Abstract
Immaturity of the epidermal barrier in the preterm infant may have serious clinical consequences. However, regardless of the degree of prematurity, the barrier rapidly matures such that by 2 wk all infants display a competent barrier. To determine whether the change from an aqueous (intrauterine) to a xeric environment might be the stimulus for this accelerated maturation, we examined the effects of air exposure on cutaneous barrier formation in vitro. Skin explants from d 17 fetal rats were incubated either submerged or at the air-medium interface. As previously reported, a competent barrier formed under submerged conditions after 3-4 d, precisely mirroring the time course of maturation in utero. In contrast, barrier maturation was accelerated in air-exposed explants, with functional, histologic, and structural markers of barrier formation observed after only 2 d of incubation. A water-impermeable membrane blocked the acceleration of barrier formation, resulting in a developmental time course comparable to that for submerged explants. In contrast, a water vapor-permeable membrane did not block the acceleration. Glucocorticoids and thyroid hormone, which accelerate barrier formation in utero or in vitro under submerged conditions, did not further accelerate barrier formation in the air-exposed model. These data indicate that: 1) air exposure accelerates barrier ontogenesis, suggesting that water flux may be an important signal for the accelerated barrier formation that occurs in premature infants; and 2) factors which accelerate barrier formation in utero may not further accelerate barrier formation in neonates.
Similar content being viewed by others
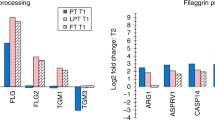
Main
The development of the epidermal permeability barrier, located in the uppermost layers of the epidermis, the SC(1), is completed in utero during the last trimester. Infants born near term(after 32 wk EGA) have a well developed epidermis, with a prominent SC, and a competent barrier both to TEWL and to transcutaneous absorption of water-soluble xenobiotics(2, 3). In contrast, in more premature infants, barrier deficiency with increased TEWL rates is present; the severity of the barrier deficiency at birth parallels the degree of prematurity(2–6). The consequences of barrier immaturity in the premature infant include increased heat loss and fluid and electrolyte imbalances due to high rates of TEWL and increased susceptibility to bacterial and fungal infection, as well as toxicity from epicutaneously applied xenobiotics due to high skin permeability(4, 5, 7, 8).
The development of the epidermal barrier in the human preterm infant is markedly accelerated by still largely undefined postnatal influences;i.e. by 2 wk of life, the epidermis is morphologically and functionally similar to that of term infants, regardless of the degree of prematurity(2–6). Whether the stimulus to rapid epidermal maturation in the preterm infant is the change from a fluid (intrauterine) to a gaseous (ambient) environment, and whether these changes are mediated purely by local factors or whether they occur in response to systemic signals is not known.
The fetal rat has proved a useful model for the study of barrier ontogenesis. Epidermal stratification begins by d 17 EGA, and exocytosis of lamellar body contents into the stratum granulosum-SC interface is evident by d 19(9, 10). A competent barrier to water loss is present on d 21 (term = 22 d), concurrent with the transformation of lamellar body-derived material into mature lamellar unit structures in the extracellular spaces of the SC(9). The timetable for skin barrier ontogenesis is identical to that observed for development of the lung surfactant system in the rat(9, 11, 12), and, as in lung, glucocorticoids accelerate maturation of the epidermal lamellar body system in utero(13, 14). Recently, we have developed anin vitro model of barrier ontogenesis in which skin explants taken from d 17 fetal rats, and submerged in a hormone-free medium, develop competent barriers to water loss, precisely mirroring the in utero time course(10). Moreover, addition of glucocorticoids or thyroid hormone to the medium accelerates barrier ontogenesis under these conditions(10).
Although this submerged in vitro model mimics the aqueous environment of in utero development, it does not simulate the xeric environment surrounding the preterm infant. The aim of the present study was to determine whether air exposure affects the rate of barrier formation in ourin vitro model, and to ascertain whether hormones that accelerate barrier development in an aqueous environment also accelerate barrier formation under these conditions.
METHODS
Materials. Sprague-Dawley timed pregnant rats (plug date = d 0) were obtained from Simonsen Laboratories (Gilroy, CA). COL-well tissue culture inserts (pore size 3 μm) were from Costar (Cambridge, MA) and M199 medium were obtained from Life Technologies, Inc. (Grand Isle, NY). Dexamethasone, triiodothyronine, EGF, and TGF-α were from Sigma Chemical Co. (St. Louis, MO). IGF-1 was obtained from Boehringer Mannheim (Indianapolis, IN).
