Abstract
Ovarian cancer is believed to develop from the ovarian surface epithelium through the accumulation of aberrations of oncogenes and/or tumor suppressor genes. However, it is unclear how the gene abnormalities are involved in ovarian carcinogenesis. To elucidate the process, we transfected genes reported to show their abnormalities in human ovarian cancers into human ovarian surface epithelial cells. Immortalization of the cells was achieved by the transfection of SV40 large T antigen (LT) and human telomerase reverse transcriptase (hTERT); however, the resultant cells showed no tumorigenesis. Additional transfection of either c-erbB-2 or mutant Ha-ras into the immortalized cells showed the anchorage-independent growth and tumorigenesis in mice with the incidence of 50% and 40%, respectively. Histologically, all the tumours were undifferentiated. In association with the tumorigenesis, the cells expressing c-erbB-2 or mutant Ha-ras demonstrated increased vascular endothelial growth factor secretion under hypoxia and enhanced resistance to apoptosis compared with the immortalized cells. Collectively, the introduction of either c-erbB-2 or mutant Ha-ras in the cells, which were efficiently immortalized by the transfection of LT and hTERT, showed tumorigenicity, suggesting that c-erbB-2 or mutant Ha-ras genes might be involved in ovarian carcinogenesis.
Similar content being viewed by others
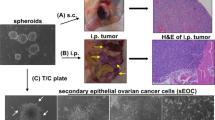
Main
Ovarian cancer is the leading cause of death in women with gynaecological malignant tumours (Herbst, 1994). Despite the recent developments in aggressive surgery and chemotherapy, the prognosis remains poor because ovarian cancers are often not diagnosed until advanced stages and the cancer cells frequently acquire drug resistance during repeated chemotherapies. Thus, there is an urgent need to develop new therapies. Epithelialovarian cancer is believed to develop from the ovarian surface epithelium (OSE) (Scully, 1977), through the accumulation of aberrations of oncogenes and/or tumour suppressor genes. Many gene abnormalities have been detected in ovarian cancers, for example, the overexpression of c-erbB-2, mutation of Ki-ras, p53, BRCA1 or BRCA2, and the expression of telomerase activity (Matias-Guiu and Prat, 1998). Nonetheless, it is still unclear how the gene abnormalities are involved in the process of ovarian carcinogenesis.
Human OSE cells provide a useful in vitro culture system for the analysis of pathogenesis of ovarian cancers using molecular biology and genetic techniques. Two studies on malignant transformation of human OSE cells by gene transfection have been reported (Ong et al, 2000; Nitta et al, 2001). A recent report demonstrated that transfection of human telomerase reverse transcriptase (hTERT) into SV40 large T antigen (LT)-transfected human somatic cells showed 100% immortalization in transfected cells (Hahn et al, 1999). Therefore, in the present study, we tried to induce malignant transformation of cultured human OSE by transfecting genes that were shown to be altered in human ovarian cancers. We also tried to analyse the phenotypic change induced in the transformation process.
Materials and methods
Cell culture
Human OSE cells were obtained with written consent from patients who underwent abdominal surgery for benign gynaecological diseases. Ovarian surface epithelium cells were collected during surgery and cultured as previously reported (Kuroda et al, 2001). The purity of the cultured OSE cells was confirmed by positive immunostaining of both cytokeratin and vimentin with negative staining of factor-VIII-related antigen (Auersperg et al, 1994). The human ovarian cancer cell line A2780 was maintained as previously reported (Tsuruta et al, 2001). The fibroblast cell line NIH 3T3 cells were cultured in Dulbecco's modified Eagle's medium (DMEM) (Nissui, Tokyo, Japan) with 10% FCS.
Gene transfection
Subconfluent cultures of OSE cells in passage 1 were transfected with 20 μg of pSV3neo, which contains LT gene, using the Lipofectin reagent (Gibco, NY, USA). Next, we transfected 20 μg of hTERT plasmid (pcDNA3-hTERTn2) into LT-transfected OSE cells. Cells expressing the hTERT gene were selected in media supplemented with 400 μg ml−1 of G418 and were cloned. We finally transfected 20 μg of the c-erbB-2 plasmid (pSV2erbB2) or the mutant Ha-ras plasmid (pSNTT) into hTERT- and LT-transfected OSE cells with 5 μg pHygEGFP vector (Clontech, CA, USA). We harvested those clones that fluoresced as successfully transfected cells.
