Abstract
Mutation analysis for five families with Wiskottt-Aldrich syndrome was performed. The mutations found were two missense mutations, two one-base deletion mutations, and a large deletion mutation in the WASP gene. The three mutations had been reported before, but the remaining two were new. We used the mutation information to determine the carrier status of the female relatives of the patients. Three different approaches were taken, depending on the type of mutation, and the carrier determination was successfully performed.
Similar content being viewed by others
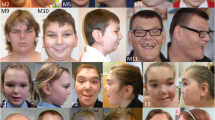
Main
WAS is one of the primary immunodeficiency diseases that is inherited as an X-linked recessive trait. The disease is characterized clinically by the triad of small cell-sized thrombocytopenia, recurrent infections due to defects in the immune system, and severe eczema(1).
Without positive familial information, the diagnosis of WAS has sometimes been difficult because the triad is usually absent during an initial evaluation(2). The carrier status for the female relatives of WAS patients has been determined by a linkage analysis of the genetic markers or by the X-chromosomal inactivation pattern(3, 4); however, because those carrier diagnoses are based on indirect information, there have been some cases in which diagnosis was impossible(5, 6). Therefore, we have not always been able to make a definite carrier diagnosis for patients' female relatives.
The responsible gene for WAS, WASP, has recently been isolated by positional cloning(7). The WASP gene spans approximately 9 kb of the genomic DNA and encodes a 502-amino acid proline-rich protein. Recently, Symons et al.(8) reported that the WASP has a GTPase binding site and that it interacts specifically with activated CDC42Hs, a member of the Rho-like GTPase family. They proposed that the WASP provides a link between CDC42Hs and the actin cytoskeleton.
Since the WASP gene has been identified, various mutations in the gene have been detected in patients with WAS, and it was revealed that X-linked thrombocytopenia resulted from a mutation of the same gene(9–14). Finding a mutation in the WASP gene allows us to confirm the clinical diagnosis of WAS in a sporadic occurrence or an atypical patient. Information on the patient's mutation in the WASP gene could be used to determine the carrier status for the patient's female family members. Here we show a mutation analysis for six patients, from five unrelated Japanese families with WAS, and the determination of the carrier status for the female relatives of the patients.
METHODS
Patients. Data of six patients from five Japanese families with WAS were included in this study. The patients were all male, and the diagnosis of WAS was based on their clinical, familial, and laboratory findings(Table 1). Two of the patients had died before the the start of this study. Case 1 died from severe infection at 20 y of age with an EBV-related lymphoproliferative disorder(15), case 2-1 died from non-Hodgkin lymphoma at 1 y of age. In both cases, their frozen spleen samples were included for use in this study. Cases 2-1 and 2-2 were maternal cousins, therefore, it was supposed that they had the same genetic defect and that their mothers were carriers for the disease. Bone marrow transplantations were successfully performed for case 2-2 and case 3, whose DNA samples were obtained before the therapy.
Mutation analysis. Genomic DNA was purified from the patients' peripheral lymphocytes, granulocytes, and frozen spleen cells by the standard phenol-chloroform method. In some cases, EBV-transformed cell lines were established and were used for DNA purification. To amplify the fragments including each exon of the WASP gene, 0.3-1.0 μg of DNA was PCR-amplified in a 20-mL volume using a buffer consisting of 50 mM KC1, 10 mM Tris-HC1, pH 8.0, 200 mM dNTPs, a 100 pM concentration of the exon specific for each pair of primers, and 2.5 U of Amplitaq polymerase (Perkin-Elmer, Foster City, CA). The PCR primers, some of which were as described in previous reports(9, 10, 13), and the annealing temperature used for PCR are listed in Table 2. The conditions used for PCR were denaturation at 94 °C for 30 s, annealing at each temperature for 30 s, and polymerization at 72 °C for 30 s. Twenty-five cycles of amplification were performed using a GeneAmp PCR System 2400 (Perkin-Elmer). The amplified fragments were purified and then directly sequenced by using an ABI PRISM Dye Terminator Cycle Sequencing Ready Reaction Kit (Perkin-Elmer) and an automated ABI 373A DNA sequencer. Primers used for sequencing were the same as those used for PCR at a concentration of 3.2 pM. Each mutation was confirmed at least twice using a different set of the PCR products.
