinase (FAK) signaling. Proc Natl Acad Sci USA. 2011;108:10544–9.
Sieg DJ, Hauck CR, Ilic D, Klingbeil CK, Schaefer E, Damsky CH, et al. FAK integrates growth-factor and integrin signals to promote cell migration. Nat Cell Biol. 2000;2:249–56.
Kim HY, Ahn BY, Cho Y. Structural basis for the inactivation of retinoblastoma tumor suppressor by SV40 large T antigen. EMBO J. 2001;20:295–304.
Lee JO, Russo AA, Pavletich NP. Structure of the retinoblastoma tumour-suppressor pocket domain bound to a peptide from HPV E7. Nature. 1998;391:859–65.
Palopoli N, Gonzalez Foutel NS, Gibson TJ, Chemes LB. Short linear motif core and flanking regions modulate retinoblastoma protein binding affinity and specificity. Protein Eng Des Sel. 2018;31:69–77.
Zhang C, Stockwell SR, Elbanna M, Ketteler R, Freeman J, Al-Lazikani B, et al. Signalling involving MET and FAK supports cell division independent of the activity of the cell cycle-regulating CDK4/6 kinases. Oncogene. 2019;38:5905–20.
Esteban-Villarrubia J, Soto-Castillo JJ, Pozas J, San Roman-Gil M, Orejana-Martin I, Torres-Jimenez J, et al. Tyrosine kinase receptors in oncology. Int J Mol Sci. 2020;21:8529 (1-48).
Mushtaq U, Bashir M, Nabi S, Khanday FA. Epidermal growth factor receptor and integrins meet redox signaling through P66shc and Rac1. Cytokine. 2021;146:155625.
Phan P, Saikia BB, Sonnaila S, Agrawal S, Alraawi Z, Kumar TKS, et al. The saga of endocrine FGFs. Cells. 2021;10:2418 (1-38).
Postel EH, Ferrone CA. Nucleoside diphosphate kinase enzyme activity of NM23-H2/PuF is not required for its DNA binding and in vitro transcriptional functions. J Biol Chem. 1994;269:8627–30.
Zhu J, Tseng YH, Kantor JD, Rhodes CJ, Zetter BR, Moyers JS, et al. Interaction of the Ras-related protein associated with diabetes rad and the putative tumor metastasis suppressor NM23 provides a novel mechanism of GTPase regulation. Proc Natl Acad Sci USA. 1999;96:14911–8.
Su H, Hu N, Yang HH, Wang C, Takikita M, Wang QH, et al. Global gene expression profiling and validation in esophageal squamous cell carcinoma and its association with clinical phenotypes. Clin Cancer Res. 2011;17:2955–66.
Hu N, Clifford RJ, Yang HH, Wang C, Goldstein AM, Ding T, et al. Genome wide analysis of DNA copy number neutral loss of heterozygosity (CNNLOH) and its relation to gene expression in esophageal squamous cell carcinoma. BMC Genomics. 2010;11:576.
Lapek JD Jr., Tombline G, Friedman AE. Mass spectrometry detection of histidine phosphorylation on NM23-H1. J Proteome Res. 2011;10:751–5.
Cavalier MC, Kim SG, Neau D, Lee YH. Molecular basis of the fructose-2,6-bisphosphatase reaction of PFKFB3: transition state and the C-terminal function. Proteins. 2012;80:1143–53.
Wieland T, Attwood PV. Alterations in reversible protein histidine phosphorylation as intracellular signals in cardiovascular disease. Front Pharmacol. 2015;6:173.
Chellappan S, Kraus VB, Kroger B, Munger K, Howley PM, Phelps WC, et al. Adenovirus E1A, simian virus 40 tumor antigen, and human papillomavirus E7 protein share the capacity to disrupt the interaction between transcription factor E2F and the retinoblastoma gene product. Proc Natl Acad Sci USA. 1992;89:4549–53.
Zhang W, Lei C, Fan J, Wang J. miR-18a promotes cell proliferation of esophageal squamous cell carcinoma cells by increasing cylin D1 via regulating PTEN-PI3K-AKT-mTOR signaling axis. Biochem Biophys Res Commun. 2016;477:144–9.