Western blotting
Western blotting was performed as previously described (Tsuruta et al, 2001). We used the following antibodies as a probe: anti-SV40 mouse monoclonal antibody (Santa Cruz, CA, USA), anti-Ha-ras mouse monoclonal antibody (Santa Cruz) and anti-c-erbB-2 rabbit polyclonal antibody (Nichirei, Tokyo, Japan).
RT–PCR analysis
The expression of hTERT mRNA and the telomerase activity were performed by RT–PCR and by stretch PCR assay, respectively, as previously reported (Kyo et al, 1999).
Immunohistochemistry
Tumour tissues were fixed in 10% buffered formalin, embedded in paraffin and sectioned. Sections were stained with haematoxylin and eosin. For the immunohistochemical staining, they were deparaffinized in xylol for 30 min and processed as previously described (Kuroda et al, 2001). We used the following antibodies as primary antibodies: a rabbit polyclonal anti-cytokeratin antibody (Santa Cruz), a rabbit polyclonal anti-vimentin antibody (Santa Cruz), a mouse monoclonal anti-CA125 antibody (Dako, CA, USA) or a mouse monoclonal anti-SV40 large T antigen (Santa Cruz).
Life span determinations
Cells were seeded on 35 mm collagen type-I-coated culture dishes. When confluent, the cells were trypsinized and seeded at 1 : 3 split ratio. This process was continued until the cells became stationary (senescence), that is did not become confluent after 1 month in culture. The population doublings (PDs) were added for each passage until the stationary phase was reached.
Assay for anchorage independence
Colony formation in semisolid agar were assayed by suspending 104 cells in 2 ml appropriate medium with 0.33% agarose (Gibco) and placing this suspension on the top of 5 ml solidified 0.5% agarose in the above medium. Triplicate cultures for each cell clone were maintained at 37°C in a 5% CO2 atmosphere. Colonies over 50 μm in diameter were counted after 2 weeks. The experiments were repeated three times for each clone.
Tumorigenicity assays
Female BALB/C nude mice, 4–6 weeks old, were purchased from CLEA JAPAN (Tokyo, Japan). The animals received proper care according to the rules of the Kyoto University Committee on Animal Care. Animal experiments were performed comply with the United Kingdom Co-ordinating Committee on Cancer Research (UKCCCR) Guidelines (Workman et al, 1988). Approximately 108 cells per animal were injected subcutaneously in nude mice. If subcutaneous mass was confirmed, they were killed and the tumours resected. Cells were considered nontumorigenic if mouse failed to form tumour within 4 months of injection.
Growth parameters
Population doubling time (PDT) was determined as described below. Cells (104 cells well−1) were seeded in each well of a collagen type-I-coated 24-well plate. Duplicate cell counts were determined daily for 7 days using a cell counter (Coulter, FL, USA). The natural logarithm of the mean number of cells per dish was plotted as a function of time in hours. The maximum doubling time was calculated from the steepest part of the plot. The experiments were repeated three times for each clone.
Measurement of bromodeoxyuridine (BrdU) incorporation rate by flow cytometry
The measurement of BrdU incorporation rate by flow cytometry was performed using the FITC-conjugated BrdU antibody (Anti-BrdU) (Becton-Dickinson, Bedford, MA, USA) according to the manufacturer's instructions. Briefly, 108 cells of each sample were incubated with 10 μ M BrdU (Nacalai tesque, Kyoto, Japan) for 30 min and washed with PBS. The cells were collected with cell scraper, fixed in 70% ethanol and denatured with 2 N Hydrochloric acid/Triton X-100 to produce single-stranded molecules. They were resuspended in 0.1 M Sodium Tetraborate Decahydrate, pH 8.5 to neutralise the acid. Then, they were incubated with anti-BrdU (Becton-Dickinson) and were resuspended in a DNA-staining solution containing propidium iodine and RNase (Cycle TESTTM PLUS DNA Reagent Kit) (Becton-Dickinson). The rate of BrdU incorporation was determined by a FACS Caliber flow cytometer (Becton-Dickinson) and analysed with CELL Quest (Becton-Dickinson). The experiments were repeated three times.
Measurement of apoptosis by ELISA using antihistone antibody
Apoptosis induced by serum deprivation was quantified by measurement of DNA fragmentation with Cell Death Detection ELISA (Roche, Mannheim, Germany) as previously described (Kuroda et al, 2001). Assays were performed in duplicate. Induced apoptosis was expressed as relative cell death, which was a ratio of apoptosis level detected in culture without serum to apoptosis level of control detected in culture with 15% serum. The experiments were repeated three times.
