Abstract
Atopic Dermatitis (AD) has been associated with gut microbiota (GM) dysbiosis in humans, indicating a causative role of GM in AD etiology. Furthermore, the GM strongly correlates to essential disease parameters in the well-known oxazolone-induced mouse model of AD. Here, we demonstrate that it is possible to transfer both a high-responding and a low-responding AD phenotype with GM from conventional mice to germ-free mice. The mice inoculated with the high-responding GM had significantly higher clinical score, increased ear thickness, and increased levels of IL-1β, TNFα, IL-4, IL-5, and IL-6 compared to the mice inoculated with the low-responding GM. The inter-individual variation was in general not affected by this increase in effect size. Germ-free mice induced with AD revealed a high disease response as well as high inter-individual variation indicating protective properties of certain microbial taxa in this model. This study underlines that the GM has a strong impact on AD in mouse models, and that the power of studies may be increased by the application of mice inoculated with a specific GM from high responders to increase the effect size.
Similar content being viewed by others
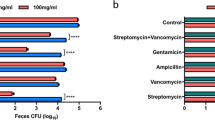
Introduction
Atopic dermatitis (AD) is a common skin disease characterized by inflammatory, noncontagious, pruritic eczema1. AD is driven by contact with environmental allergens, which often in their form of haptens become immunogenic when reacting with host proteins. Pathogenesis includes skin barrier disruption, genetic predisposition2, elevated IgE levels and immune dysregulation characterized by initial T helper cell type 2 (Th2) and T helper cell type 1 (Th1) in the chronic phase3,4,5.
Stress, infections, and exposure to food- and aeroallergens all contribute to the disease6,7. The hygiene hypothesis proposed by Strachan in 1989, postulates that a decreased exposure to bacteria early in life due to increased hygiene in the Western industrialized countries results in impaired immune system activation and regulation leading to higher prevalence of allergies and atopic diseases8,9. This could explain the higher prevalence of AD in Western industrialized countries being more sanitized than developing countries10,11,12.
Human studies have revealed gut microbiota (GM) compositional changes in AD patients compared to healthy controls. In general, patients with AD have a less diverse GM, with a lower abundance of Bifidobacterium spp. while being more frequently colonized with Staphylococcus aureus, certain clostridia, and Escherichia coli13,14,15. The amount of E. coli in the GM further correlates to serum IgE concentrations16. Bifidobacterium spp. has an alleviating effect, showing a positive effect of probiotics in both prevention and treatment of AD17,18,19. GM composition changes are demonstrated early in life prior to any clinical manifestations, indicating that dysbiosis is causative rather than an effect of AD20,21.
A well-known model for AD-like symptoms is the oxazolone-induced mouse, in which multiple epicutaneous administration of oxazolone, a potent hapten, induces a chronic Th2 hypersensitivity reaction resembling the features of initial human AD. Clinically, this model is characterized by a thickened ear/oedema, haemorrhagia, excoriations, and lichenefication22,23. In this model, the GM has shown to strongly correlate with ear tissue levels of IFN-γ, TNF-α, and IL-1024.
Previously, a causal relationship between GM and obesity25, colitis26 and enteric infections27 has been demonstrated by fecal transfer of phenotypes to germ-free mice. However, it has never been demonstrated that sensitivity to chronic oxazolone inflammation, resembling AD, can be transferred with the GM between mice. This is of fundamental interest, as it will indicate a causal role of GM in AD and provide an excellent model for identifying protective or AD enhancing bacteria. Furthermore, from an animal model perspective, effect size and study power may be increased if mice prior to induction are inoculated with a GM yielding a stronger AD resembling phenotype. Finally, it is of interest to know, whether transfer of a GM from a high responder will increase the Th2 response on the cost of a Th1 response, thereby making the oxazolone model more translational to the initial Th2 dominated response in human AD28.
We, therefore, hypothesized that both a high and a low responding oxazolone phenotype can be transferred from conventional mice with the GM to germ-free mice, and that germ-free mice differ in disease sensitivity from conventional mice.
Material and Methods
Ethics
The experiments were carried out in accordance with the Danish Act on Animal experimentation (LBK nr 474 of 15/05/2014), which implements the directive 2010/63/EU on the protection of animals used for scientific purposes. The study was approved by the Animal Experimentation Inspectorate, Ministry of Environment and Food, Denmark (license No. 2012-15-2934-00399) and health monitoring was performed according to FELASA guidelines29.
Animals
C57BL/6Ntac mice (n = 16, 7wks) (Taconic, Lille Skensved, Denmark) allowed to acclimate for 1 week were housed in our AAALAC accredited barrier protected facility (Faculty of Health and Medical Sciences, University of Copenhagen, Frederiksberg, Denmark) in open cages without filter lids with free access to an Altromin 1324 diet (Brogaarden, Lynge, Denmark) and tap water.
Nine germ-free C57BL/6Ntac (6 females, 3 males, 7wks) (Taconic, Germantown, NY) were housed in our AAALAC accredited germ-free facility (Faculty of Health and Medical Sciences, University of Copenhagen, Frederiksberg, Denmark) in HEPA-ventilated isolators (PFI systems, Milton Keynes, UK) (pressure 110 pascal, 23 °C) with free access to an irradiated Altromin 1324 diet (Brogaarden) and sterile water. Germ-free status was tested both by culturing and PCR methods30. DNA extractions was made with the mobio Powerlyzer Powersoil DNA isolation kit (Mo bio laboratories, inc., Carlsbad, CA) following manufactures instructions.
