Abstract
Protection of metal nanoparticles from agglomeration is critical for their functions and applications. The conventional method for enhancing their stability is to cover them with passivation layers to prevent direct contact. However, the presence of a protective shell blocks exposure of the metal species to reactants, thereby significantly impeding the nanoparticles' utility as catalysts. Here, we report that metal nanoparticles can be prepared and used in a surface-exposed state that renders them inherently catalytically active. This strategy is realised by spatial confinement and electronic stabilisation with a dual-module mesoporous and microporous three-dimensional π-network in which surface-exposed nanoparticles are crystallised upon in situ reduction. The uncovered palladium nanoparticles serve as heterogeneous catalysts that are exceptionally active in water, catalyse unreactive aryl chlorides for straightforward carbon–carbon bond formation and are stable for repeated use in various types of cross couplings. Therefore, our results open new perspectives in developing practical heterogeneous catalysts.
Similar content being viewed by others
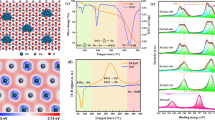
Introduction
Metal nanoparticles (MNPs) are a class of nanomaterials that consist of clusters of reduced metal atoms. They play a pivotal role in areas such as optoelectronics1,2, energy storage and conversion3, catalysts for the production of fuels and chemicals4,5,6,7 and biomedical applications8,9. MNPs tend to agglomerate to form large particles, a phenomenon known as Ostwald ripening, which results in a reduction of their surface area and a loss of their specific functions. A general strategy for preventing agglomeration is to cover the surface with passivation layers that block the direct contact of particles. Various surface-modification methods have been demonstrated to be effective in enhancing the stability of MNPs10,11,12,13,14. However, these methods are not free from problems. The presence of passivation shell unavoidably impedes the energy, electron and magnetic/spin exchanges and interactions with other molecules and thus stifles their inherent functions. A particular concern pertaining to the development of MNP-based catalysts is that the stability of MNPs is enhanced at the expense of catalytic performance because the presence of protective layers renders the metal species under the surface shells inaccessible to reactants. Surface exposure and particle stability are two incompatible aspects; their combination is highly desirable for disclosing their inherent functions and applications but remains a substantial challenge for MNPs15,16,17,18,19.
In this study, we develop a set of techniques concerning the surface-exposed yet stable MNPs, including a general technique for producing MNPs, a facile method to construct dual-module porous π-network as MNP carriers and a practical application of these surface-exposed MNPs as exceptional active heterogeneous catalysts. We explore the surface-exposed MNPs, with preservation of their stability, using conjugated microporous/mesoporous polymers (CMPs) as matrices through the in situ reductive crystallisation of nanoparticles. CMPs are a class of organic porous polymers that combine the properties of π-conjugated three-dimensional networks and permanent nanopores20,21,22,23,24,25,26,27,28,29,30,31,32,33,34, in contrast to other porous materials that are not π-conjugated and to conventional conjugated polymers that are nonporous. We design the CMPs with an electron-rich three-dimensional network capable of π-cloud delocalisation for the electronic stabilisation of uncovered MNPs and with a dual-module nanoporous hierarchy in which mesopores spatially confine palladium nanoparticles (PdNPs) and micropores help to block their association. This uncovered configuration exposes the inherent catalytic functions of MNPs. Surface-exposed PdNPs in H2P-CMP are highly active heterogeneous catalysts (PdNPs⊃H2P-CMP) that function stably in neat water, are robust for repeated use in various cross-coupling reactions and efficiently catalyse even unreactive aryl chlorides for carbon–carbon bond formation. We demonstrate the generality and diverse functions of PdNPs⊃H2P-CMP by using three different types of carbon–carbon bond formations, including Suzuki, Sonogashira and Stille reactions with a wide range of various different reactants. PdNPs⊃H2P-CMP combines activity, stability, reusability, versatility and environmental benignity that meet the requirements of catalysts for practical application. These results unambiguously reveal the significance of exposed surface in disclosing inherent functions and applications of nanoparticles. The present surface-exposure technique is general and widely applicable to other nanoparticles.
The field of homogeneous palladium catalysis for carbon-carbon bond formation remains problematic with respect to the practical application owing to the difficulty in the separation of expensive catalysts for repeated use and the contamination of products by metal species35,36,37,38. The development of heterogeneous catalysts has proved to be increasingly important as an attractive solution to these problems. Using aryl chloride substrates is critical for applications, as they are readily available and inexpensive39,40. However, aryl chlorides are much more difficult to activate than the widely employed aryl bromides and iodides. Although heterogeneous palladium catalysts have been investigated for the carbon–carbon bond formation reactions by loading Pd NPs in various porous supporters such as metal-organic frameworks, covalent organic frameworks, CMPs, porous organic polymers, porous carbon and porous silica and other carriers such as dendrimers, graphenes, nanotubes, linear polymers and oligomers (Table S1), the successful examples that can use aryl chlorides have been very limited; the activation of aryl chlorides remains particularly challenging41,42,43. The majority of heterogeneous systems are working in organic solvents or organic-aqueous mixtures (Table S1)44; the exploration of neat water as solvent is of particular environmental and economic concerns. Cycle performance is crucial for applications, however, satisfying results are rarely achieved for heterogeneous PdNP catalysts thus far reported; the reusability issue remains to be well explored (Table S1).