Beca F, Pereira M, Cameselle-Teijeiro JF, Martins D, Schmitt F. Altered PPP2R2A and Cyclin D1 expression defines a subgroup of aggressive luminal-like breast cancer. BMC Cancer. 2015;15:285.
Geng Y, Yu Q, Sicinska E, Das M, Bronson RT, Sicinski P. Deletion of the p27Kip1 gene restores normal development in cyclin D1-deficient mice. Proc Natl Acad Sci USA. 2001;98:194–9.
Ikeguchi M, Sakatani T, Ueta T, Kaibara N. Cyclin D1 expression and retinoblastoma gene protein (pRB) expression in esophageal squamous cell carcinoma. J Cancer Res Clin Oncol. 2001;127:531–6.
Ma WW. Development of focal adhesion kinase inhibitors in cancer therapy. Anti-Cancer Agents Med Chem. 2011;11:638–42.
Hochwald JS, Zhang J. Glucose oncometabolism of esophageal cancer. Anti-Cancer Agents Med Chem. 2017;17:385–94.
Kim SY, Kawaguchi T, Yan L, Young J, Qi Q, Takabe K. Clinical relevance of microRNA expressions in breast cancer validated using the cancer genome atlas (TCGA). Ann Surg Oncol. 2017;24:2943–9.
Kee JM, Oslund RC, Perlman DH, Muir TW. A pan-specific antibody for direct detection of protein histidine phosphorylation. Nat Chem Biol. 2013;9:416–21.
Kim SC, Chen Y, Mirza S, Xu Y, Lee J, Liu P, et al. A clean, more efficient method for in-solution digestion of protein mixtures without detergent or urea. J Proteome Res. 2006;5:3446–52.
Zu XL, Besant PG, Imhof A, Attwood PV. Mass spectrometric analysis of protein histidine phosphorylation. Amino Acids. 2007;32:347–57.
An B, Zhang M, Johnson RW, Qu J. Surfactant-aided precipitation/on-pellet-digestion (SOD) procedure provides robust and rapid sample preparation for reproducible, accurate and sensitive LC/MS quantification of therapeutic protein in plasma and tissues. Anal Chem. 2015;87:4023–9.
Oslund RC, Kee JM, Couvillon AD, Bhatia VN, Perlman DH, Muir TW. A phosphohistidine proteomics strategy based on elucidation of a unique gas-phase phosphopeptide fragmentation mechanism. J Am Chem Soc. 2014;136:12899–911.
Shen X, Shen S, Li J, Hu Q, Nie L, Tu C, et al. An IonStar experimental strategy for MS1 Ion current-based quantification using ultrahigh-field orbitrap: reproducible, in-depth, and accurate protein measurement in large cohorts. J Proteome Res. 2017;16:2445–56.
Wang X, Niu J, Li J, Shen X, Shen S, Straubinger RM, et al. Temporal effects of combined birinapant and paclitaxel on pancreatic cancer cells investigated via large-scale, ion-current-based quantitative proteomics (IonStar). Mol Cell Proteom. 2018;17:655–71.
Funding
Roswell Park Comprehensive Cancer Center Alliance Foundation Grant (SH and JZ).
Author information
Authors and Affiliations
Contributions
JZ: Designed and performed experiments and prepared the manuscript. IG: TCGA database search and analysis, study design, data interpretation and critical revision of manuscript. JQ: Designed MS study and interpreted MS results. SH: Study design, data analysis/interpretation and manuscript preparation.
Corresponding author
Ethics declarations
Competing interests
The authors declare no competing interests.
Additional information
Publisher’s note Springer Nature remains neutral with regard to jurisdictional claims in published maps and institutional affiliations.
Supplementary information
Rights and permissions
Springer Nature or its licensor (e.g. a society or other partner) holds exclusive rights to this article under a publishing agreement with the author(s) or other rightsholder(s); author self-archiving of the accepted manuscript version of this article is solely governed by the terms of such publishing agreement and applicable law.
About this article
Cite this article
Zhang, J., Gelman, I.H., Qu, J. et al. Phosphohistidine signaling promotes FAK-RB1 interaction and growth factor-independent proliferation of esophageal squamous cell carcinoma. Oncogene 42, 449–460 (2023). https://doi.org/10.1038/s41388-022-02568-4
Received:
Revised:
Accepted:
Published:
Issue Date:
DOI: https://doi.org/10.1038/s41388-022-02568-4