Southern blot analysis was performed as previously described(16). The restriction enzymes used were obtained from Takara Shuzo Co. Ltd. (Kyoto, Japan). The PCR products of each fragment from the normal control DNAs were cloned using a TA cloning kit (Invitrogen Co., San Diego, CA) and then used as probes after confirmation by sequencing.
Carrier diagnosis based on the mutation detected. We used the mutation information to determine the carrier status of the patients' female relatives. An obligate carrier has the mutation allele and the normal allele, and a noncarrier possesses only the normal allele; therefore, we attempted to determine whether the candidates had the mutant allele, or not. We approached the diagnosis using three methods, depending on the mutation type. PCR using the allele-specific primers was applied for the family with the missense mutation. Direct sequencing of the fragment including the mutation was useful for the one-base deletion family. Southern blot analysis was used for the family with the large deletion within the gene.
RESULTS
We found missense mutations in two cases of WAS (Fig. 1). Case 3 was found to have a mutation in exon 1, GAG to AAG which replaced Glu-31 by Lys. The mutation in case 4 was found to be located in exon 2, ACG to ATG, which substituted Thr-45 by Met. These mutations were reported previously as being the responsible mutations for WAS patients(11, 13).
Sequencing analysis of cases 1-4 with WAS. PCR products of the fragments including each of the WASP gene exons were directly sequenced. Except for case 4, the sequence of the sense strand is shown; the sequence of the antisense strand is shown for case 4. Case 1 showed a one C deletion from five consecutive Cs in exon 10, which would result in a frame shift change. Case 2 (the result of case 2-1 is shown) showed the same kind of mutation as did case 1, that being a one-G deletion from five consecutive Gs in exon 1. Case 3 and 4 had missense mutations, G to A in exon 1 and C to T (G to A in the antisense strand in the figure) in exon 2, respectively.
Case 1 and cases 2-1 and 2-2 had a one-base deletion in exon 10 and exon 1, respectively (Fig 1). Case 1's mutation was CCCCC to CCCC in exon 10, which resulted in a frame shift change after Pro-352, and the mutation in cases 2-1 and 2-2 was GGGGG to GGGG in exon 1, which also resulted in a frame shift change after Gly-112. The same mutation in case 1 had been reported previously(12). Both the frame shift changes would create premature termination codons after codons 92 and 32 after the deletions, respectively.
Sequencing analysis of the PCR products including exons 1-2, 8, 9, 10, 11, 12, of case 5 revealed no abnormalities; however, we could not amplify the fragments with exons 3-4, 5-6, or 7 (Fig. 2). Southern blot analysis using part of the WASP cDNA strongly suggested that the patient had a deletion within the WASP gene. Abnormal bands were observed in the patient's DNA after it had been digested with several restriction enzymes and was hybridized with the fragment including exons 1-2, or the fragment including exon 9. No hybridized band was detected in the patient's DNA using the probe which included exons 3-4, 5-6, or 7 (data not shown). The deletion size seemed to be approximately 2.0 kb in length based upon the Hin dIII and EcoRI digestion patterns probed with the fragment of exons 1-2 and the fragment of exon 9. A more precise mutation analysis of case 5 has been reported elsewhere(17).
We applied the information of the mutations detected for determination of the carrier status in the female relatives of the WAS patients. The mutation detected in cases 2-1 and 2-2 was a deletion of one G from five consecutive Gs in exon 1. Cases 2-1 and 2-2 had a maternal aunt for determination of carrier status. Direct sequencing analysis of her PCR fragment, including the mutation site in exon 1, indicated that she was not a carrier for the disease. The sequence of the mothers of cases 2-1 and 2-2, who were supposed to be carriers for the disease, looked ambiguous just after the deletion mutation site. On the other hand, the sample from the aunt showed the same pattern as that of the normal controls, and there was no ambiguous sequence (Fig. 3).