Measurement of human vascular endothelial growth factor (VEGF) secretion by ELISA
The level of vascular endothelial growth factor (VEGF) in culture supernatants was measured by AN'ALYZATM (TECHNE, MN, USA). Each assay was performed in duplicate. The cells were cultured on 60 mm collagen type-I-coated culture dishes. When confluent, all cultures were then maintained in medium with 15% FCS at 37°C under 20 or 1% O2 concentration for 72 h. Cells were collected with 2.5% trypsin and cell numbers were counted. The supernatants were collected and the amount of human VEGF in supernatants was measured with ELISA according to the manufacturer's protocol. The values of VEGF were standardised with cell number. The experiments were repeated three times.
Statistical analysis
For analysis of between-group differences, P-values were determined using Mann–Whitney's U-test. A P-value less than 0.05 was considered significant.
Results
Confirmation of introduced gene expression in OSE cells
To confirm the successful expression of introduced gene products in OSE cells, the expressions of LT, c-erbB-2 and mutant Ha-ras protein were confirmed by Western blotting, as shown in Figure 1A, B and C, respectively. The hTERT mRNA expression and telomerase activity in hTERT-introduced OSE cells were confirmed by RT–PCR (Figure 1D) and by stretch PCR assay (Figure 1E), respectively.
Protein expressions evaluated by Western blotting for LT (A), mutant Ha-ras (B) and c-erbB-2 (C). The expression of hTERT mRNA and telomerase activity in OSE cells by RT-PCR, strech PCR, respectively (D, E). (A) Lane 1: OSE cells; lane 2: LT-transfected OSE cells; lane 3: OSE cells transfected with a combination of LT and hTERT. (B). Lane 1: OSE cells; lane 2: OSE cells transfected with a combination of LT and hTERT; lane 3: OSE cells transfected with a combination of LT, hTERT and mutant Ha-ras. (C) Lane 1: OSE cells; lane 2: LT-transfected OSE cells; lane 3: OSE cells transfected with a combination of LT and hTERT; lane 4: OSE cells transfected with a combination of LT, hTERT and c-erbB-2. (D) Lane 1: OSE cells; lane 2: LT-transfected OSE cells; lane 3: OSE cells transfected with a combination of LT and hTERT. (E) Lane 1: positive control; lane 2: negative control; lane 3: LT-transfected OSE cells; lane 4: OSE cells transfected with a combination of LT and hTERT. IS=internal standard.
Malignant transformation of cultured OSE cells
To evaluate malignant tranformation of OSE cells transfected with various genes, we analysed immortalization, ability of anchorage-independent growth and tumorigenicity in mice. Untransfected OSE cells underwent senescence in 3±1 passages in culture. Transfection with LT extended the life span of OSE cells, but, these LT-transfected OSE cells (OSE+LT) underwent senescence in 9±2 passages. In contrast, the PDs of OSE cells transfected with a combination of LT and hTERT (OSE+LT+hTERT) was 116±11 passages and the cells did not achieve senescence, suggesting that they had been immortalized. Ovarian surface epithelium cells transfected with a combination of LT, hTERT and mutant Ha-ras (OSE+LT+hTERT+Ha-ras), and OSE cells transfected with a combination of LT, hTERT and c-erbB-2 (OSE+LT+hTERT+c-erbB-2) clones also were proliferating without senescence with PDs of 106±11 and 106±11 passages to date, respectively. As for growth ability in soft agar, the results of the assay for anchorage-independent growth are shown in Table 1.
OSE+LT and OSE+LT+hTERT showed slightly enhanced proliferative activity in soft agar compared with original OSE cells. The additional introduction of c-erbB-2 or mutant Ha-ras into OSE+LT+hTERT cells further enhanced the growth activity with statistical significance (P<0.05). OSE+LT+hTERT+c-erbB-2 cells showed significantly enhanced growth activity in soft agar compared with that of OSE+LT+hTERT+Ha-ras cells (P<0.05). However, compared with the A2780 ovarian cancer cell line, colonies of OSE+LT+hTERT+c-erbB-2 cells were small in number. We examined tumorigenicity in immunodeficient mice. As shown in Table 2, the OSE cells expressing LT or both LT and hTERT did not form tumours. In contrast, those cells additionally transfected with c-erbB-2 and mutant Ha-ras formed tumours in five of 10 cases (50%), four of 10 cases (40%), respectively, in nude mice within 1–3 months. On the other hand, injection of A2780 in nude mice formed tumour in 10 of 10 cases (100%) within 2–3 weeks.