Results
Synthesis and characterisation
The key to PdNPs⊃H2P-CMP is to combine extended π-cloud delocalisation with dual-module mesopores and micropores in a three-dimensional network (Figs. 1a, b). CMPs are usually obtained as microporous materials; dual-module microporous and mesoporous CMPs are rare21,30,34. We observed that the porosity of CMPs is largely dependent on the reaction time. For example, the Yamamoto coupling reaction of 5, 10, 15, 20-tetrakis(4′-bromophenyl)porphyrin for 24 h yielded H2P-CMP samples with dual-module mesopores and micropores (Fig. S1a, b). Further extension of the reaction time caused the mesopores to disappear and eventually resulted in only microporous networks (Fig. S1c, d). This method is general and applicable to other CMPs21,30,34. PdNPs⊃H2P-CMP was prepared via impregnation of Pd(NO3)2 in H2P-CMP dispersed in THF to generate Pd(II)-impregnated H2P-CMP, followed by reduction under H2 flow at 200°C (Supplementary Information, SI). The PdNPs⊃H2P-CMP assume a spherical shape with a size of 200–300 nm, as revealed by field-emission scanning electron microscopy (FE-SEM, Fig. 1a). The average size of the PdNPs is 2.4 nm (Fig. 1b, c) and the content of PdNPs in PdNPs⊃H2P-CMP is 4.98 wt% as determined by using inductively coupled plasma atomic emission spectroscopy (ICP-AES, SI). PdNPs⊃H2P-CMP is a highly porous dual-module material with a Brunauer–Emmett–Teller surface area of 1031 m2 g−1 and pore volume of 1.03 cm3 g−1 (Figs. 1d, e); it contains both mesopores of 3.5 nm and micropores of 1.5 nm (Fig. 1d). The contributions of mesopores and micropores to the total pore volume are 21% and 79%, respectively. Usually, porous materials upon loading of PdNPs leave low BET surface area and small pore volume in the resulted products (Table S1). Notably, the PdNPs⊃H2P-CMP preserves a high BET surface area and large pore volume, as a result of highly porous and dual-module structure of H2P-CMP. Such a porous structure benefits the transport of reactants into and products out of the heterogeneous catalysts. High-resolution transmission electron microscopy (HR-TEM) revealed that PdNPs in PdNPs⊃H2P-CMP are crystals with clear (111) facets that have a d-spacing of 2.24 Å (Fig. 1d). X-ray diffraction (XRD) profiles of PdNPs⊃H2P-CMP revealed a series of peaks at 40.7°, 46.7°, 68.2° and 82.2°, which are assigned to the (111), (200), (220) and (311) facets, respectively (Fig. 1e). X-ray photoelectron spectroscopy (XPS) measurements indicate a reduced Pd(0) state on the basis of the characteristic peaks at 334.7 and 340.3 eV, which are assigned to Pd 3d5/2 and Pd 3d3/2 electrons, respectively (Fig. 1g). The small peaks at 336.1 and 341.2 eV are assignable to Pd(II) 3d5/2 and Pd(II) 3d3/2 electrons, respectively. The molar ratio of Pd(0) to Pd(II) is estimated to be 97:3 in the CMPs as evaluated from the XPS curves.
Synthesis and characterization.
(a), Nitrogen sorption isotherms of PdNPs⊃H2P-CMP measured at 77 K. (b), Pore size distribution and pore volume profiles of PdNPs⊃H2P-CMP. (c), FE-SEM image of PdNPs⊃H2P-CMP. (d), HR-TEM image of PdNPs⊃H2P-CMP. (e), XRD patterns of H2P-CMP (black; amorphous halo peak at 18°) and PdNPs⊃H2P-CMP. (f), Size distribution profile of PdNPs. (g), XPS profile of PdNPs⊃H2P-CMP.
H2P-CMP with an extended π-conjugation network, as indicated by its red-shifted Soret absorption band (Fig. S2), constitutes an electron-rich environment that electronically stabilises the uncovered PdNPs45,46,47. In the PdNPs⊃H2P-CMP, the mesopores (3.5 nm) are much larger than the average size (2.4 nm) of PdNPs, in addition to the presence of micropores, thus leaving sufficient space for mass transfer to PdNPs. The dual-module porous structure helps lock PdNPs within the mesopores because PdNPs are too large to pass through the micropores. PdNPs⊃H2P-CMP is stably dispersible in neat water (Fig. S3) and is thermally stable up to 500°C (Fig. S4).
Heterogeneous catalysis of Suzuki reaction
The Suzuki cross-coupling reactions of various aryl chlorides with arylboronic acids were investigated in the presence of the PdNPs⊃H2P-CMP catalyst (Table 1). Suzuki coupling reactions are typically conducted in organic solvents or in aqueous-organic mixed solvents (Table S1). The use of neat water as an environmentally benign solvent has received considerable attention with respect to green chemistry.