In vitro submerged model. Fetal rats were delivered by cesarean section on gestational d 17 after anesthesia of the mothers with isofluorane as previously described(10). Flank skin was excised from d 17 EGA fetal rats and full-thickness skin explants were placed dermis-side down on collagen membranes, inserted into culture wells, and submerged in 4 mL of M199 media, as described previously(10).
in vitro air-exposed model. Skin explants were prepared and placed on membranes as described above. Polyester biopsy sponges (Histoprep, Fisher) were placed into culture wells, and 4 mL of M199 medium were added. Membranes and explants were then placed on the sponges, so that the explants were incubated at the air-medium interface and received media only from below.
Dexamethasone or triiodothyronine were added to the medium in ≤0.05% vehicle (ethanol or DMSO, respectively) in concentrations of 1-100 nM. The growth factors EGF, IGF-1, or TGF-α, were added in concentrations ranging from 1 to 100 ng/mL.
Water loss was inhibited by placing a piece of water-impermeable plastic(Saran wrap) on the epidermal surface of the explant. Omiderm wound dressing(Omikron Scientific Ltd.) was used as a water-permeable membrane.
Barrier function. Water loss in explants was measured gravimetrically. Explants were removed from the culture wells, all surfaces(i.e. cut surfaces and dermal surface) except the epidermis were sealed with petrolatum, and explants were then weighed hourly using a Cahn balance (sensitivity 0.001 mg), as previously described(10). Results are reported as milligrams of water lost/h/mm2.
Light microscopy and histochemistry. Samples were frozen and sectioned as described previously(10). Ten-microgram sections were stained with hematoxylin, and 5-μm sections were stained with Nile red, as previously described(10). Samples for Feulgen-staining were fixed in buffered formalin, paraffin-embedded, and sectioned (5 μm).
Histometric measurements. Hematoxylin-stained sections were examined using a microscope equipped with a micometer scale. Five points were selected at random on each section, and epidermal thickness was measured in micrometers as the distance from the bottom of the basal layer to the top of the stratum granulosum.
Electron microscopy. Explants were minced to ≤1 mm3 pieces and fixed overnight at 4°C in 0.1 M sodium cacodylate buffer containing 2% glutaraldehyde, 2% paraformaldehyde, and 0.06% CaCl2 (pH 7.3). Postfixation, dehydration, and embedding were performed as previously described(10). Samples were examined using a Zeiss 10A electron microscope at 60 kV.
Statistics. Statistical analysis was performed using thet test.
RESULTS
Effects of air exposure on barrier formation in vitro. We first compared the kinetics of barrier formation in submerged explantsversus those incubated at an air-medium interface (Fig. 1). As we have described previously, epidermal barrier development in skin explants taken from d 17 fetal rats and submerged in hormone- and serum-free medium parallels the developmental timetablein utero(10). Thus, a competent barrier to water loss is present in submerged explants after 3-4 d of incubation, corresponding to 20-21-d EGA. In contrast, in air-exposed explants, barrier development is accelerated, with a competent barrier present after only 2 d of incubation (Fig. 1). Three separate experiments were performed, and a typical experiment is shown in Figure 1. These studies show that barrier development is accelerated under air-exposed conditions in vitro.
Barrier development in fetal skin in vitro is accelerated by air exposure. Explants from d 17 rats were incubated either submerged (closed squares) or at the air-medium interface(open squares). Barrier function was measured gravimetrically at 24-h intervals. Barrier function after 3-4 d under submerged conditions approaches that observed in skin from term pups after intrauterine gestation(dotted line). In contrast, air-exposed explants exhibit more competent barriers than submerged explants after 1, 2, or 3 d in culture and approached the competence of term pups on d 2 of incubation. Similar results were seen in two additional experiments. Data are expressed as the mean± SEM, n = 4. *p < 0.005, **p < 0.01, compared with controls.