Morphologic features of tumours formed in mice
Morphologic features of inoculated tumours were assessed by microscopic observation. Histologically, all the tumours expressing mutant Ha-ras were made up in major part of homogenous spindle-shaped cells with minimal nuclear atypia and infrequent mitoses (Figure 2A) Immunohistochemically, tumours were positive for cytokeratin and negative for vimentin, suggesting that they were epithelial tumours. All the tumours formed by the injection of c-erbB-2-expressing cells showed the same features as described above, except that they were positive for vimentin. All the tumours were confirmed to be derived from the injected OSE cells by immunohistochemical expression of LT protein (Figure 2B).
Microscopic and immunohistochemical findings of tumours formed in mice. (A) Microscopic findings of tumours expressing mutant Ha-ras. The tumours, irrespective of the introduced gene, c-erbB-2 or mutant Ha-ras, were shown to be composed of spindle-shaped cells with minimal nuclear atypia and less frequent mitoses. (B) Immunohistochemistry of tumour cells transfected with a combination of LT, hTERT and mutant Ha-ras, using the anti-SV40 large T antigen antibody. All tumour cells were positively stained for the antigen, indicating that these tumours originate from the injected transformed human OSE cells. Scale bar, 200 μm.
Effect of gene transfections on proliferative activity
To investigate whether introduced genes have an effect on proliferation activity in OSE cells during the malignant transformation, PDT measurement and BrdU incorporation assay were performed. LT-transfected OSE cells showed approximately one third the PDT of the original OSE cells, indicating that the proliferative activity accelerated. Moreover, OSE+LT+hTERT showed a significant decrease in PDT compared with OSE+LT. However, there were no significant decreases in PDT associated with the introduction of c-erbB-2 or mutant Ha-ras into OSE+LT+hTERT cells (Table 3). By a BrdU incorporation assay, the populations at the S phase of the total population in OSE+LT+hTERT were significantly increased compared with that of NIH 3T3, which has normal cell cycle (45.6±3.6, 1.6±1.4% respectively) (P<0.05). The population rate at S phase in OSE+LT+hTERT+Ha-ras and OSE+LT+hTERT+c-erbB-2 were not significantly different (45.3±5.3% and 42.6±6.9% respectively), consistent with the results of PDT (Table 4).
Effect of the genes on apoptosis resistance
To investigate the detail mechanisms of how c-erbB-2 or mutant Ha-ras genes are involved in the malignant transformation of OSE cells, the effect of these genes on apoptosis resistance was evaluated. Apoptosis was induced by serum deprivation for 72 h. The level of apoptosis in tumorigenic c-erbB-2- or mutant Ha-ras-expressing OSE cells was measured by ELISA and compared with that of the nontumorigenic OSE cells. Tumorigenic OSE+LT+hTERT+c-erbB-2 or mutant Ha-ras demonstrated the lower level of apoptosis, compared with immortalised OSE+LT+hTERT cells (P<0.05), demonstrating that the tumorigenic OSE cells possess enhanced resistance to apoptosis than the immortalized, but nontumorigenic OSE cells (Figure 3).
Levels of apoptosis induced by serum deprivation in the immortalised and tumorigenic OSE clones, which expressed as relative cell death. Relative cell death was a ratio of apoptosis level induced by culture without serum to apoptosis level of control detected in culture with 15% serum. The tumorigenic OSE cells expressing c-erbB-2 or mutant Ha-ras demonstrated the lower level of apoptosis compared with the immortalized OSE cells (P<0.05). Lane 1: OSE cells transfected with a combination of LT and hTERT, lane 2: OSE cells transfected with a combination of LT, hTERT and c-erbB-2, lane 3: OSE cells transfected with a combination of LT, hTERT and mutant Ha-ras.
Effect of the genes on VEGF secretion
We also analysed whether c-erbB-2 or mutant Ha-ras genes have effect on upregulation of VEGF secretion. When cells were cultured under hypoxic condition, VEGF level in supernatant, measured by ELISA, significantly increased, compared with that under room air for each immortalized or tumorigenic OSE group. As shown in Figure 4, in the hypoxic condition, VEGF level for tumorigenic OSE+LT+hTERT+c-erbB-2 or mutant Ha-ras was significantly higher than that for immortalized OSE+LT+hTERT cells (P<0.05).