The heterogeneous catalytic reaction was carried out in neat water at 80°C (Table 1). A phase-transfer reagent, tetrabutylammonium bromide (TBAB), was added to enhance the reactivity in water (for optimized condition see Table S2). As a result, we were able to lower the catalyst loading to 0.5 mol% (Table S3). Outstanding catalytic activity was observed for the coupling reaction of chlorobenzene (entry 1) and activated aryl chlorides, such as 4-chlorobenzonitrile (entries 2 and 3), 4-acetyl-1-chlorobenzene (entry 4), 1-chloro-4-formyl-benzene (entry 5), 1-chloro-4-fluoro-benzene (entry 6) and 1-chloro-4-nitrobenzene (entry 7), with phenylboronic acid.
High activities were achieved using the PdNPs⊃H2P-CMP catalyst for deactivated aryl chlorides, such as 1-chloro-4-methyl-benzene (entry 8), 1-chloro-4-methoxy-benzene (entry 9) and 1-chloro-3-methyl-benzene (entry 10). PdNPs⊃H2P-CMP catalysed efficiently non-robust hetero cross-coupling reactions in which steric hindrance at ortho-positions relative to the chloride group are unreactive and are typically difficult to accomplish48,49. Notably, the PdNPs⊃H2P-CMP catalyst successfully facilitated the coupling reactions of the severely deactivated aryl chlorides, such as 2-chlorotoluene (entry 11), 2-chlorobenzonitrile (entry 12) and 1-chloro-2, 5-dimethyl-benzene (entry 13), with phenylboronic acid. These coupling reactions were achieved in excellent yields. Phenylboronic acids with different substituents, such as 4-cyano-phenylboronic acid (entry 2), 4-methylphenylboronic acid (entries 3, 8 and 12) and 4-methoxyphenylboronic acid (entry 7), are favourable for catalysis by PdNPs⊃H2P-CMP. All of the coupling reactions, irrespective of the types and positions of the substituent groups, proceeded cleanly and highly efficiently in water.
The Suzuki coupling reaction catalysed by PdNPs⊃H2P-CMP was promoted using microwave irradiation at 100°C with the same catalyst loading of 0.5 mol% (Table 1). The reaction time was shortened from 12 h to only 25 min while preserving the high yields (Table 1). Remarkably, the coupling of phenylboronic acid with unreactive aryl chlorides such as 2-chlorotoluene (entry 11), 2-chlorobenzonitrile (entry 12) and 1-chloro-2,5-dimethyl-benzene (entry 13), proceeded smoothly and achieved high yields upon 25-min microwave irradiation.
The coupling reaction of chlorobenzene and phenylboronic acid was further performed in the presence of commercially available Pd catalysts, including heterogeneous catalysts Pd/C and Pd/Al2O3 and the authentic homogeneous catalyst Pd(OAc)2 and gave rise to rather low yields (entries 14–16). To the best of our knowledge, PdNPs⊃H2P-CMP exhibits the highest catalytic activity reported to date for the heterogeneous Suzuki coupling reactions of aryl chlorides (Table S1).
Heterogeneous catalysis of Sonogashira reaction
Encouraged by the notable catalytic performance of PdNPs⊃H2P-CMP in the Suzuki coupling reaction, we performed a Sonogashira coupling reaction of aryl chlorides with alkynes in the presence of the PdNPs⊃H2P-CMP catalyst. Typically, the use of aryl chlorides requires a high catalyst loading, elevated reaction temperature, prolonged reaction time and the use of either CuI as co-catalyst or large amounts of ligands such as phosphines36. Remarkably, we were able to conduct efficient ligand and copper-free Sonogashira coupling reactions of various aryl chlorides at 80°C in the presence of PdNPs⊃H2P-CMP in neat water without enhanced catalyst loading (Table 2). High activity was achieved for the reactions of phenylacetylene with chlorobenzene (entry 1) and activated aryl chlorides, such as 1-chloro-4-nitrobenzene (entries 2 and 3), 1-chloro-4-acetylbenzene (entry 4) and 1-chloro-4-trifluoromethylbenzene (entry 5). The coupling reactions of these aryl chlorides are achieved in 94-96% yields. Deactivated aryl chlorides such as 4-chloro-toluene (entries 6 and 7), 1-chloro-4-methoxybenzene (entry 8) and 1-chloro-4-ethylbenzene (entry 9) were efficiently activated by PdNPs⊃H2P-CMP to afford good yields. In addition to aryl chlorides, a heteroaryl chloride, 2-chloropyridine (entry 10), which is particularly detrimental to the catalytic activity of palladium49, was readily activated by PdNPs⊃H2P-CMP to give high yield. Notably, severely deactivated aryl chlorides such as 2-chlorobenzonitrile (entry 11) and 1-chloro-2, 5-dimethyl-benzene (entry 12) reacted with phenylacetylene effectively and achieved satisfactory yields of 84% and 90%, respectively, without requiring a higher reaction temperature or an enhanced loading of the catalyst. The PdNPs⊃H2P-CMP exhibited high activity, regardless of the presence of activated and deactivated substituents, toward phenylacetylene (entries 3, 5, 7, 9 and 10). Notably, this is the first successful example of a heterogeneous PdNP catalyst for the Sonogashira reaction of aryl chlorides in neat water (Table S1).