Histologic effects of air exposure. To determine the basis for these functional changes, we next assessed the histologic changes that underlie this functional acceleration. Frozen sections of explants, incubated for 1 or 2 d under submerged or air-exposed conditions, were stained with either hematoxylin (Fig. 2) or Nile red (Fig. 3). Six to eight explants of each condition were examined. Explants incubated at the air-medium interface for 1 d(Fig. 2B, L + 1) exhibit an apparent increase in epidermal thickness, in comparison with explants incubated submerged for the same time period (Fig. 2A, S + 1). After 2 d of incubation, a distinct SC is not yet present in submerged explants, although increased epidermal thickness is now evident (Fig. 2C, S+ 2). In contrast, explants air-exposed for 2 d exhibit a distinct granular layer and SC (Fig. 2D, L + 2), a morphology similar to that previously described in submerged explants incubated 4 d(10), or in epidermis from d 21 pups in utero(9).
Effects of air exposure on histology of fetal epidermisin vitro. Skin explants from d 17 fetal rats were incubated for 1 or 2 d either submerged (S) or air-exposed (L) as described in “Methods.” Frozen sections (10 μm) were stained with hematoxylin. Explants incubated for 1 d at the air-medium interface(panel B, L + 1) exhibit increased epidermal thickness compared with submerged explants (panel A, S + 1). After 2 d of incubation, submerged explants lack a well defined SC (panel C, S + 2). In contrast, explants incubated air-exposed for 2 d (panel D, L + 2) exhibit a distinct granular layer and SC (arrows). Bars, 15μm.
Effects of air exposure on lipid histochemistry of fetal epidermis in vitro. Explants were incubated for 2 d either submerged or air-exposed. Frozen sections (5 μm) were stained with Nile red. Submerged explants (S + 2) showed a cytosolic pattern of red-orange fluorescence indicating a predominance of polar lipid in the outermost cell layers (arrowheads). Air-exposed explants (L + 2) exhibited yellow-gold fluorescence indicative of neutral lipid in a membrane pattern throughout the multilayered SC (arrows). E, epidermis;D, dermis. Bars, 10 μm.
To determine whether accelerated barrier maturation reflects enhanced lipid deposition in the epidermis, we next examined frozen sections stained with the hydrophobic lipid probe, Nile red. Explants incubated submerged for 2 d display a predominantly cytosolic pattern of orange-red staining indicative of polar lipid (Fig. 3, S + 2), as described previously(10). In contrast, explants incubated at the air-medium interface for 2 d exhibit intense yellow-gold staining, indicative of neutral lipid, distributed in a mature, membrane pattern throughout the SC(Fig. 3, L + 2, arrows). These results demonstrate that acceleration of the functional maturation of the barrier is accompanied by increased epidermal thickness, formation of a multilayered SC, and membrane pattern deposition of neutral lipids.
Ultrastructural effects of air exposure. We next examined the upper epidermis in explants incubated either submerged or air-exposed for 2 d by electron microscopy using ruthenium tetroxide postfixation to stain the intercellular lipid lamellae. As reported previously(10), secreted lamellar body-derived material that has not yet been reorganized into mature lamellar structures predominates the extracellular spaces of a single-layered SC in explants submerged in hormone- and serum-free media for 2 d (Fig. 4A). In contrast, the interstices of a multilayered SC are filled with multiple arrays of mature lamellar membrane unit structures in explants incubated at the air-medium interface for 2 d (Fig. 4B and inset). These data demonstrate that the formation of mature intercellular membrane structures in the SC membranes underlie the functional acceleration of barrier formation produced by exposure to air.
Effects of air exposure on epidermal ultrastructurein vitro. Representative electron micrographs of upper epidermis in fetal skin explants. Explants incubated 2 d under submerged conditions(panel A) show lamellar body-derived material in the extracellular spaces of the single cell layered SC, which has not been reorganized into mature lamellar unit structures. In explants incubated air-exposed for 2 d(panel B) the interstices of the multilayered SC (SC1-SC4) are filled with mature lamellar bilayers. The inset shows a higher magnification of a lamellar unit structure (arrowheads). P, periderm; SG, stratum granulosum. Bars, 0.5 μm.