Carrier determination for the family of case 2 (2-1 and 2-2). The PCR fragments including the mutation sites (exon 1) derived from the patient (case 2-1), his mother, and the single maternal aunt were sequenced. The sequence of the patient's mother became ambiguous just after the mutation site, whereas the sequence of the aunt was not ambiguous and was the same as that of the control individuals. The same sequencing results were obtained from PCR fragments of case 2-2 and his mother.
The mutation of case 3 was a one-base change of G to A in exon 1. We set up two pairs of primers (M, for the mutation allele; N, for the normal allele) to amplify the mutation and the normal allele, respectively. The primers consisted of the common sense primer(GGTTTTTTGCATTTCCTGTTC) and the antisense primer specific for M(TCAAAGAGTCGCTGGTTCT T) or N(TCAAAGAGTCGCTGGTTCT C), respectively. The conditions for PCR were denaturation at 94 °C for 30 s, annealing at 65 °C for 30 s, and polymerization at 72 °C for 30 s. Twenty-five cycles of amplification were performed. It was clearly shown that the patient's mother was the carrier for the disease, and his sister was not. Under the condition of using the M primers, the PCR product of the patient showed a band, whereas the normal control sample showed a band only when the primers for N were used. His mother showed a band under both conditions, and his sister showed a band only under the N primers (Fig. 4). These findings were confirmed by sequencing analysis of their PCR products (data not shown).
Carrier determination for the family of case 3. We set up two pairs of the primers based of the mutation information of case 3;M for the mutation allele and N for the normal allele (see“Results”). The PCR product of the patient showed a band under only the condition when the M primers were used, whereas the normal control showed a band only when the N primers were used. His mother(Mo) showed a band under both the conditions, and his sister(Si) showed a band only under the condition when the N primer were used. Pa, patient; Fa, father.
The carrier determination for the family of case 5 was performed by Southern blot analysis. Using the fragment which included exon 9 as a probe, the patient's DNA showed an abnormal band when digested with several restriction enzymes. The mother of the patient was revealed to be a carrier for the disease, because her digested DNA showed the same combination pattern as the patient and a normal control. For example, BamHI digestion revealed that the patient had an abnormal 8-kb band instead of a normal 6-kb band, and that the mother had both the 8-kb and the 6-kb bands. The same kind of pattern was observed with PvuII digestion (Fig. 5).
Carrier detection for the family of case 5. With Southern blot analysis using the fragment including exon 9, the patient showed abnormally large bands compared with a normal control, when digested with Bam HI or PvuII. The mother (Mo) showed the mixed pattern of the patient (Pa) and the control (C).
DISCUSSION
We studied the genetic analysis for the six patients with WAS from five different Japanese families and found the mutation responsible for the disease. Three of the mutations were already reported elsewhere(11–13), but the remaining two were new. Although the mutation of the WASP gene seemed to vary greatly(18), there could be some significant site for mutation. The same mutations found in WAS patients from different races may suggest that the three mutations we found are potential areas for mutation location. The two novel mutations were thought to result in the disruption of the greater part of the WAS protein. The mutation found in cases 2-1 and 2-2 was a one-base deletion in exon 1. This mutation leads to a frame shift change and thus creates a premature termination codon in exon 1, which induces truncation of the greater part of the WAS protein. The mutation in case 5 was a large deletion within the WASP gene. It is very unlikely that case 5 possessed the functional WAS protein. Thus, the two novel mutations we found could be responsible for the disease.
Using the information on the patients' mutations, we attempted to determine the carrier status for the female relatives for cases 2-1, 2-2, 3, and 5. The carrier diagnosis for the female relatives of WAS patients has been performed by genetic marker linkage, or X-chromosomal inactivation pattern. Although the genetic marker linkage method has recently been improved(19), we cannot expect to accurately determine all the candidates for the carrier state. The obligate carrier of WAS showed nonrandom inactivation of the X chromosome at the hematopoietic stem cell stage(20). Then, all kinds of hematologic cells of the carrier are supposed to show a nonrandom inactivation pattern of the X chromosome; however, rare exceptions were observed for the carriers of the disease. Furthermore, a recent report suggested that a skewing X-chromosomal activation pattern was not exceptional in normal females and that the incidence of excessive skewing increased with age(21). Because the carrier diagnoses must be performed correctly, a more definite and direct method should be recommended.