Microwave irradiation also promoted the Sonogashira couplings catalysed by PdNPs⊃H2P-CMP. As shown in Table 2, the reaction time was significantly shortened, to 20 min, whereas the yields were improved to 90–96%, irrespective of substituents on both reactants.
Heterogeneous catalysis of Stille reaction
On the basis of these results, we conducted the Stille coupling reaction of aryl chlorides with organostannanes in the presence of PdNPs⊃H2P-CMP in neat water at 80°C (Table 3). To our best knowledge, heterogeneous catalysts that activate aryl chlorides for the Stille reaction in water have been very limited (Table S1)23. The reaction of chlorobenzene (entry 1), activated aryl chlorides, such as 4-acetyl-1-chlorobenzene (entry 2), 1-chloro-4-formylbenzene (entry 3), 1-chloro-4-fluorobenzene (entry 4) and 1-chloro-3-nitrobenzene (entry 5) with trimethyl(phenyl)stannane proceeded smoothly in 5 h, with complete conversion and high yields of 93–96%. Satisfyingly, deactivated 1-chloro-4-methoxybenzene was efficiently converted in 5 h, with an impressive yield of 94% (entry 6). Furthermore, for severely deactivated 2-chlorotoluene (entry 7), 2-chlorobenzonitrile (entry 8) and 1-chloro-2, 5-dimethyl-benzene (entry 9), the Stille reaction catalysed by PdNPs⊃H2P-CMP afforded noteworthy yields of 94%, 86% and 88%, respectively; no prolonged reaction times or elevated reaction temperatures are required. To our knowledge, this result also represents the first example of a MNP-catalysed heterogeneous Stille coupling reaction of aryl chlorides (Table S1). Under microwave irradiation at 100°C, the Stille coupling of aryl chlorides with organostannne was further promoted; PdNPs⊃H2P-CMP completely converts all of the aryl chlorides, reaching high yields in only 15 min, irrespective of their substituents (Table 3).
PdNPs⊃H2P-CMP efficiently catalyses aryl chlorides in Suzuki, Sonogashira and Stille coupling reactions. One of the structural features of PdNPs⊃H2P-CMP is the uncovered PdNPs with a catalytically active Pd(0) surface that is readily accessible to aryl chlorides. Mechanistically, oxidative addition of aryl chlorides to Pd(0) is the key step for the coupling reaction. The superb catalytic activity observed for PdNPs⊃H2P-CMP indicates that PdNPs⊃H2P-CMP facilitates the oxidative addition step as well as the reductive elimination.
Discussions
Turnover number and frequency
We evaluated the turnover number (TON) and turnover frequency (TOF) with 0.02 mol% Pd for the aforementioned three types of C–C bond formation reactions using 50 mmol of chlorobenzene under microwave irradiation conditions. Remarkably, for the Suzuki coupling of chlorobenzene and phenylboronic acid, the yield was 96% after 45 min; thus, the TON and TOF were 4800 and 6400 h−1, respectively. The Sonogashira reaction of chlorobenzene and phenylacetylene reached 98% yield in 30 min; the TON and TOF were evaluated to be 4900 and 9800 h−1, respectively. In the Stille reaction of chlorobenzene and trimethyl(phenyl)stannane, 96% yield was achieved in 20 min; the TON and TOF were 4800 and 14400 h−1, respectively. Most of the heterogeneous PdNP catalytic systems have not been evaluated their TON and TOF values (Table S1). In Table S1, the reaction yields are given in percentage when the Pd catalysts utilized the aryl chlorides as substrates. While the Pd catalysts are inactive for the aryl chlorides, the symbol “−” was used for the yield. As summarized in Table S1, PdNP⊃H2P-CMP affords the highest yields among the catalysts.
Kinetic aspects
Kinetic studies were conducted through the entire reaction region between 0% and 100% conversion, with each experiment performed at least in duplicate, whereas the average conversions were used for kinetics evaluations. The decease of chlorobenzene (open circles) and increase of the product (filled circles) in the coupling reactions are straightforward and the catalytic reactions proceed smoothly (Figure 2a–c). It is noteworthy that no induction periods are observable for these surface-exposed metal nanoparticle heterogeneous catalytic systems.
Reaction kinetics and cycle performance.
(a–c), Kinetics of (a) Suzuki, (b) Sonogashira and (c) Stille coupling reactions between chlorobenzene (CB) and phenylboronic acid phenylacetylene or trimethyl(phenyl)stannane (open circle: the remained CB concentration relative to loading concentration; filled circle: the coupling product concentration). (d–f), Plot of natural logarithm of the remained concentration of CB during reaction vs. time in (d) Suzuki, (e) Sonogashira and (f) Stille coupling reactions. (g–i), Recycling of PdNPs⊃H2P-CMP (0.5 mol% Pd) for (g) Suzuki, (h) Sonogashira and (i) Stille coupling reactions under thermal (red bar) and microwave (black bar) conditions.