Effects of inhibition of water loss on air-exposed barrier formation. To determine whether the acceleration of barrier maturation under air-exposed conditions is mediated by changes in water flux across the epidermis, either a water vapor-impermeable or a water vapor-permeable membrane was placed over the surface of the explants during incubation. All experiments were performed at least twice. The application of a water-permeable membrane had no effect on barrier formation in air-exposed explants; i.e. accelerated formation of a competent barrier was still observed. In contrast, occlusion with a water-impermeable membrane blocked the acceleration in barrier formation; barrier competence in these cultures mirrored that observed in submerged explants (Fig. 5). Morphologic examination of membrane-treated explants revealed no evidence of necrosis or toxicity (data not shown). Moreover, removal of the water-impermeable membrane after 2 d of incubation, followed by further incubation for 2 d at the air-medium interface, also results in development of a competent barrier and normal morphology (data not shown). Furthermore, 4 d of occlusion with a water-impermeable membrane results in formation of a competent barrier, as seen in submerged cultures (data not shown). Thus, occlusion does not completely inhibit barrier formation, it simply prevents the acceleration that occurs with air exposure. These data suggest that changes in water flux through the epidermis may signal the acceleration of barrier formation.
Air-exposed barrier development is inhibited by occlusion with a water-impermeable membrane. Explants were incubated air-exposed for 2 d with either no membrane, or covered with a water-permeable or water-impermeable membrane. Barrier function was assessed gravimetrically as described in “Methods.” Note that barrier function in explants occluded with a water-impermeable membrane is similar to that measured in explants incubated under submerged conditions for 2 d (dotted line). Results represent mean ± SEM, n = 4. *p < 0.005 compared with air-exposed controls. Similar results were obtained in two separate experiments.
Effects of air exposure and occlusion on epidermal thickness. Because the histologic sections suggested that epidermal hyperplasia occurs in fetal skin grown at an air-medium interface, we next measured epidermal thickness in hematoxylin-stained frozen sections of explants incubated either submerged or at an air-medium interface for 1 or 2 d. As shown in Table 1, air exposure results in significantly increased epidermal thickness in comparison with submerged controls (53% over controls after 1 d, 86% after 2 d). To determine whether the increase in epidermal thickness resulting from air exposure could be attributer to stimulation of epidermal proliferation, we examined samples stained with a DNA-specific stain(Feulgen). Feulgen-stained nuclei were markedly increased throughout the basal layer in 2-day air-exposed versus submerged explants (data not shown). Taken together, these data imply that increased epidermal proliferation occurs in response to air exposure.
Epidermal thickness was also measured in air-exposed explants after 2 d of occlusion with a water-permeable or water-impermeable membrane. Occlusion with a permeable membrane resulted in a significant increase in the thickness of the epidermis compared with control explants submerged for 2 d. In contrast, occlusion with an impermeable membrane blocked the increase, so that epidermal thickness was not significantly different from submerged controls(Table 1). These data indicate that increased water movement through the epidermis may signal epidermal proliferation.
Effects of hormones and growth factors on air-exposed barrier formation. We have shown previously that glucocorticoids or thyroid hormone, when added to the incubation medium for 2 d, accelerates the formation of a competent barrier in submerged skin explants(10). To determine whether factors which accelerate development under submerged conditions might further accelerate development in air-exposed conditions, we next examined the effects of dexamethasone or thyroid hormone on air-exposed barrier formation. Neither hormone, in varying concentrations, added to the incubation medium or added topically to air-exposed explants, further accelerated barrier formation or morphology(data not shown). Furthermore, the addition of EGF, IGF-1, or TGF-α to the media did not affect barrier formation in air-exposed explants (data not shown).
DISCUSSION
Most of the insensible water loss in the preterm infant is attributed to evaporative loss from the skin surface and losses of up to 190 mL/kg/d are encountered in the very premature infant(8); however, these excessive rates of water loss are only temporary. By 1-2 wk of life, even the most premature infants generate a SC with barrier properties indistinguishable from term infants(2–6). The effects of duration of gestation, and of postnatal age, on the functional and morphologic development of the epidermis have been well documented(2–6). However, the mechanism by which barrier formation so rapidly accelerates in the very premature neonate is unknown.
We previously described epidermal barrier development in the fetal ratin utero(9). A measurable barrier is not present in pups of gestational age d 19, when secreted lamellar body-derived contents have not yet been reorganized into lamellar bilayer unit structures in the extracellular spaces of the SC(9). By d 21, coincident with the formation of mature lamellar bilayers throughout the SC, all pups display competent barriers(9). Because premature rodent pups do not survive, air-exposed barrier formation cannot be studied in this in vivo model. Hence, we have used an in vitro model of fetal barrier formation, in which competent barriers form in d 17 fetal rat skin explants after 4 d of submersion in hormone- and serum-free media(10). Functional, histochemical, and structural markers of maturation in this system precisely mirror the in utero time course of barrier development(9, 10).