Amount of human VEGF in culture supernatants of the immortalized and tumorigenic OSE clones. Cells were cultured under hypoxic condition of 1% O2, and VEGF level for tumorigenic OSE cells transfected with a combination of LT, hTERT and c-erbB-2 or mutant Ha-ras was significantly higher than that for immortalized OSE cells (P<0.05). Lane 1: OSE cells transfected with a combination of LT and hTERT, lane 2: OSE cells transfected with a combination of LT, hTERT and c-erbB-2, lane 3: OSE cells transfected with a combination of LT, hTERT and mutant Ha-ras.
Discussion
Epithelial ovarian cancer is believed to develop from the OSE (Scully, 1977), through the amplifications of oncogenes and mutations of tumour suppressor genes. Although many gene abnormalities have been detected in ovarian cancers (Matias-Guiu and Prat, 1998), their roles in the process of ovarian carcinogenesis have not yet been elucidated. Human OSE cells provide a useful experimental system for the analysis of ovarian carcinogenesis. Two studies on immortalization of human OSE cells by the introduction of LT alone have been already reported (Maines-Bandiera et al, 1992; Nitta et al, 2001). Maines-Bandiera et al reported that OSE lines transfected with LT exhibited an extended life span, but subsequently underwent a progressive reduction of growth and characteristic morphologic changes associated with senescence within 20 passages. Nitta et al reported that only six out of 10 OSE lines transfected with LT were immortalized, and they had very high telomerase activity. These results suggest that introduction of LT alone is not sufficient to acquire unlimited growth capacity, and additional genetic change, that is telomerase activation, is necessary to accomplish immortalization. It is also reported that the immortalization of somatic cells by the introduction of LT alone is very infrequent (Van Der Haegen and Shay, 1993). On the other hand, a recent report showed that the transfection of hTERT into LT-transfected human somatic cells immortalized with the incidence of 100% (Hahn et al, 1999). In the present study, we tried to immortalize cultured human OSE by the introduction of hTERT along with LT. As a result, PDs of these clones had already exceeded 100 without reaching senescence.
Immunohistochemically, the immortalized OSE cells expressing LT and hTERT were positive for cytokeratin, vimentin, but negative for CA125, and they were similar to the OSE cells of the primary culture (data not shown). Therefore, the immortalized OSE cells seem to maintain phenotypes similar to those of the original OSE cells, although the immortalized cells showed slight enhancement of growth ability in soft agar without tumorigenesis in mice. Thus, immortalized OSE cells expressing LT and hTERT are considered to be useful for the study of the oncogenic process of human ovarian cancers.
We selected c-erbB-2 and mutant Ha-ras for the additional gene transfection for the following reasons. Amplification and/or overexpression of the c-erbB-2 gene have been reported in 10–50% of all human epithelial ovarian cancers (Matias-Guiu and Prat, 1998). The ras oncoprotein consists of three subtypes, that is Ha-ras, Ki-ras and N-ras, all of which are thought to possess identical functions. The activation of Ha-ras by point mutation has been reported to be important for the genesis and maintenance of solid tumours (Chin et al, 1999). The Ki-ras mutation has been implicated in the pathogenesis of ovarian cancers (Matias-Guiu and Prat, 1998).
The additional introduction of c-erbB-2 or mutant Ha-ras in immortalized OSE cells triggered tumorigenesis in mice. However, the incidence of tumour formation was 50% and 40% in cases of c-erbB-2 and mutant Ha-ras introduction, respectively. The low incidence of tumour formation in our experiments cannot exclude the possibility that some unknown incidental genetic changes may be associated, especially because of p53 suppression by LT in the OSE cells. However, this possibility seems unlikely because all four tumours expressing mutant Ha-ras formed in the four different mice had similar histology with the same results of the immunohistochemical study. The consistency of histological features among the four different tumours strongly suggests that the malignant transformation was induced by the transfection of mutant Ha-ras. Five cases with tumours expressing c-erbB-2 also showed consistency in the phenotypic features.