First, dermatitis was induced on the ears of all the barrier housed (CONV) mice. Based upon an overall evaluation of the dermatitis score, ear thickness, and concentrations of IFN-γ, IL-10, IL-1β, IL-2, IL-5, IL-6, and TNF-α, a high and low-responding donor were selected (Table S1). Next, feces from the donors was suspended in phosphate-buffered saline and given by oral gavage to pregnant germ-free dams in two different isolators, and subsequently the off-spring of these (n = 13) (HIGH and LOW mice) as well as germ-free off-spring (n = 12) in a third isolator was used for induction of dermatitis as done for the CONV group (Fig. 1).
Germ-free C57BL/6 dams in three different isolators were inoculated with fecal gut microbiota from the lowest responding conventional mouse (LOW), the highest responding conventional mouse (HIGH) or not inoculated at all. The off-spring of the inoculated dams develops oxazolone induced dermatitis on the ears at the age of eight weeks.
Induction of skin inflammation
All mice (8wks), were sensitized once with 0.8% (w/v) 4-ethoxymethylene-2-phenyl-2-oxazolin-5-one (Oxazolone)(Sigma-Aldrich, St. Louis, MO, USA) dissolved 4:1 in acetone (Emsure, MerckChemicals, Germany) and olive oil (Organic extra virgin olive oil, Svansø, Denmark) with a pipette on both sides of the right ear. After one week the mice were challenged on days 0, 3, 7, 10, 12, 14, 16, 18 and 20 with a fresh solution of 0.4% (w/v) oxazolone dissolved in acetone and olive oil 4:1 on both sides of the right ear. All mice were sacrificed and sampled on day 21. The dosage of oxazolone was based upon an in-house pilot study as well as a previous study showing correlations between the GM and ear tissue concentrations of inflammatory cytokines in the oxazolone model24.
Clinical Scores
At euthanasia the inflamed ear was macroscopically scored blindly by two independent persons for each of the signs 1) flare haemorrhage, 2) oedema, 3) excoriation and erosion, and 4) incrustation and xerosis as follows: 0 = no sign; 1 = mild; 2 = moderate; or 3 = severe31 adding the sum of the mean between the two scores as the total dermatitis score. Ear thickness was recorded on day 21 by using a micrometer (Mitutoyo Low Force Caliper Series 573, Aurora, Illinois); each measurement was repeated twice and the calculated mean used. The same person performed all measurements to ensure similar pressure and placement of the micrometer.
Serum IgE
Orbital blood was collected terminally under anesthesia with 25% Hypnorm (Fentanyl/Fluanison, Vetapharma, Leeds, UK) and 25% Midazolam Hameln (5 mg/ml, Matrix Pharmaceuticals, Hellerup, Denmark) 0.1 ml pr. 10 g BW. Serum IgE concentration was determined in a 1:20 dilution with the mouse IgE ELISA kit from Bethyl Laboratories (Montgomery, TX), following manufacturer’s instructions.
Ear tissue cytokines
An 8 mm biopsy punch (Kruuse, Langeskov, Denmark) from the inflamed ear was frozen in liquid nitrogen and stored at −80 degrees. The day of analysis, the ear tissue was thawed on ice and homogenized in 300 μl lysis buffer (stock solution contained 10 ml Tris lysis buffer (Mesoscale Discovery, Rockville, MD), 100 μl phosphatase inhibitor 1, 100 μl phosphatase inhibitor 2 and 200 μl protease inhibitor (MSD inhibitor pack, Mesoscale Discovery)) using a tissue blender (Polytron® PT 1200 E, Kinematica AG, Lucerne, Switzerland). The homogenized tissue was centrifuged (7500 g; 5 min) and the supernatant used for analyzing the amount of IFNγ, IL1β, IL2, IL4, IL5, IL6, keratinocyte-derived chemokine/growth-related oncogene (KC/GRO), IL10, IL12p70, and TNFα (Proinflammatory Panel 1 (mouse) kits, Vplex (Mesocale Discovery)) and IL-13 (mouse IL-13 assay ultra-sensitive kit (Mesoscale Discovery)) according to manufactures instructions. Results were read on a MESO QuickPlex SQ 120 (Mesoscale Discovery).
High throughput sequencing of the gut microbiota
The fecal microbiota of all groups were determined using tag-encoded 16S rRNA gene (V3-V4-region) MiSeq-based (2 × 250 PE) (Illumina, San Diego, CA) high throughput sequencing. DNA extraction was made with mobio Powerlyzer Powersoil DNA isolation kit (Mo bio Laboratories). DNA storage conditions, sequencing library preparation and sequencing steps were conducted as previously described30.
The raw dataset containing pair-ended reads with corresponding quality scores were merged and trimmed using fastq_mergepairs and fastq_filter scripts implemented in the UPARSE pipeline setting minimum overlap length to 10 bp. The minimum length of merged reads was 250 bp. The max expected error E = 2.0, and first truncating position with quality score N ≤ 4. Dataset from chimeric reads purging and constructing de novo Operational Taxonomic Units (OTU) were conducted UPARSE pipeline32. The green genes (13.8) 16S rRNA gene collection was used as a reference database. Quantitative Insight Into Microbial Ecology (QIIME) open source software package (1.7.0, 1.8.0 and 1.9.1)33,34 was used for analysis.
Alpha and beta diversity analysis was performed as previously described using iterative subsampling (36,000 reads/sample)35. Permanova (compare_categories.py, Qiime 1.8.0) and PermanovaG (the Generalized UniFrac R package; GUniFrac)36 were used to evaluate group differences using weighted, unweighted and generalized uniFrac37 distance matrices respectively. All distance matrices were generated based on rarefied (36,000 reads/sample) OTU tables. The relative distribution of the genera registered was calculated for unified and summarized in the genus level OTU tables.
The G test of independence (q_test) and ANOVA determined respectively: qualitative (presence/absence) and quantitative (relative abundance) association of OTUs with given category. Parametric student’s t-test incorporated in group_significance script (QIIME v9.1) was used to test frequencies of species level OTUs between the “high” and the “low” categories. The probability (p-value) was calculated based on random subsampled OTU tables rarefied to 36,000 per sample.