In the present study we have examined barrier development in skin explants incubated under air-exposed conditions. When explants taken from d 17 fetal rats are incubated at the air-medium interface, designed to correspond to the environmental interface at which the epidermal barrier develops in the preterm neonate, a competent barrier develops after only 2 d of incubation. This acceleration of barrier development coincides with the formation of a multilayered SC (cf. Fig. 1), the appearance of a mature pattern of neutral lipid staining in the SC (cf.Fig. 2), and, finally, with the accelerated formation of continuous, lamellar unit structures throughout the extracellular spaces of the SC (cf. Fig. 3). Thus, air-exposed explants exhibit a significantly accelerated timetable for barrier development when compared either with submerged explants or in utero maturation. This compressed timetable mimics the acceleration of barrier ontogenesis, which occurs in the human infant after very premature birth.
Our observation of accelerated barrier maturation in fetal skin explants in response to air exposure is in agreement with earlier studies demonstrating enhanced epidermal differentiation when either adult or neonatal human keratinocytes are incubated at the air-medium interface(15–17). However, the formation of lamellar bodies and deposition of intercellular SC membranes are reduced in quantity in comparison with epidermis in vivo(16). Thus, even in the most “optimized” systems, formation of a fully competent barrier is not achieved(15–17). In contrast, fetal skin cultured at the air-medium interface undergoes an accelerated maturation of barrier competence, the final product of which is indistinguishable from skinin vivo.
Systemic factors have been suggested as mediators of the acceleration of epidermal development after premature birth. EGF accelerates morphogenesis(precocious eyelid opening, tooth eruption) when injected into neonatal mice(reviewed inRef. 18). However, no change in the urinary excretion of EGF occurs in preterm infants in the early neonatal period(19). Moreover, exogenous EGF does not accelerate barrier development in vitro(10). Cortisol levels increase during late gestation and also rise in response to the stress of premature labor(20). Furthermore, we have shown previously that glucocorticoids accelerate barrier formation in the ratin utero and in vitro(10, 14). However, glucocorticoids and other hormones do not appear to be required for barrier formation, because maturation occurs on time in hormone-free media in vitro(14). The present study extends these observations to suggest that local skin-derived factors, rather than systemic influences, mediate the acceleration of barrier formation in the preterm neonate, because barrier formation accelerates after exposure to air in the absence of exogenous hormones or growth factors, and addition of hormones and growth factors causes no further maturational acceleration. Nonetheless, these studies do not exclude a mediatory role for locally produced growth factors, and whether the endogenous levels of various growth factors change with exposure to air remains to be determined. This study also demonstrates that factors that stimulate barrier formation in utero and under submerged conditions may not further stimulate barrier development under air-exposed conditions.
Our studies using occlusive membranes also address the potential signal for accelerated barrier ontogenesis with air exposure. Water flux appears to be an important signal for the acceleration of barrier formation, because occlusion with a water-impermeable but not a water-permeable membrane results in a timetable of barrier development in air-exposed explants similar to that of submerged explants. These reports have important clinical implications for the use of emollients and dressings with different permeabilities, aimed toward decreasing water and caloric loss in the human premature infant. Our studies suggest that excessively occlusive dressings could inhibit the normal acceleration of barrier formation in the preterm neonate. Mancini et al.(21) have demonstrated that semipermeable membrane dressings do not impede formation of a barrier in preterm infants. Moreover, the semipermeable nature of the membrane appears to be of further benefit, because a several thousand-fold increase in microbial flora has been demonstrated at skin sites treated with vapor-impermeable membranes(21).