Under the reported conditions, all of the model reactions showed apparent first-order behavior (Figure 2d–f), consistent with a zero-order dependence on phenylboronic acid, phenylacetylene and trimethyl(phenyl)stannane. The rate constant (kobs) and lifetime (t1/2) were calculated to be kobs = 0.222 ± 0.006 h−1 and t1/2 = 4.96 h for Suzuki reaction, kobs = 0.704 ± 0.014 h−1 and t1/2 = 1.96 h for Sonogashira reaction and kobs = 0.920 ± 0.027 h−1 and t1/2 = 1.64 h for Stille reaction. The average catalytic productivity and activity of PdNPs⊃H2P-CMP are increased in the order of Suzuki, Sonagashira and Stille reactions. A plausible explanation for the observed first-order rate may involve the oxidative addition of aryl chlorides to the surface-exposed nanoparticle catalyst as the rate-determining step, while the capture of corresponding Pd-intermediates by phenylboronic acid, phenylacetylene or trimethyl(phenyl)stannane is immediate with a consequent rapid reductive elimination to yield the corresponding cross-coupling products.
Catalyst longevity
A long catalyst lifetime and the capability of repeated use are highly desired for industrial applications. The PdNPs⊃H2P-CMP catalyst is easily separated from the reaction mixture and recovered; filtration and subsequent rinsing with solvents and water refreshed the catalyst for the next reaction round. As for the heterogeneous PdNP catalysts thus far reported, their ability of cycle use for the activation of aryl chlorides remains unclear (Table S1). In general, MNP catalysts undergo considerable agglomeration during reactions and finally lose activity. PdNPs⊃H2P-CMP was subjected to repeated use for the Suzuki, Sonagashira and Stille coupling reactions under both thermal and microwave conditions. Notably, the PdNPs⊃H2P-CMP catalyst retained its high activities and achieved high yields even after 10 cycles (Figure 2g–i), without the use of a prolonged reaction time or elevated reaction temperature. The PdNPs⊃H2P-CMP catalyst exhibits the longest catalyst lifetime reported to date for heterogeneous carbon-carbon bond formation reactions. Together with the high activity and broad reactant scope, the excellent extended performance renders PdNPs⊃H2P-CMP a more economic and environmentally benign process.
To gain structural insights into the extended performance of PdNPs⊃H2P-CMP, we characterised the catalyst after 10 cycles using various analytical methods. Firstly, the size distribution of PdNPs was almost unchanged after ten cycles (Fig. S5a–c), which suggests that the agglomeration of PdNPs was negligible (Fig. S5d–f). Secondly, XRD measurements revealed that the crystal structure of PdNPs in PdNPs⊃H2P-CMP was retained after 10 cycles (Fig. S6). The XPS profile suggests that PdNPs are retained in the Pd(0) state (Fig. S7). Thirdly, ICP-AES monitoring of the filtrates of the reaction mixtures revealed that no Pd was leached during 8 cycles, while was less than 0.01 mol% of the starting Pd catalyst in the filtrates of the reaction mixtures was detected after nine cycles. The Pd content in PdNPs⊃H2P-CM after 10 cycles was 4.96 wt%, which is very close to that (4.98 wt%) of the pristine catalyst. These results indicate that PdNPs⊃H2P-CMP is maintained within the pores of three-dimensional π-network, without losing its crystallinity and catalytic activity.
In summary, we have developed the techniques for producing surface-exposed yet stable metal nanoparticles by locking them within a dual-module mesoporous and microporous three-dimensional π-network. The palladium nanoparticles exhibit inherently superior activity in the heterogeneous catalysis of different types of carbon–carbon bond formation reactions. Unreactive aryl chlorides are efficiently catalysed in Suzuki, Sonogashira and Stille coupling reactions in neat water under mild conditions. This novel class of heterogeneous catalysts, unlike previous examples thus far reported, combines activity, stability, reusability, versatility and environmental compatibility; these advantages offer a plausible solution to long-standing challenges for real applications in the field of heterogeneous catalyst. Therefore, these advancements open new perspectives in the design of heterogeneous catalysts for the sustainable production of fuels and chemicals. The present technique is applicable to producing various surface-exposed metal nanoparticles; utilization of this technique may disclose inherent functions and applications of other nanoparticles.
Methods
H2P-CMP
1,5-Cyclooctadiene (65 mg, 0.802 mmol) was added to a solution of bis(1,5-cyclooctadiene)nickel(0) (170 mg, 0.62 mmol) and 2, 2′-bipyridyl (96 mg, 0.62 mmol) in freshly distilled dehydrated dioxane (10 mL) and the mixture was heated at 90°C for 1 h. To the purple mixture solution was added 5, 10, 15, 20-tetrakis(4'-bromophenyl)porphyrin (121 mg, 0.13 mmol) and the mixture was stirred at 100°C for 24 h. After cooling, concentrated HCl (4 mL) was added to the mixture. The precipitate was collected by filtration, washed with saturated aqueous ethylenediaminetetraacetic acid solution (50 mL), H2O (30 mL × 5), CHCl3 (30 mL × 5) and THF (30 mL × 5), extracted by using Soxhlet with methanol, acetone and THF for 2 days, respectively and dried at 150°C under vacuum overnight, to afford H2P-CMP as red purple powders in 91% isolation yield.