GM correlation analysis
Correlations between disease parameters and genera relative abundance were verified with the Pearson’s product-moment correlation coefficient (QIIME 1.7.0), based on 1000 rarefied OTU tables unified to an equal number of reads per sample (36,000). Correlations still significant after randomly removing three subsequent observations were considered valid.
Statistics
GraphPad Prism version 6.03 (GraphPad Software, San Diego, CA) was used for statistical analysis, and p values less than 0.05 were considered significant. Differences were estimated by one-way ANOVA test with post hoc t-tests for multiple testing or by Kruskal-Wallis test with post hoc Mann-Whitney U-test for multiple testing for data that either did not follow Gaussian distributions or did not have equal variances in the groups. Brown-Forsythe’s test was used to compare differences in variances and ranges. Regressions on the correlation between different parameters, the GM excluded, were made as fitted line plots in Minitab 17 (Minitab Ltd., Coventry, UK).
Results
In this study, we tried to transfer sensitivity to oxazolone induced dermatitis with the GM from a high and low responding donor to two groups of germfree mice. A third group of mice was kept germfree to investigate the role of bacteria in oxazolone induced dermatitis.
Immunological parameters
We found that the most relevant cytokines for the oxazolone model were IL-1β, TNFα, IL-4, and IL-6, which shared more than 25% of their variation with the variation in ear thickness in all dermatitis induced mice (Fig. 2). For all of these cytokines, as well as IL-5, the HIGH mice had a higher level in ear tissue than the LOW mice. IL-1β, TNFα, IL-6 and KC/GRO were also higher in HIGH mice compared to the CONV mice, while all germfree derived mice (HIGH, LOW and germfree) had lower concentrations of IL-12p70 compared to the CONV mice (Fig. 2). Data from IL-13 are not shown due to concentrations under detection level of the assay.
Graphs illustrate cytokine responses (pg/ml) with SD in ear tissue from mice with oxazolone induced dermatitis. CONV: Mice randomly selected from a conventionally barrier-protected colony. CONV mouse marked with ★ = Donor HIGH. CONV mouse marked with X = Donor LOW. LOW: Ex-germ-free mice inoculated with the gut microbiota from the lowest responding CONV mouse; HIGH: Ex-germ-free mice inoculated with the gut microbiota from the highest responding CONV mouse; Germfree: Germ-free mice. Highlights reflects the correlation to the clinical score ‘Ear Thickness’ (ET) with the adjusted correlation coefficient (cc) stated with the significance level, i.e. red highlight: cc > 35%; yellow highlight: cc > 25%; green highlight; cc ≥ 15%; blue highlight: cc < 15%; no highlight: not significant. Significance lines indicate differences between means of all groups compared by ANOVA with subsequent t-tests for data with equal variances and by Kruskall-Wallis and Mann-Whitney test for data not showing equal variances. Data from IL-13 is not shown due to concentrations under detection level of the assay. *p < 0.05; **p < 0.01; ***p < 0.001; ****p < 0.0001.
Generally, the CONV mice had the lowest variation (TNF-α, KC/GRO) and the germ-free mice had the highest variation (IL-1β, IL-4, IL-6). The inter-individual variation was not lowered in the HIGH and LOW mice inoculated with a uniform GM (Table S2).
Clinical parameters and serum IgE concentration
The LOW mice had lower dermatitis score compared to all other groups which did not differ in dermatitis score (Fig. 3A). There were no significant differences in the size of the ranges between the groups (Table S2). The HIGH mice had thicker and the LOW mice had thinner ears compared to both CONV and germ-free mice (Fig. 3B). The germ-free mice expressed a higher variation in ear thickness compared to the other groups (Table S2).
Graphs illustrate median and range for Total dermatitis score (A) and mean and standard deviation for ear thickness (B; mm) from ears as well as serum IgE (C; ng/ml) of mice with oxazolone induced dermatitis. CONV: Mice randomly selected from a conventionally barrier-protected colony. CONV mouse marked with ★ = Donor HIGH. CONV mouse marked with X = Donor LOW; LOW: Ex-germ-free mice inoculated with the gut microbiota from the lowest responding CONV mouse; HIGH: Ex-germ-free mice inoculated with the gut microbiota from the highest responding CONV mouse; Germfree: Germ-free mice. Significant lines indicate differences between means of all groups compared by ANOVA with subsequent t-tests for data with equal variances and by Kruskall-Wallis and Mann-Whitney test for data not showing equal variances. Differences in dermatitis score (A) is calculated by Kruskall-Wallis and Mann-Whitney test. *p < 0.05; **p < 0.01; ***p < 0.001; ****p < 0.0001.
Germ-free mice had significantly higher levels and ranges of serum IgE compared to the other groups, in which no differences were evident (Fig. 3C and Table S2).
Gut microbiota upon transfer to germ-free mice
Sequences purged from chimeric reads yielded 3,571,114 giving an average of 101,998 sequences per sample (min = 42,442; max = 223,427; SD = 43,185 with a mean sequence length of 451 bp (±25 bp). Five samples were discarded due to low read number (<300 reads). GM transfer resulted in two new GM profiles clearly different from each other and from the conventional mice (Fig. 4A,B and C). The CONV and HIGH mice had comparable alpha diversity indexes, while the LOW mice had significantly lower diversity compared to CONV and HIGH according to observed species and chao1 indexes (p < 0.01), while the LOW Shannon diversity index was only significantly lower compared to CONV (p < 0.01)(Fig. 5). This difference was mostly due to the LOW mice missing a number of Clostridiales assigned OTUs. The HIGH mice did not harbor the specific murine bacterium Mucispirillum schaedleri (Table 1).