Insights into the mechanisms whereby changes in water flux stimulate barrier ontogenesis may be gained by a model of barrier disruption in the adult mouse. Barrier deficiency, produced by removal of the SC by tape-stripping or removal of SC lipids through acetone wipes, initiates the following sequence of events in the upper epidermis: 1) immediate secretion of lamellar bodies, 2) increased synthesis of barrier lipids, 3) formation of new lamellar bodies and further secretion, and 4) processing of secreted material into mature lamellar unit structures and restoration of barrier competence(22). Each step in this repair response is prevented by occlusion with a water-impermeable membrane immediately after barrier abrogation, but not by application of a vapor-permeable membrane(22). Thus, changes in water flux are critical in initiating the sequence of barrier repair in the mature animal. However, water transit across the SC per se is not the signal for barrier recovery, because immersion of damaged skin in solutions of differing osmolarities does not prevent barrier repair(23). Normally, calcium, and perhaps other ions, becomes more concentrated in the extracellular milieu surrounding the upper nucleated epidermal cells(23, 24). The mechanisms whereby this gradient is established is not known. It appears that loss of the SC barrier with increased transepidermal water flux leads to passive loss of ions, particularly calcium and potassium, from the extracellular environment of the upper epidermis(24). A decrease in cation concentrations is then a signal for lamellar body secretion and barrier repair in the mature rodent(24, 25). Whether changes in the concentration of calcium or other ions in the external milieu of the uppermost epidermal cell layers, as a result of the increased water loss after air exposure, signals the acceleration of barrier formation in the preterm infant remains to be determined.
In addition to signals leading to barrier repair, barrier disruption in the adult hairless mouse results in a burst in epidermal DNA synthesis(26). This augmented synthesis is largely suppressed by occlusion with impermeable dressings(26). The increase in epidermal thickness which accompanies accelerated barrier formation in air-exposed explants in the present study suggests a similar stimulation of DNA synthesis may be imposed by xeric stress to the premature infant. In the current model, occlusion with an impermeable membrane inhibits the increase in thickness induced by air exposure. These data suggest that epidermal proliferation is stimulated by the change in water flux in the uppermost epidermal layers.
In summary, we have developed an air-exposed model of barrier ontogenesis which displays an accelerated timetable of development when compared with barrier formation under submerged conditions or to in utero maturation, similar to the accelerated barrier maturation observed in premature neonates. Changes in water flux appear to be essential for this acceleration. Glucocorticoids or thyroid hormone, which accelerates barrier ontogenesis in the submerged system, as well as a variety of growth factors, do not further accelerate formation in the air-exposed model. Because consequences of barrier immaturity in the preterm infant, such as increased absorption of xenobiotics and systemic infection by cutaneous microflora, contribute to the morbidity and mortality of prematurity, further understanding of the manner in which barrier formation in the preterm neonate can be stimulated should be of considerable clinical interest.
Abbreviations
- SC:
-
stratum corneum
- TEWL:
-
transepidermal water loss
- EGA:
-
estimated gestational age
- EGF:
-
epidermal growth factor
- IGF:
-
insulin-like growth factor
- TGF:
-
transforming growth factor
References
Elias PM, Menon GM 1991 Structural and lipid biochemical correlates of the epidermal permeability barrier. Adv Lipid Res 33: 301–313
Cartlidge PHT, Rutter N 1992 Skin barrier function. In: Polin RA, Fox WW (eds) Fetal and Neonatal Physiology. WB Sanders, Philadelphia, pp 569–585
Evans NJ, Rutter N 1986 Development of the epidermis in the newborn. Biol Neonate 49: 74–80
Barker N, Hadgraft J, Phil D, Rutter N 1987 Skin permeability in the newborn. J Invest Dermatol 88: 409–411
Harpin VA, Rutter N 1983 Barrier properties of newborn infant's skin. J Pediatr 102: 419–425
Okah FA, Wickett RR, Pickens WL, Hoath SB 1995 Surface electrical capacitance as a noninvasive bedside measure of epidermal barrier maturation in the newborn infant. Pediatrics 96: 688–694
Rowen JL, Atkins JT, Levy ML, Baer SC, Baker CJ 1995 Invasive fungal dermatitis in the ≤1000-gram neonate. Pediatrics 95: 682–687
Lorenz JM, Kleinman LI, Ahmed G, Markarian K 1995 Phases of fluid and electrolyte homeostasis in the extremely low birth weight infant. Pediatrics 96: 484–489
Aszterbaum M, Menon GK, Feingold KR, Williams ML 1992 Ontogeny of the epidermal barrier to water loss in the rat: correlation of function with stratum corneum structure and lipid content. Pediatr Res 31: 308–317
Hanley K, Rassner U, Elias PM, Williams ML, Feingold KR 1996 Epidermal barrier ontogenesis: maturation in serum-free media and acceleration by glucocorticoids and thyroid hormone but not selected growth factors. J Invest Dermatol 106: 404–411
Hodson WA Normal and abnormal structural development of the lung, In: Polin RA, Fow WW (eds) Fetal and Neonatal Endocrinology. WB Sanders, Philadelphia, pp 771–782.