PdNPs⊃H2P-CMP
An H2P-CMP sample (500 mg) was dispersed in THF (10 mL) and was stirred for 2 h at room temperature. A THF solution (1 mL) of 150 mg Pd(NO3)2·2H2O was added dropwise to the H2P-CMP dispersion under vigorous stirring. The 20 mL vial containing the slurry was subjected to ultrasounication for 30 min and was then magnetically stirred at room temperature for 48 h. The Pd(II)-impregnated H2P-CMP sample was collected by filtration, washed with THF till the filtrate became colorless and was slowly dried in air at room temperature for 24 h to yield Pd(II)⊃H2P-CMP. The Pd(II)⊃H2P-CMP sample was further dried at 150°C under vacuum overnight and heated in a stream of H2 at 200°C for 6 h to yield PdNPs⊃H2P-CMP.
General procedure for Suzuki coupling reactions
Aryl chlorides (0.50 mmol), arylboronic acids (0.75 mmol), PdNPs⊃H2P-CMP (0.5 mol%), TBAB (0.5 mmol) and 1.5 M KOH aqueous solution (1 M) were added to a 5 mL dry Schlenk tube with a stirring bar. Argon was bubbled through the solution for 10 min. The reaction mixture was stirred at 80°C for 12 h and then cooled to room temperature. After separating the catalyst by filtration, the aqueous phase was extracted with EtOAc (3 mL × 5) and the collected organic phase was dried over anhydrous magnesium sulfate, evaporated to dryness and further purified by silica gel column chromatography.
General procedure for Sonogashira coupling reactions
Aryl chlorides (0.5 mmol), alkynes (0.6 mmol), PdNPs⊃H2P-CMP (0.5 mol%), TBAB (0.5 mmol) and 1.5 M Cs2CO3 aqueous solution (1 mL) were added to a 5 mL dry Schlenk tube with a stirring bar. Argon was bubbled through the solution for 10 min. The reaction mixture was stirred at 80°C for 8 h and then cooled to room temperature. After separating the catalyst by filtration, the aqueous phase was extracted with EtOAc (3 mL × 5) and the collected organic phase was dried over anhydrous magnesium sulfate, evaporated to dryness and further purified by silica gel column chromatography.
General procedure for Stille coupling reactions
Aryl chlorides (0.5 mmol), trimethyl(phenyl)stannane (0.6 mmol), PdNPs⊃H2P-CMP (0.5 mol%), TBAF (1.5 mmol) and water (1 mL) were added to a 5 mL dry Schlenk tube with a stirring bar. Argon was bubbled through the solution for 10 min. The reaction mixture was stirred at 80°C for 5 h and then cooled to room temperature. After separating the catalyst by filtration, the aqueous phase was extracted with EtOAc (3 mL × 5) and the collected organic phase was dried over anhydrous magnesium sulfate, evaporated to dryness and further purified by silica gel column chromatography.
General procedure for cycle use
For testing cycle performance of PdNPs⊃H2P-CMP, coupling reactions were conducted by maintaining the same reaction conditions as described above, except the catalyst. The catalyst was isolated by filtration of the reaction mixture at the end of each cycle, washed with toluene, THF and water and then dried at 120°C under vacuum overnight. The catalyst was used in the next-round reaction.
References
Maier, S. A. et al. Local detection of electromagnetic energy transport below the diffraction limit in metal nanoparticle plasmon waveguides. Nature Mater. 2, 229–232 (2003).
Cho, K.-S. et al. High-performance crosslinked colloidal quantum-dot light-emitting diodes. Nature Photonics 3, 341–345 (2009).
Aricò, A. S., Bruce, P., Scrosati, B., Tarascon, J. M. & van Schalkwijk, W. Nanostructured materials for advanced energy conversion and storage devices. Nature Mater. 4, 366–377 (2005).
Tao, F. Metal nanoparticles for catalysis: advances and applications. RSC Publishing, Cambridge, 2014.
Román-Leshkov, Y., Barret, C. J., Liu, Z. Y. & Dumesic, J. A. Production of dimethylfuran for liquid fuels from biomass-derived carbohydrates. Nature 447, 982–985 (2007).
Kesavan, L. et al. Solvent-free oxidation of primary carbon-hydrogen bonds in toluene using Au–Pd alloy nanoparticles. Science 14, 195–199 (2011).
Torres, G. et al. Supported iron nanoparticles as catalysts for sustainable production of lower olefins. Science 17, 835–838 (2012).
Thanha, N. T. K. & Green, L. A. W. Functionalisation of nanoparticles for biomedical applications. Nano Today 5, 213–230 (2010).
Rosi, N. L. & Mirkin, C. A. Nanostructures in biodiagnostics. Chem. Rev. 105, 1547–1562 (2005).
McCaffrey, R. et al. Template synthesis of gold nanoparticles with an organic molecular cage. J. Am. Chem, Soc. 136, 1782–1785 (2014).
Wang, Y., Zhang, J., Zhang, W. & Zhang, M. Pd-catalysed C−C cross-coupling reactions within a thermoresponsive and pH-responsive and chelating polymeric hydrogel. J. Org. Chem. 74, 1923–1931 (2009).