The growth activity of immortalized OSE cells expressing either c-erbB-2 or mutant Ha-ras in soft agar were significantly lower when compared with that of the ovarian cancer cell line A2780, which showed tumorigenesis with 100% incidence. The latent period of tumour formation after injection of the former was 1–3 months, but that of the latter was 2–3 weeks. These results suggest that the malignant potential of immortalized OSE cells expressing either c-erbB-2 or mutant Ha-ras may be lower than that of A2780. The low malignant potential of immortalized OSE cells expressing either c-erbB-2 or mutant Ha-ras might be one of the causes of the low incidence of tumour formation in this study. Nonetheless, the loss of transfected genes after introduction by lipofection, or the varied efficiency of the post-transcriptional process of introduced genes may be the cause of the low incidence of tumor formation in this study. This seems unlikely because the immortalized cells expressing either c-erbB-2 or mutant Ha-ras showed extension of their lifespan with stable growth activity, and because none of the clones showed significant deviation in the results of anchorage-independent growth and PDT assays. Therefore, we concluded that the additional introduction of c-erbB-2 or mutant Ha-ras genes in the immortalized OSE cells was the cause of malignant transformation of human OSE cells, and these oncogenes might be playing an important role during the malignant transformation of OSE cells in vivo.
To clarify the mechanisms underlying introduction of c-erbB-2 or mutant Ha-ras genetic material into the OSE cells and the resultant malignant transformation, we compared OSE cells before and after transfection of c-erbB-2 or mutant Ha-ras. Interestingly, both the doubling times and the population rate at the S phase of the immortalized cells before the additional gene transfections and the tumorigenic cells expressing either c-erbB-2 or mutant Ha-ras were nearly identical. Such a result suggests that the malignant transformation induced by introduction of c-erbB-2 or mutant Ha-ras might proceed via signalling pathways unrelated to cell-cycle regulation. Resistance to apoptosis is considered to be one of the mechanisms underlying carcinogenesis, and genetic abnormalities of molecules regulating apoptosis have been detected in ovarian cancers (Matias-Guiu and Prat, 1998). The tumorigenic OSE cells expressing either mutant Ha-ras or c-erbB-2 demonstrated the enhanced resistance to apoptosis induced by serum-deprivation, compared with the immortalized OSE cells. Neovascularisation is also critical in tumour formation and VEGF is a major angiogenic factor. The tumorigenic OSE cells expressing either c-erbB-2 or mutant Ha-ras demonstrated the increased secretion of VEGF in hypoxic condition compared with the immortalized OSE cells. Such a finding suggests that tumorigenic cells might possess a greater capacity for neovascularisation. Reportedly, ras and c-erbB-2 genes actually demonstrated angiogenic function, upregulating the expression of VEGF in colon cancer and in head and neck squamous cell carcinomas, respectively (O-charoenrat et al, 2000; Zhang et al, 2001). Taken together, c-erbB-2 or mutant Ha-ras might allow malignant transformation of OSE cells via induction of resistance to apoptosis and enhancement of VEGF secretion.
In conclusion, immortalization of human OSE cells is induced efficiently by the transfection of hTERT in addition to LT. Although the incidence is not so high, introduction of either c-erbB-2 or mutant Ha-ras into immortalized cells results in formation of malignant tumours. Further study is necessary to elucidate the genes involved in the oncogenesis of human ovarian cancer using this kind of immortalized OSE cells.