Projection of differences in the gut microbiota composition between mice with oxazolone induced dermatitis. CONV: Mice randomly selected from a conventionally barrier-protected colony; LOW: Ex-germ-free mice inoculated with the gut microbiota from the lowest responding SPF mouse (marked with a green circle); HIGH: Ex-germ-free mice inoculated with the gut microbiota from the highest responding CONV mouse (marked with a green square); Germfree: Germ-free mice. PCoA plots based on unweighted (A), weighted (B) and generalised UniFrac distance matrices calculated from 10 rarefied (36,000 reads/sample) OTU tables. Group differences between the three categories were based on PermanovaG.
Rarefaction curves based on observed species index (97% sequence similarity threshold), Chao1 and Shannons diversity index. Alpha diversity measure expressed with observed species and chao1 indexes revealed significantly reduced (p < 0.01) diversity in ex-germ-free mice inoculated with the gut microbiota from the lowest responding CONV mouse (LOW) compared to ex-germ-free mice inoculated with the gut microbiota from the highest responding CONV mouse (HIGH) as well as to barrier protected mice (CONV). Shannon diversity index only revealed a reduced (p < 0.01) diversity in LOW mice compared to CONV mice. **p < 0.01.
The community level differences in relative abundance between CONV, HIGH and LOW groups (Fig. 4B) were also reflected in the relative abundance of several OTUs being significantly different between the groups. Noticeable differences are that the HIGH and LOW mice had a lower abundance of unclassified species belonging to the families of Bacteroidales S24-7 and Lachnospiraceae and higher abundance of Lactobacillus spp. compared to the CONV mice (Table 2A). When comparing the GM of LOW and HIGH mice alone, the GM of HIGH mice was to a higher degree dominated by species belonging to the family of Lachnospiraceae, as well as Bacteroides uniformis and an unclassified genus of Rikenellaceae (Table 2B). In contrast, the LOW mice tended to have more unclassified Bacteroides spp. compared to the HIGH mice (p = 0.008, FDR = 0.059).
These differences evident between the inoculated groups were not present in the donor mice, in which there was no difference in the occurrence or abundance of Lachnospiraceae spp., Bacteroides spp., Rikenellaceae spp. or M. schaedleri (Table S3). The LOW donor seemed to have a lower abundance of Lactobacillus compared to the HIGH donor. This was opposite in the colonized groups in which the LOW mice tended to have more Lactobacillus than the HIGH mice. However, a pronounced abundance of Lactobacillus is evident in both the colonized groups (Table 2A).
The relative abundance of several species correlated with various clinical and immunological parameters. Even after random removal of a data point three times, significant correlations, mostly within the phylum Firmicutes, were found (Figure S1). Most strikingly, ear thickness in CONV mice showed a strong correlation to Mollicutes spp., Enterococcus spp., and Mucispirillum spp.
Discussion
The HIGH and the LOW phenotypes from the conventional mice were clearly transferred to germ-free mice, as there was a higher expression of dermatitis score, ear thickness, IL-1β, TNFα, IL-4, and IL-6 in the HIGH mice compared to the LOW mice, and for most of these also higher than the CONV mice. GM transfer in general had very little impact on inter-individual variation within the groups in this model. However, the germ-free mice had the highest variation in ear thickness, IgE, IL-1β, TNFα, IL-4, and IL-6, so most likely GM secures a uniform response in these parameters. In study designs, group sizes may be reduced by using tailor-made mice inoculated with a high responder GM due to the increased clinical effect size.
The strong correlation between macrophage produced cytokines IL-1 β38, TNFα39, and IL-640 and ear thickness indicate a GM impact on macrophage activity with subsequent impact on inflammation and T-cell proliferation. The IL-1β augmented TNFα signaling due to up-regulation of TNFα secretion and surface receptor expression in macrophages is triggered by Gram negative bacterial wall lipopolysaccharides (LPS)41, and is an important part of antimicrobial immunity42. IL-4 from Th2 cells43 induce IgE isotype switch in B-cells44, which is closely linked to atopic allergy diseases45,46. GM transfer therefore seems to increase T cell responses in the HIGH mice, and their increased level of the Th2 cytokine IL-543 indicates a higher Th2 response. Moreover, IL-1β, highly correlating with ear thickness, promotes the formation of IL-17 producing Th17 cells47; a key element in the Th2 activation in AD in mice48. The low level of IL12p70 in GM transplanted mice indicates that the role of Th1 cells was decreased by GM transfer. IgE responses did not seem to differ between neither the mice with different GM, nor in the donor mice. Interestingly, germ-free mice had a much higher and more varying IgE production compared to all colonized mice strongly supporting the protective role of bacteria in type 1 hypersensitivity reactions known from other murine allergy models49,50.
Neither the GM of HIGH, nor LOW mice clustered with their donors. GM transfer dramatically increased the relative abundance of Firmicutes; primarily due to an increase in Lactobacillus spp. Previously, we found that when germ-free mice were inoculated at weaning they clustered with the inoculum later in life, but we also showed that timing of inoculation is important for transferring GM profiles51. It is, however, likely that there will be changes in the GM, when the mothers are inoculated, when the offspring are born, and over time from birth to termination that could explain why clustering is not observed between inoculum and recipients. Further, it cannot be ruled out that short exposure to oxygen during transfer, influences the inoculum giving oxygen-tolerant species such as Lactobacillus spp. a competitive advantage when the GM of the inoculated mice is established.
Remarkably, germ-free mice responded highly in key parameters, such as total dermatitis score, ear thickness, TNFα, IL-4, and IL-5. As the LOW mice had the lowest GM diversity compared to HIGH and CONV mice, the high response of the germ-free mice indicates a GM impact on AD in mice to be a question of the presence of protective bacteria rather than a question of diversity. Bacteria present in the LOW mice in contrast to the HIGH mice (Table 1) were M. schaedleri, Blautia producta and an unclassified species of Erysipelotrichaceae. None of these are known for specific protecting roles against AD. M. schaedleri of the Deferribacteres phylum is not found in the human gut, but it may in mice have abundance up to 1%, and it has been used for decades as a member of the altered Schaedler flora (ASF 457)52,53,54. However, in the CONV mice there was a strong positive correlation between the abundance of M. schaedleri and ear thickness, so it is not the most likely AD-protecting candidate.