Wen, F. et al. Synthesis of noble metal nanoparticles embedded in the shell layer of core-shell poly(styrene-co-4-vinylpyridine) micospheres and their application in catalysis. Chem. Mater. 20, 2144–2150 (2008).
Gallon, B. J., Kojima, R. W., Kaner, R. B. & Diaconescu, P. L. Palladium nanoparticles supported on polyaniline nanofibers as a semi-heterogeneous catalyst in water. Angew. Chem., Int. Ed., 46, 7251–7254 (2007).
Ogasawara, S. & Kato, S. Palladium nanoparticles captured in microporous polymers: a tailor-made catalyst for heterogeneous carbon cross-coupling reactions. J. Am. Chem. Soc. 132, 4608–4613 (2010).
Zhang, P., Weng, Z., Guo, J. & Wang, C. Solution-dispersible, colloidal, conjugated porous polymer networks with entrapped palladium nanocrystals for heterogeneous catalysis of the Suzuki–Miyaura coupling reaction. Chem. Mater. 23, 5243–5249 (2011).
Johnston, E. V. et al. Highly dispersed palladium nanoparticles on mesocellular foam: an efficient and recyclable heterogeneous catalyst for alcohol oxidation. Chem. Eur. J. 18, 12202–12206 (2012).
Siamaki, A. R., Lin, Y., Woodberry, K., Connell, J. W. & Gupton, B. F. Palladium nanoparticles supported on carbon nanotubes from solventless preparations: versatile catalysts for ligand-free Suzuki cross coupling reactions. J. Mater. Chem. A 1, 12909–12918 (2013).
Chan-Thaw, C. E. et al. Covalent triazine framework as catalytic support for liquid phase reaction. Nano Lett. 10, 537–541 (2010).
Yin, L. & Liebscher, J. Carbon-carbon coupling reactions catalysed by heterogeneous palladium catalysts. Chem. Rev. 107, 133–173 (2007).
Jiang, J. X. et al. Metal–organic conjugated microporous polymers. Angew. Chem., Int. Ed. 50, 1072–1075 (2011).
Chen, L., Yang, Y. & Jiang, D. CMPs as scaffolds for constructing porous catalytic frameworks: a built-in heterogeneous catalyst with high activity and selectivity based on nanoporous metalloporphyrin polymers. J. Am. Chem. Soc. 132, 9138–9143 (2010).
Wang, C. A. et al. “Bottom-up” embedding of the Jørgensen–Hayashi catalyst into a chiral porous polymer for highly efficient heterogeneous asymmetric organocatalysis. Chem. Eur. J. 18, 6718–6723 (2012).
Xie, Y., Wang, T., Liu, X., Zou, K. & Deng, W. Capture and conversion of CO2 at ambient conditions by a conjugated microporous polymer. Nature Commun. 4, 10.1038/ncomms2960 (2013).
Xu, Y., Jin, S., Xu, H. & Jiang, D. Conjugated microporous polymers: design, synthesis and application. Chem. Soc. Rev. 42, 8012–8031 (2013).
Jiang, J. X. et al. Conjugated microporous poly(aryleneethynylene) networks. Angew. Chem., Int. Ed. 46, 8574–8578 (2007).
Kou, Y., Xu, Y., Guo, Z. & Jiang, D. Supercapacitive energy storage and electric power supply using an aza-fused π-conjugated microporous framework. Angew. Chem., Int. Ed. 50, 8753–8757 (2011).
Chen, L., Yang, Y., Guo, Z. & Jiang D. Highly efficient activation of molecular oxygen with nanoporous metalloporphyrin frameworks in heterogeneous systems. Adv. Mater. 23, 3149–3154 (2011).
Liu, X., Xu, Y. & Jiang, D. Conjugated microporous polymers as molecular sensing devices: microporous architecture enables rapid response and enhances sensitivity in fluorescence-on and fluorescence-off sensing. J. Am. Chem. Soc. 134, 8738–8741 (2012).
Xu, Y., Chen, L., Guo, Z., Nagai, A. & Jiang, D. Light-emitting conjugated polymers with microporous network architecture: interweaving scaffold promotes electronic conjugation, facilitates exciton migration and improves luminescence. J. Am. Chem. Soc. 133, 17622–17625 (2011).
Wu, Z. et al. High-performance electrocatalysts for oxygen reduction derived from cobalt porphyrin-based conjugated mesoporous polymers. Adv. Mater. 26, 1450–1455 (2013).
Gu, C. et al. Controlled synthesis of conjugated microporous polymer films: versatile platforms for highly sensitive and label-free chemo- and biosensing. Angew. Chem., Int. Ed. 53, 4850–4855 (2014).
Zhang, K., Kopetzki, D., Seeberger, P. H., Antonietti, M. & Vilela, F. Surface area control and photocatalytic activity of conjugated microporous poly(benzothiadiazole) networks. Angew. Chem., Int. Ed. 52, 1432–1436 (2013).
Xu, F. et al. Redox-active conjugated microporous polymers: a new organic platform for highly efficient energy storage. Chem. Commun. 50, 4788–4790 (2014).
Dawson, R. et al. Mesoporous poly(phenylenevinylene) networks. Macromolecules 41, 1591–1593 (2008).