Change history
16 November 2011
This paper was modified 12 months after initial publication to switch to Creative Commons licence terms, as noted at publication
References
Auersperg N, Maines-Bandiera SL, Dyck HG, Kruk PA (1994) Characterization of cultured human ovarian surface epithelial cells: phenotypic plasticity and premalignant changes. Lab Invest 71: 510–518
Chin L, Tam A, Pomerantz J, Wong M, Holash J, Bardeesy N, Shen Q, O'Hagan R, Pantginis J, Zhou H, Horner II JW, Cordon-Cardo C, Yancopoulos GD, DePinho RA (1999) Essential role for oncogenic ras in tumour maintenance. Nature 400: 468–472
Hahn WC, Counter CM, Lundberg AS, Beijersbergen RL, Brooks MW, Weinberg RA (1999) Creation of human tumour cells with defined genetic elements. Nature 400: 464–468
Herbst AL (1994) The epidemiology of ovarian carcinoma and the current status of tumor markers to detect disease. Am J Obstet Gynecol 170: 1099–1105
Kuroda H, Mandai M, Konishi I, Tsuruta Y, Kusakari T, Kariya M, Fujii S (2001) Human ovarian surface epithelial (OSE) cells express LH/hCG receptors, and hCG inhibits apoptosis of OSE cells via up-regulation of insulin-like growth factor-1. Int J Cancer 92: 309–315
Kyo S, Takakura M, Kanaya T, Zhuo W, Fujimoto K, Nishio Y, Orimo A, Inoue M (1999) Estrogen activates telomerase. Cancer Res 59: 5917–5921
Maines-Bandiera SL, Kruk PA, Auersperg N (1992) Simian virus 40-transformed human ovarian surface epithelial cells escape normal growth controls but retain morphogenetic responses to extracellular matrix. Am J Obstet Gynecol 167: 729–735
Matias-Guiu X, Prat J (1998) Molecular pathology of ovarian carcinomas. Virchows Arch 433: 103–111
Nitta M, Katabuchi H, Ohtake H, Tashiro H, Yamaizumi M, Okamura H (2001) Characterization and tumorigenicity of human ovarian surface epithelial cells immortalized by SV40 large T antigen. Gynecol Oncol 81: 10–17
O-charoenrat P, Rhys-Evans P, Modjtahedi H, Eccles SA (2000) Vascular endothelial growth factor family members are differentially regulated by c-erbB signaling in head and neck squamous carcinoma cells. Clin Exp Metast 18: 155–161
Ong A, Maines-Bandiera SL, Roskelley CD, Auersperg N (2000) An ovarian adenocarcinoma line derived from SV40/E-cadherin-transfected normal human ovarian surface epithelium. Int J Cancer 85: 430–437
Scully RE (1977) Ovarian tumors: a review. Am J Pathol 87: 686–720
Tsuruta Y, Mandai M, Konishi I, Kuroda H, Kusakari T, Yura Y, Hamid AA, Tamura I, Kariya M, Fujii S (2001) Combination effect of adenovirus-mediated pro-apoptotic bax gene transfer with cisplatin or paclitaxel treatment in ovarian cancer cell lines. Eur J Cancer 37: 531–541
Van Der Haegen BA, Shay JW (1993) Immortalization of human mammary epithelial cells by SV40 large T-antigen involves a two step mechanism. In vitro Cell Dev Biol 29A: 180–182
Workman P, Twentyman P, Balkwill F, Balmain A, Chaplin D, Double J, Embleton J, Newell D, Raymond R, Stables J, Stephens T, Wallace J (1988) United Kingdom Co-ordinating Committee on Cancer Research (UKCCCR) Guidelines for the Welfare of Animals in Experimental Neoplasia (second edition). Br J Cancer 77: 1–10
Zhang X, Gaspard JP, Chung DC (2001) Regulation of vascular endothelial growth factor by the Wnt and K-ras pathways in colonic neoplasia. Cancer Res 61: 6050–6054
Acknowledgements
We thank Professors Gordon N Gill (University of California, San Diego), F Ishikawa (Tokyo Technology University, Tokyo) and J Fujita (Kyoto University, Kyoto) for their generous gifts of pSV2erbB2, pcDNA3-hTERTn2, pSV3neo and pSNTT. This study was partly supported by Grant-in-Aid for Scientific Research to SF (13307047) from the Ministry of Education and Science, Japan.
Author information
Authors and Affiliations
Corresponding author
Rights and permissions
From twelve months after its original publication, this work is licensed under the Creative Commons Attribution-NonCommercial-Share Alike 3.0 Unported License. To view a copy of this license, visit http://creativecommons.org/licenses/by-nc-sa/3.0/
About this article
Cite this article
Kusakari, T., Kariya, M., Mandai, M. et al. C-erbB-2 or mutant Ha-ras induced malignant transformation of immortalized human ovarian surface epithelial cells in vitro. Br J Cancer 89, 2293–2298 (2003). https://doi.org/10.1038/sj.bjc.6601423
Received:
Revised:
Accepted:
Published:
Issue Date:
DOI: https://doi.org/10.1038/sj.bjc.6601423
Keywords
This article is cited by
-
A prospect of cell immortalization combined with matrix microenvironmental optimization strategy for tissue engineering and regeneration
Cell & Bioscience (2019)
-
Ovarian cancer in endometriosis: molecular biology, pathology, and clinical management
International Journal of Clinical Oncology (2009)
-
Disruption of the retinoblastoma pathway by small interfering RNA and ectopic expression of the catalytic subunit of telomerase lead to immortalization of human ovarian surface epithelial cells
Oncogene (2007)