The two colonized groups differed in the relative abundance of a range of bacteria. For example, Lachnospiraceae spp. was more abundant in the HIGH mice, which may increase AD response, as children suffering from AD tend to have a higher abundance of Clostridia14,21,55. On the other hand, LOW mice showed a tendency to have a higher abundance of Lactobacillus spp., which was only significant if a general outlier in the LOW group was removed (p = 0.039 with outlier removed), and Lactobacillus spp. may have a possible protective effect against AD, as previous studies have shown that different species of dietary Lactobacillus may relieve AD symptoms in murine models56,57,58,59,60.
LOW mice compared to HIGH mice showed a tendency (p = 0.008, FDR-corrected p = 0.059) for higher abundance of the genus Bacteroides, which may have a preventive effect, as a previous study has shown that Bacteroides fragilis is anti-inflammatory and contributes to development of host immunity61. Further, monocolonization with B. fragillis lowered the amount of IL-4 production from CD4+ T cells in germ-free mice62. Therefore, the lower concentration of IL-4 in the LOW mice could be mediated by the higher abundance of Bacteroides. Compared to the LOW mice, HIGH mice showed a greater abundance of another Bacteroides fraction identified as Bacteroides uniformis. Administration of B. uniformis has been shown to ameliorate obesity and the associated immune alteration63. Obesity is Th1 dominated64, in contrast to the Th2 dominated AD3,4,5. Consequently, the higher abundance of B. uniformis in the HIGH mice may alter the Th1/Th2 balance, thereby contributing to Th2 predominance in the HIGH mice.
This study shows that the expression of oxazolone induced dermatitis in mice can be influenced solely by GM interventions. As mentioned in the introduction, patients with AD have compositional changes in their GM13,14,15,16,21, which indicates that GM intervention as treatment or prevention strategy could have an alleviating effect on AD. GM intervention would be a good alternative to the present treatment of AD, which now comprises mainly of topical corticosteroids, which can cause adverse skin side effects65.
The diet has been shown to be an important driver of GM composition and function66,67,68, so a dietary intervention could be a possible and easy accessible approach to change the GM in patients with AD, resembling that of healthy individuals. A more complicated, yet possible, method is fecal transplantation from healthy individuals to patients with AD. Fecal transplantation has recently shown to be effective against other GM associated diseases e.g. Inflammatory Bowel Disease69,70,71 and Clostridium difficile infection72, so it is not unlikely that it could influence the expression of AD. The effect of fecal transplantation may decrease over time in a certain fraction of the patients, e.g. as observed for ulcerative colitis73, but since AD is considered a childhood disease, where symptoms often diminish in adulthood, this will most likely be a minor problem. Before fecal transplantation can be an option for treatment of AD, it would require much more research to identify biomarkers and protective bacteria to find the optimal donors.
In conclusion, a high and a low responding phenotype of AD can be transferred with the GM to germ-free mice. The use of a high responding donor improves the applicability of the inoculated mice as models, because they develop increased ear thickness, higher IL-1β, and TNFα concentrations in ear tissue, as well as a more Th2 dominated response.
Additional Information
How to cite this article: Zachariassen, L. F. et al. Sensitivity to oxazolone induced dermatitis is transferable with gut microbiota in mice. Sci. Rep. 7, 44385; doi: 10.1038/srep44385 (2017).
Publisher's note: Springer Nature remains neutral with regard to jurisdictional claims in published maps and institutional affiliations.
References
De Benedetto, A., Agnihothri, R., McGirt, L. Y., Bankova, L. G. & Beck, L. A. Atopic dermatitis: a disease caused by innate immune defects? J Invest Dermatol 129, 14–30, doi: 10.1038/jid.2008.259 (2009).
Coleman, R., Trembeth, R. C. & Harper, J. I. Genetic studies of atopy and atopic dermatitis. British Journal of Dermatology 1–5 (1997).
Bieber, T. Atopic Dermatitis. Ann Dermatol 125–137 (2010).
Zeller, S., Rhyner, C., Meyer, N., Schmid-Grendelmeier, P., Akdis, C. A. & Crameri R. Exploring the repertoire of IgE-binding self-antigens associated with atopic eczema. The Journal of Allergy and Clinical Immunology 278–285 (2009).
Novak, N. & Bieber, T. The role of dendritic cell subtypes in the pathophysiology of atopic dermatiits. J am acad dermatol 171–176 (2005).
Sabounchi, S., Bollyky, J. & Nadeau, K. Review of Environmental Impact on the Epigenetic Regulation of Atopic Diseases. Current allergy and asthma reports 15, 33, doi: 10.1007/s11882-015-0533-1 (2015).
Kim, K. Influences of Environmental Chemicals on Atopic Dermatitis. Toxicological research 31, 89–96, doi: 10.5487/tr.2015.31.2.089 (2015).
Williams, H. & Flohr, C. How epidemiology has challenged 3 prevailling concepts about atopic dermatitis. Journal of Allergy and Clinical Immunology 209–213 (2006).
Strachan, D. Hay fever, hygiene, and household size. British Medical Journal 1259–1260 (1989).
Boguniewicz, M. & Leung, D. Recent insights into atopic dermatitis and implications for management of infectious complications. Journal of Allergy and Clinical Immunology 4–13 (2010).
Williams, H., Stewart, A., von Mutius, E., Cookson, W. & Anderson, H. R. Is eczema really on the increase worldwide? Journal of Allergy and Clinical Immunology 947–954 (2008).