Once the mutation of the responsible gene is detected in a patient with some form of genetic disease, definitive carrier determination of the patient's family should be possible. There are several ways to diagnose the carrier status based on the mutation information. We previously showed the determination of the carrier status in the sisters or the mothers of the patients with X-linked chronic granulomatous disease. In cases in which there was a deletion, or a mutation that destroyed some part of the restriction site in the cording region of the gp91-phox gene, Southern blot analysis was used(22, 23). In the case in which there was a small insertion/deletion that resulted in a change in the size of the PCR product, we could determine the carrier status just from the size of the PCR products on the gel(24).
We set two pairs of the primers (M and N) for the family of case 3. The difference between M and N was only one base at the end of 3′ site of the antisense primers, and it came from the mutant or the normal sequence. The common sense primer was used. Strict PCR conditions worked well for distinguishing the mutant allele from the normal allele, which allowed us to diagnose the patient's mother as being a carrier for the disease and his sister as not a carrier (Fig. 3). Theoretically, this approach might also be useful for the family of case 4; however, those samples were not available.
We did not take the same approach to the carrier diagnosis for the family of cases 2-1 and 2-2. Because the mutation was a one-G deletion from five consecutive Gs, it seemed that it would be difficult to set the specific pairs of primers for use in the PCR method, which was useful for the diagnosis of the case 3 family. Instead, the PCR products from the patients, their mothers, their maternal aunt, and the control individuals were directly sequenced. As expected, their mothers were confirmed as the carriers for the disease because the sequence just after the mutation site became ambiguous. The ambiguous sequence seemed to be the result of a mixture of the mutant fragment and the normal fragment. This speculation was confirmed by the same ambiguous sequencing results obtained from the PCR products from DNA mixture of the patient and healthy control. On the other hand, their aunt proved not to be a carrier because there was no ambiguous sequence, and the sequence was the same as that of the normal controls (Fig. 4). This approach would also be useful for the case 1 family, whose mutation was a one-C deletion from five consecutive Cs in exon 10; however, the family samples were not available. The result of a sequencing study of a PCR product generated from the mixture of DNA from case 1 and a healthy individual reinforced this possibility for application (data not shown).
The mutation in case 5 was a large deletion within the WASP gene. Generally, Southern blot analysis is available for carrier detection, as in the case of the deletion mutation in the family previously described. Thus, using the fragment near the deletion area as a probe, we could show that the mother of case 5 was a carrier for the disease (Fig. 5). Although this kind of mutation has been observed in various genetic diseases, no such case has been reported in the WASP gene. Characterization of this deletion mutation detected in case 5 has been reported elsewhere(17).
Detection of mutations in the WASP gene in clinically suspected WAS patients will provide various benefits. First, we can confirm the clinical diagnosis of WAS. As mentioned in the introduction, the diagnosis of a patient with WAS is sometimes difficult, especially when the patient does not have a positive familial history. Second, as we reported, the carrier determination of the female relatives of the patient will be definitive and easily obtained. In the same way, prenatal diagnosis for the WAS candidates in the patient's family will be possible. Finally, a large amount of data on WASP gene mutations in WAS patients with various clinical phenotypes will shed light on the role of the WAS protein in vivo.
We have had to reassess our approach to some genetic diseases, since their causative genes have been identified. For example, some atypical cases of X-linked agammaglobulinemia, with some B cells in the peripheral blood and with small levels of serum immunoglobulin, was reported(25). The diagnosis could be made because the mutations in the responsible gene (Btk, Bruton's tyrosine kinase) were detected. Without genetic analysis, however, the diagnosis would be missed, as the patients showed no agammaglobulinemia. Thus, identification of the responsible gene for a genetic disease may expand its clinical phenotype and reconstitute the disease entity. We may have to reorganize WAS and X-linked thrombocytopenia as the same disease entity, because not only do they share the same responsible gene, but the same mutations were reported from patients with either of the diseases(18).