Bourbon JR, Farrell PM, Doncet E, Brown DJ, Valenza C 1987 Biochemical maturation of fetal rat lung: a comprehensive study including surfactant determination. Biol Neonate 52: 48–60
Shimizu H, Miyamura K, Kuroki Y 1990 Appearance of surfactant proteins, SP-A and SP-B, in developing rat lung and the effects ofin vivo dexamethasone treatment. Biochim Biophys Acta 1081: 53–60
Aszterbaum M, Feingold KR, Menon GM, Williams ML 1993 Glucocorticoids accelerate fetal maturation of the epidermal permeability barrier in the rat. J Clin Invest 1: 2703–2708
Prunieras M, Regnier M, Woodley D 1983 Methods of cultivation of keratinocytes with an air-liquid interface. J Invest Dermatol 81: 28s–33s.
Williams ML, Brown BE, Monger DJ, Grayson S, Elias PM 1988 Lipid content and matabolism of human keratinocyte cultures grown at the air-medium interface. J Cell Physiol 136: 103–110
Mak VHW, Cumpstone MV, Kennedy AH, Harmon CS, Guy RH, Potts RO 1991 Barrier function of human keratinocyte cultures grown at the air-medium interface. J Invest Dermatol 96: 323–327
Cohen S, Elliot GA 1963 The stimulation of epidermal keratinisation by a protein isolated from the submaxillary gland of the mouse. J Invest Dermatol 40: 1–5
Evans NJ, Rutter N, Gregory H 1985 Urinary excretion of epidermal growth factor in the newborn. Early Hum Dev 14: 277–282
Polk DH, Fisher DA Fetal and neonatal endocrinology. In: DeGroot L (ed) Endocrinology. WB Sanders, Philadelphia, pp 2239–2257.
Mancini AJ, Sookdeo-Drost S, Madison KC, Smoller BR, Lane AT 1994 Semipermeable dressings improve epidermal barrier function in premature infants. Pediatr Res 36: 306–314
Grubauer G, Elias PM, Feingold KR 1989 Transepidermal water loss: the signal for recovery of barrier structure and function. J Lipid Res 30: 323–333
Lee SH, Elias PM, Menon GK, Molo-Quiang M, Feingold KR 1992 Calcium and potassium are important regulators of barrier homeostasis in murine epidermis. J Clin Invest 89: 530–538
Menon GK, Elias PM, Lee SH, Feingold KR 1992 Localization of calcium in murine epidermis following disruption and repair of the permeability barrier. Cell Tissue Res 270: 503–512
Menon GK, Price LF, Bommannan B, Elias PM, Feingold KR 1994 Selective obliteration of the epidermal calcium gradient leads to enhanced lamellar body secretion. J Invest Dermatol 102: 789–795
Proksch E, Holleran WM, Menon GK, Elias PM, Feingold KR 1993 Barrier function regulates epidermal lipid and DNA synthesis. Br J Dermatol 128: 473–482
Acknowledgements
The authors appreciate the expert technical assistance of Debra Crumrine.
Author information
Authors and Affiliations
Additional information
Supported by the National Institutes of Health Grants HD 29706, AR 19098, PO 39448, and AR 39639 and the Medical Service, Department of Veterans Affairs Medical Center.
Rights and permissions
About this article
Cite this article
Hanley, K., Jiang, Y., Elias, P. et al. Acceleration of Barrier Ontogenesis in Vitro through Air Exposure. Pediatr Res 41, 293–299 (1997). https://doi.org/10.1203/00006450-199702000-00022
Received:
Accepted:
Issue Date:
DOI: https://doi.org/10.1203/00006450-199702000-00022
This article is cited by
-
A new dynamic culture device suitable for rat skin culture
Cell and Tissue Research (2019)
-
Development of Solar UVR-Related Pigmentation Begins as Early as the First Summer of Life
Journal of Investigative Dermatology (2010)
-
PPARδ Activation Promotes Stratum Corneum Formation and Epidermal Permeability Barrier Development during Late Gestation
Journal of Investigative Dermatology (2010)