Williams, H. et al. Worldwide variations in the prevalence of symptoms of atopic eczema in the International Study of Asthma and Allergies in Childhood. J Allergy Clin Immunol 125–138 (1999).
Björkstén, B., Naaber, P., Sepp, E. & Mikelsaar, M. The intestinal microflora in allergic Estonian and Swedish 2-year-old children. Clinical and Experimental Allergy 342–346 (1999).
Björkstén, B., Sepp, E., Julge, K., Voor, T. & Mikelsaar, M. Allergy development and the intestinal microflora during the first year of life. Journal of Allergy and Clinical Immunology 516–520 (2001).
Watanabe, S. et al. Differences in fecal microflora between patients with atopic dermatitis and healthy control subjects. J Allergy Clin Immunol 587–591 (2003).
Kirjavainen, P. V., Arvola, T., Salminen, S. J. & Isolauri, E. Aberrant composition of gut microbiota of allergic infants: a target of bifidobacterial therapy at weaning. Gut 51–55 (2002).
Dotterud, C. K., Storrø, O., Johnsen, R. & Oien, T. Probiotics in pregnant women to prevent allergic disease: a randomized, double-blind trial. Br. J. Dermatol 616–623 (2010).
Huurre, A., Laitinen, K., Rautava, S., Korkeamäki, M. & Isolauri, E. Impact of maternal atopy anf probiotic supplementation during pregnancy on infant sensitization: a double-blind placebo-controlled study. Clin. Exp. Allergy 1342–1348 (2008).
West, C. E., Hammarström, M. L. & Hernell, O. Probiotics during weaning reduce incidence of eczema. Pediatr. Allergy Immunol. 430–437 (2009).
Mah, K. et al. Distinct pattern of commensal gut microbiota in toddlers with eczema. International archives of allergy and immunology 140, 157–163, doi: 10.1159/000092555 (2006).
Kalliomäki, M., Kirjavainen, P., Eerola, E., Kero, P., Salminen, S. & Isolaur, E. Distinct patterns of neonatal gut microflora in infants in whom atopy was and was not developing. The Journal of allergy and clinical immunology 107, 129–134, doi: 10.1067/mai.2001.111237 (2001).
Mao-Qiang, M. et al. Characterization of a hapten-induced, murine model with multiple features of atopic dermatitis: structural, immunologic, and biochemical changes following single versus multiple oxazolone challenges. Journal of Investigative Dermatology 79–86 (2008).
Jin, H., He, R., Oyoshi, M. & Geha, R. S. Animal models of atopic dermatitis. J.Invest Dermatol. 129, 31–40, doi: jid2008106 [pii];10.1038/jid.2008.106 [doi] (2009).
Lundberg, R. et al. Gastrointestinal Microbiota and local inflammation during oxazolone-induced dermatitis in BALB/cA mice. Comparative Medicine 62, 371–380 (2012).
Turnbaugh, P., Ley, R., Mahowald, M., Magrini, V., Mardis, E. & Gordon, J. An obesity-associated gut microbiome with increased capacity for energy harvest. Nature 444, 1027- 1031 (2006).
Grimm, V., Radulovic, K. & Riedel, C. U. Colonization of C57BL/6 Mice by a Potential Probiotic Bifidobacterium bifidum Strain under Germ-Free and Specific Pathogen-Free Conditions and during Experimental Colitis. PLoS One 10, e0139935, doi: 10.1371/journal.pone.0139935 (2015).
Kubinak, J. et al. MHC variation sculpts individualized microbial communities that control susceptibility to enteric infection. Nature communications 6, 8642, doi: 10.1038/ncomms9642 (2015).
Wang, A. X. & Xu Landen, N. New insights into T cells and their signature cytokines in atopic dermatitis. IUBMB life 67, 601–610, doi: 10.1002/iub.1405 (2015).
Mähler, M. et al. FELASA working group on revision of guidelines for health monitoring of rodents and rabbits: FELASA recommendations for the health monitoring of mouse, rat, hamster, guinea pig and rabbit colonies in breeding and experimental units. Laboratory Animals, doi: 10.1177/0023677213516312 (2014).
Jørgensen, B. et al. A possible link between food and mood: dietary impact on gut microbiota and behavior in BALB/c mice. PLoS One 9, e103398, doi: 10.1371/journal.pone.0103398 (2014).
Ohmura, T. et al. Suppression of atopic-like dermatitis by treatment with antibody to lymphocyte function-associated antigen-1 in NC/Nga mouse. European journal of pharmacology 504, 113–117, doi: 10.1016/j.ejphar.2004.09.035 (2004).
Edgar, R. C. UPARSE: highly accurate OTU sequences from microbial amplicon reads. Nature methods 10, 996–998, doi: 10.1038/nmeth.2604 (2013).
McDonald, D. et al. An improved Greengenes taxonomy with explicit ranks for ecological and evolutionary analyses of bacteria and archaea. The ISME journal 6, 610–618, doi: 10.1038/ismej.2011.139 (2012).
Caporaso, J. et al. QIIME allows analysis of high-throughput community sequencing data. Nature methods 7, 335–336, doi: 10.1038/nmeth.f. 303 (2010).
Krych, L., Hansen, C. H. F., Hansen, A. K., van den Berg, F. W. & Nielsen, D. S. Quantitatively Different, yet Qualitatively Alike: A Meta-Analysis of the Mouse Core Gut Microbiome with a View towards the Human Gut Microbiome. PLoS One 8, e62578, doi: 10.1371/journal.pone.0062578 (2013).
Chen, J. et al. Associating microbiome composition with environmental covariates using generalized UniFrac distances. Bioinformatics (Oxford, England) 28, 2106–2113, doi: 10.1093/bioinformatics/bts342 (2012).