Abbreviations
- WAS:
-
Wiskott-Aldrich syndrome
- WASP:
-
Wiskott-Aldrich syndrome protein
- PCR:
-
polymerase chain reaction
References
Aldrich RA, Steinberg AG, Campbell DC 1954 Pedigree demonstrating a sex-linked recessive condition characterized by draining ears, eczematoid dermatitis and bloody diarrhea. Pediatrics 13: 133–139
Sullivan KE, Mullen CA, Blaese RM, Winkelstein JA 1994 A multiinstitutional survey of Wiskott-Aldrich syndrome. J Pediatr 125: 876–885
Fearon ER, Kohn DB, Winkelstein JA, Vogelstein B, Blaese RM 1988 Carrier detection in the Wiskott Aldrich syndrome. Blood 72: 1735–1739
Notarangelo LD, Parolini O, Faustini R, Porteri V, Albertini A, Ugazio AG 1991 Presentation of Wiskott-Aldrich syndrome as isolated thrombocytopenia [etter]. Blood 77: 1124–1128
Notarangelo LD, Parolini O, Porta F, Locatelli F, Lanfranchi A, Marconi M, Nespoli L, Albertini A, Craig IW, Ugazio AG 1991 Analysis of X-chromosome inactivation and presumptive expression of the Wiskott-Aldrich syndrome (WAS) gene in hematopoietic cell lineages of a thrombocytopenic carrier female of WAS. Hum Genet 88: 237–241
de Saint-Basile G, Schlegel N, Caniglia M, Le Deist F, Kaplan C, Lecompte T, Piller F, Fischer A, Griscelli C 1991 X-linked thrombocytopenia and Wiskott-Aldrich syndrome: similar regional assignment but distinct X-inactivation pattern in carriers. Ann Hematol 63: 107–110
Derry JMJ, Ochs HD, Francke U 1994 Isolation of a novel gene mutated in Wiskott-Aldrich syndrome. Cell 78: 635–644
Symons M, Derry JMJ, Karlak B, Jiang S, Lemahieu V, McCormick F, Francke U, Abo A 1996 Wiskott-Aldrich syndrome protein, a novel effector for the GTPase CDC42Hs, is implicated in actin polymerization. Cell 84: 723–734
Villa A, Notarangelo L, Macchi P, Mantuano E, Cavagni G, Brugnoni D, Strina D, Patrosso MC, Ramenghi U, Sacco MG, Ugazio A, Vezzoni P 1995 X-linked thrombocytopenia and Wiskott-Aldrich syndrome are allelic diseases with mutations in the WASP gene. Nat Genet 9: 414–417
Derry JMJ, Kerns JA, Weinberg KI, Ochs HD, Volpini V, Estivill X, Walker AP, Francke U 1995 WASP gene mutations in Wiskott-Aldrich syndrome and X-linked thrombocytopenia. Hum Mol Genet 4: 1127–1135
Kolluri R, Shehabeldin A, Peacocke M, Lamhonwah A-M, Teichert-Kuliszewska K, Weissman SM, Siminovitch KA 1995 Identification of WASP mutations in patients with Wiskott-Aldrich syndrome and isolated thrombocytopenia reveals allelic heterogeneity at the WAS locus. Hum Mol Genet 4: 1119–1126
Wengler GS, Notarangelo LD, Berardelli S, Pollonni G, Mella P, Fasth A, Ugazio AG, Parolini O 1995 High prevalence of nonsense, frame shift, and splice-site mutations in 16 patients with full-blown Wiskott-Aldrich syndrome. Blood 86: 3648–3654
Kwan S-P, Hagemann TL, Radtke BE, Blaese RM, Rosen FS 1995 Identification of mutations in the Wiskott-Aldrich syndrome gene and characterization of a polymorphic dinucleotide repeat at DXS6940, adjacent to the disease gene. Proc Nat Acad Sci USA 92: 4706–4710
Zhu Q, Zhang M, Blaese RM, Derry J.MJ, Junker A, Francke U, Chen S-H, Ochs HD 1995 The Wiskott-Aldrich syndrome and X-linked congenital thrombocytopenia are caused by mutations of the same gene. Blood 86: 3797–3804