Jana, R., Pathak, T. P. & Sigman, M. S. Advances in transition metal (Pd,Ni,Fe)-catalysed cross-coupling reactions using alkyl-organometallics as reaction partners. Chem. Rev. 111, 1417–1492 (2011).
Cahiez, G. & Moyeux, A. Cobalt-catalysed cross-coupling reactions. Chem. Rev. 110, 1435–1462 (2010).
Miyaura, N. & Suzuki, A. Palladium-catalysed cross-coupling reactions of organoboron compounds. Chem. Rev. 95, 2457–2483 (1995).
Chinchilla, R. & Nájera, C. The Sonogashira reaction: a booming methodology in synthetic organic chemistry. Chem. Rev. 107, 874–922 (2007).
Martin, R. & Buchwald, S. L. Palladium-catalysed Suzuki–Miyaura cross-coupling reactions employing dialkylbiaryl phosphine ligands. Acc. Chem. Res. 41, 1461–1473 (2008).
Littke, A. F. & Fu, G. C. Palladium-catalysed coupling reactions of aryl chlorides. Angew. Chem., Int. Ed. 41, 4176–4211 (2002).
Yuan, B., Pan, Y., Li, Y., Yin, B. & Jiang, H. A highly active heterogeneous palladium catalyst for the Suzuki–Miyaura and Ullmann coupling reactions of aryl chlorides in aqueous media. Angew. Chem., Int. Ed. 49, 4054–4058 (2010).
Jin, M.-J. & Lee, D.-H. A practical heterogeneous catalyst for the Suzuki, Sonogashira and Stille Coupling reactions of unreactive aryl chlorides. Angew. Chem., Int. Ed. 49, 1119–1122 (2010).
Li, B. et al. Highly dispersed Pd catalyst locked in knitting aryl network polymers for Suzuki–Miyaura coupling reactions of aryl chlorides in aqueous media. Adv. Mater. 24, 3390–3395 (2012).
Butler, R. N. & Coyne, A. G. Water: nature's reaction enforcer-comparative effects for organic synthesis “in-water” and “on-water”. Chem. Rev. 110, 6302–6337 (2010).
Scheuermann, G. M., Rumi, L., Steurer, P., Bannwarth, W. & Mulhaupt, R. Palladium nanoparticles on graphite oxide and its functionalized graphene derivatives as highly active catalysts for the Suzuki-Miyaura coupling reaction. J. Am. Chem. Soc. 131, 8262–8270 (2009).
Zhang, P., Gong, Y., Li, H., Chen, Z. & Wang, Y. Solvent-free aerobic oxidation of hydrocarbons and alcohols with Pd@N-doped carbon from glucose. Nature Commun. 4., 10.1038/ncomms2586 (2013).
Okamoto, K., Akiyama, R., Yoshida, H., Yoshida, T. & Kobayashi, S. Formation of nanoarchitectures including subnanometer palladium clusters and their use as highly active catalysts. J. Am. Chem. Soc. 127, 2125–2135 (2005).
Kudo, N., Perseghini, N. & Fu, G. C. A versatile method for Suzuki cross-coupling reactions of nitrogen heterocycles. Angew. Chem., Int. Ed. 45, 1282–1284 (2006).
Billingsley, K. L., Anderson, K. W. & Buchwald, S. L. A highly active catalyst for Suzuki–Miyaura cross-coupling reactions of heteroaryl compounds. Angew. Chem., Int. Ed. 45, 3484–3486 (2006).
Acknowledgements
D.J. acknowledges the support of a Grant-in-Aid for Scientific Research (A) (24245030) from the Ministry of Education, Culture, Sports, Science and Technology, Japan (MEXT).
Author information
Authors and Affiliations
Contributions
D.J. conceived the project, designed experiments and provided funding. N.H. and Y.X. performed experiments. N.H. and D.J. wrote the manuscript.
Ethics declarations
Competing interests
The authors declare no competing financial interests.
Electronic supplementary material
Supplementary Information
Supplementary Information
Rights and permissions
This work is licensed under a Creative Commons Attribution-NonCommercial-NoDerivs 4.0 International License. The images or other third party material in this article are included in the article's Creative Commons license, unless indicated otherwise in the credit line; if the material is not included under the Creative Commons license, users will need to obtain permission from the license holder in order to reproduce the material. To view a copy of this license, visit http://creativecommons.org/licenses/by-nc-nd/4.0/
About this article
Cite this article
Huang, N., Xu, Y. & Jiang, D. High-performance heterogeneous catalysis with surface-exposed stable metal nanoparticles. Sci Rep 4, 7228 (2014). https://doi.org/10.1038/srep07228
Received:
Accepted:
Published:
DOI: https://doi.org/10.1038/srep07228
This article is cited by
-
A tri-layer approach to controlling nanopore formation in oxide supports
Nano Research (2019)
-
Micro/mesoporous conjugated fluorinated iron-porphyrin polymer: porosity and heterogeneous catalyst for oxidation
Advanced Composites and Hybrid Materials (2018)
Comments
By submitting a comment you agree to abide by our Terms and Community Guidelines. If you find something abusive or that does not comply with our terms or guidelines please flag it as inappropriate.