Lozupone, C. & Knight, R. UniFrac: a new phylogenetic method for comparing microbial communities. Applied and environmental microbiology 71, 8228–8235, doi: 10.1128/aem.71.12.8228-8235.2005 (2005).
Auron, P. et al. Nucleotide sequence of human monocyte interleukin 1 precursor cDNA. Proc Natl Acad Sci USA 81, 7907–7911 (1984).
Olszewski, M. B., Groot, A. J., Dastych, J. & Knol, E. F. TNF trafficking to human mast cell granules: mature chain-dependent endocytosis. J Immunol 178, 5701–5709 (2007).
Natsuka, S. et al. Macrophage differentiation-specific expression of NF-IL6, a transcription factor for interleukin-6. Blood 79, 460–466 (1992).
Walsh, L. J., Trinchieri, G., Waldorf, H. A., Whitaker, D. & Murphy, G. F. Human dermal mast cells contain and release tumor necrosis factor alpha, which induces endothelial leukocyte adhesion molecule 1. Proc Natl Acad Sci USA 88, 4220–4224 (1991).
Jayaraman, P. et al. IL-1 beta Promotes Antimicrobial Immunity in Macrophages by Regulating TNFR Signaling and Caspase-3 Activation. Journal of Immunology 190, 4196–4204, doi: 10.4049/jimmunol.1202688 (2013).
Mosmann, A. T. R. & Coffman, R. L. TH1 and TH2 Cells: Different Patterns of Lymphokine Secretion Lead to Different Functional Properties. Annual Review of Immunology 7, 145–173, doi: doi: 10.1146/annurev.iy.07.040189.001045 (1989).
de Vries, J. E., Punnonen, J., Cocks, B. G., Malefyt, R. D. W. & Aversa, G. Regulation of the human IgE response by IL4 and IL13. Research in Immunology 144, 597–601, 10.1016/S0923-2494(05)80009-4 (1993).
Hershey, G. K., Friedrich, M. F., Esswein, L. A., Thomas, M. L. & Chatila, T. A. The association of atopy with a gain-of-function mutation in the alpha subunit of the interleukin-4 receptor. The New England journal of medicine 337, 1720–1725, doi: 10.1056/nejm199712113372403 (1997).
Di Lernia, V. Therapeutic strategies in extrinsic atopic dermatitis: focus on inhibition of IL-4 as a new pharmacological approach. Expert opinion on therapeutic targets 19, 87–96, doi: 10.1517/14728222.2014.965682 (2015).
Song, L. et al. Th17 can regulate silica-induced lung inflammation through an IL-1beta-dependent mechanism. Journal of cellular and molecular medicine 18, 1773–1784, doi: 10.1111/jcmm.12341 (2014).
Nakajima, S. et al. IL-17A as an inducer for Th2 immune responses in murine atopic dermatitis models. J Invest Dermatol 134, 2122–2130, doi: 10.1038/jid.2014.51 (2014).
Yu, W. et al. Reduced airway microbiota diversity is associated with elevated allergic respiratory inflammation. Annals of allergy, asthma & immunology: official publication of the American College of Allergy, Asthma, & Immunology 115, 63–68, doi: 10.1016/j.anai.2015.04.025 (2015).
Herbst, T. et al. Dysregulation of allergic airway inflammation in the absence of microbial colonization. Am J Respir Crit Care Med 184, 198–205, doi: 10.1164/rccm.201010-1574OC (2011).
Hansen, C. F. H. et al. Patterns of early gut colonization shape future immune responses of the host. PLoS One, 7(3), e34043. doi: 10.1371/journal.pone.0034043 (2012).
Robertson, B. et al. Mucispirillum schaedleri gen. nov., sp. nov., a spiral-shaped bacterium colonizing the mucus layer of the gastrointestinal tract of laboratory rodents. Int.J.Syst.Evol.Microbiol. 55, 1199–1204, doi: 55/3/1199 [pii];10.1099/ijs.0.63472-0 [doi] (2005).
Dewhirst, F. et al. Phylogeny of the defined murine microbiota: altered Schaedler flora. Appl.Environ.Microbiol. 65, 3287–3292 (1999).
Schaedler, R. W., DUBS, R. & Costello, R. Association of germfree mice with bacteria isolated from normal mice. J.Exp.Med. 122, 77–82 (1965).
Sepp, E., Julge, K., Mikelsaar, M. & Bjorksten, B. Intestinal microbiota and immunoglobulin E responses in 5-year-old Estonian children. Clin Exp Allergy 35, 1141–1146, doi: 10.1111/j.1365-2222.2005.02315.x (2005).
Yeom, M. et al. Oral administration of Lactobacillus casei variety rhamnosus partially alleviates TMA-induced atopic dermatitis in mice through improving intestinal microbiota. Journal of applied microbiology 119, 560–570, doi: 10.1111/jam.12844 (2015).
Inoue, N. A., Fukushima, Y. & Ushida, K. Oral treatment with probiotic Lactobacillus johnsonii NCC533 (La1) for a specific part of the weaning period prevents the development of atopic dermatitis induced after maturation in model mice, NC/Nga. Br J Dermatol 156, 499–509 (2007).
Tanaka, J. K. et al. Oral supplementation with Lactobacillus rhamnosus CGMCC 1.3724 prevents development of atopic dermatitis in NC/NgaTnd mice possibly by modulating local production of IFN-gamma. Exp Dermatol 18, 1022–1027 (2009).
Shah, M. et al. Lactobacillus acidophilus strain L-92 induces CD4(+)CD25(+)Foxp3(+) regulatory T cells and suppresses allergic contact dermatitis. Biol Pharm Bull 35, 612–616 (2012).
Chapat, L. C. K., Dubois, B., Bourdet-Sicard, R. & Kaiserlian, D. Lactobacillus casei reduces CD8+ T cell-mediated skin inflammation. Eur J Immunol 34, 2520–2528 (2004).