Nakanishi M, Kikuta H, Tomizawa K, Kojima K, Ishizaka A, Okano M, Sakiyama Y, Matsumoto S 1993 Distinct clonotypic Epstein-Barr virus-induced fatal lymphoproliferative disorder in a patient with Wiskott-Aldrich syndrome. Cancer 72: 1376–1381
Whitehead AS, Woods DE, Fleishnick E, Chin JE, Yunis EJ, Katz AJ, Gerald PS, Alper CA, Colten HR 1984 DNA polymorphism of C4 genes: a new marker for analysis of the major histocompatibility complex. N Engl J Med 310: 88–91
Ariga T, Yamada M, Ito S, Iwamura M, Iseki M, Sakiyama Y 1997 Characterization of a deletion mutation involving exons 3-7 of the WASP gene which was detected in a patient with Wiskott-Aldrich syndrome. Hum Mutat( in press)
Remold-O'Donnell E, Rosen FS, Kenney DM 1996 Defects in Wiskott-Aldrich syndrome blood cells. Blood 87: 2621–2631
Kwan S-P, Hagemann TL, Blaese RM, Rosen FS 1995 A high resolution map of genes, microsatellite markers and new dinucleotide repeats from UBEI to the GATA locus in the region Xp11.23. Genomics 29: 247–251
Wengler G, Gorlin JB, Williamson JM, Rosen FS, Bing DH 1995 Nonrandom inactivation of the X chromosome in early lineage hematopoietic cells in carriers of Wiskott-Aldrich syndrome. Blood 85: 2471–2477
Busque L, Mio R, Mattioli J, Brais E, Blais N, Lalonde Y, Maragh M, Gilliland DG 1996 Nonrandom X-inactivation patterns in normal females; lyonization ratios vary with age. Blood 88: 59–65
Ariga T, Nakanishi M, Tomizawa K, Imajoh-Ohmi S, Kanegasaki S, Sakiyama Y, Matsumoto S 1992 Genetic heterogeneity in patients with X-linked recessive chronic granulomatous disease. Pediatr Res 31: 516–519
Ariga T, Sakiyama Y, Furuta H, Matsumoto S 1994 Molecular genetic studies of two families with X-linked chronic granulomatous disease: mutation analysis and definitive determination of carrier status in patients' sisters. Eur J Haematol 52: 99–102
Ariga T, Sakiyama Y, Matsumoto S 1995 A 15-base pair(bp) palindromic insertion associated with a 3-bp deletion in exon 10 of the gp91-phox gene, detected in two patients with chronic granulomatous disease. Hum Genet 96: 6–8
Ohta Y, Haire RN, Litman RT, Fu SM, Nelson RP, Kratz J, Kornfeld SJ, de la Morena M, Good RA, Litman GW 1994 Genomic organization and structure of Bruton agammaglobulinemia tyrosine kinase: localization of mutations associated with varied clinical presentations and course in X chromosome-linked agammaglobulinemia. Proc Natl Acad Sci USA 91: 9062–9066
Acknowledgements
The authors thank Drs. Tatsuzawa, Iwamura, and Iseki for allowing us to analyze their patient samples.
Author information
Authors and Affiliations
Additional information
Supported by a grant from the Ministry of Health and Welfare, Japan.
Rights and permissions
About this article
Cite this article
Ariga, T., Yamada, M. & Sakiyama, Y. Mutation Analysis of Five Japanese Families with Wiskott-Aldrich Syndrome and Determination of the Family Members' Carrier Status Using Three Different Methods. Pediatr Res 41, 535–540 (1997). https://doi.org/10.1203/00006450-199704000-00013
Received:
Accepted:
Issue Date:
DOI: https://doi.org/10.1203/00006450-199704000-00013