Mazmanian, S. K., Round, J. L. & Kasper, D. L. A. Microbial symbiosis factor prevents intestinal inflammatory disease. Nature 453, 620–625, doi: 10.1038/nature07008 (2008).
Mazmanian, S. K., Liu, C. H., Tzianabos, A. O. & Kasper, D. L. An immunomodulatory molecule of symbiotic bacteria directs maturation of the host immune system. Cell 122, 107–118, doi: 10.1016/j.cell.2005.05.007 (2005).
Gauffin Cano, P., Santacruz, A., Moya, A. & Sanz, Y. Bacteroides uniformis CECT 7771 ameliorates metabolic and immunological dysfunction in mice with high-fat-diet induced obesity. PLoS One 7, e41079, doi: 10.1371/journal.pone.0041079 (2012).
Harford, K. A., Reynolds, C. M., McGillicuddy, F. C. & Roche, H. M. Fats, inflammation and insulin resistance: insights to the role of macrophage and T-cell accumulation in adipose tissue. The Proceedings of the Nutrition Society 70, 408–417, doi: 10.1017/s0029665111000565 (2011).
Mehta, A. B. et al. Topical corticosteroids in dermatology. Indian journal of dermatology, venereology and leprology 82, 371–378, doi: 10.4103/0378-6323.178903 (2016).
Sheflin, A. M., Melby, C. L., Carbonero, F. & Weir, T. L. Linking Dietary Patterns with Gut Microbial Composition and Function. Gut Microbes 0, doi: 10.1080/19490976.2016.1270809 (2016).
David, L. A. et al. Diet rapidly and reproducibly alters the human gut microbiome. Nature 505, 559–563, doi: 10.1038/nature12820 (2014).
Wu, G. D. et al. Linking long-term dietary patterns with gut microbial enterotypes. Science 334, 105–108, doi: 10.1126/science.1208344 (2011).
Wei, Y. et al. Fecal Microbiota Transplantation Improves the Quality of Life in Patients with Inflammatory Bowel Disease. Gastroenterology research and practice 2015, 517597, doi: 10.1155/2015/517597 (2015).
Aroniadis, O. C. & Brandt, L. J. Fecal microbiota transplantation: past, present and future. Current opinion in gastroenterology 29, 79–84, doi: 10.1097/MOG.0b013e32835a4b3e (2013).
Moayyedi, P. et al. Fecal Microbiota Transplantation Induces Remission in Patients With Active Ulcerative Colitis in a Randomized Controlled Trial. Gastroenterology 149, 102–109 e106, doi: 10.1053/j.gastro.2015.04.001 (2015).
Khoruts, A., Dicksved, J., Jansson, J. K. & Sadowsky, M. J. Changes in the composition of the human fecal microbiome after bacteriotherapy for recurrent Clostridium difficile-associated diarrhea. Journal of clinical gastroenterology 44, 354–360, doi: 10.1097/MCG.0b013e3181c87e02 (2010).
Colman, R. J. & Rubin, D. T. Fecal microbiota transplantation as therapy for inflammatory bowel disease: a systematic review and meta-analysis. Journal of Crohn’s & colitis 8, 1569–1581, doi: 10.1016/j.crohns.2014.08.006 (2014).
Acknowledgements
Thanks to the Danish 3R center for funding this project. This study was conducted with financial support from the Danish 3R center.
Author information
Authors and Affiliations
Contributions
Study conception and design: L.F.Z., A.K.H., C.H.F.H., K.E., D.S.N. Acquisition of data: L.F.Z., K.E., L.K., W.K. Analysis and interpretation of data: L.F.Z., A.K.H., C.H.F.H., D.S.N., L.K., W.K. Drafting of manuscript: L.F.Z., A.K.H., C.H.F.H., L.K. Critical revision: L.F.Z., A.K.H., C.H.F.H., K.E., D.S.N., L.K., W.K. Final approval: L.F.Z., A.K.H., C.H.F.H., K.E., D.S.N., L.K., W.K.
Corresponding author
Ethics declarations
Competing interests
The authors declare no competing financial interests.
Supplementary information
Rights and permissions
This work is licensed under a Creative Commons Attribution 4.0 International License. The images or other third party material in this article are included in the article’s Creative Commons license, unless indicated otherwise in the credit line; if the material is not included under the Creative Commons license, users will need to obtain permission from the license holder to reproduce the material. To view a copy of this license, visit http://creativecommons.org/licenses/by/4.0/
About this article
Cite this article
Zachariassen, L., Krych, L., Engkilde, K. et al. Sensitivity to oxazolone induced dermatitis is transferable with gut microbiota in mice. Sci Rep 7, 44385 (2017). https://doi.org/10.1038/srep44385
Received:
Accepted:
Published:
DOI: https://doi.org/10.1038/srep44385
This article is cited by
-
Controlling the uncontrolled variation in the diet induced obese mouse by microbiomic characterization
Scientific Reports (2022)
-
The microbiome and rodent models of immune mediated diseases
Mammalian Genome (2021)
-
Dietary prebiotics promote intestinal Prevotella in association with a low-responding phenotype in a murine oxazolone-induced model of atopic dermatitis
Scientific Reports (2020)
-
Bifidobacteria adolescentis regulated immune responses and gut microbial composition to alleviate DNFB-induced atopic dermatitis in mice
European Journal of Nutrition (2020)
-
Severe gut microbiota dysbiosis caused by malnourishment can be partly restored during 3 weeks of refeeding with fortified corn-soy-blend in a piglet model of childhood malnutrition
BMC Microbiology (2019)
Comments
By submitting a comment you agree to abide by our Terms and Community Guidelines. If you find something abusive or that does not comply with our terms or guidelines please flag it as inappropriate.