Abstract
Reproductive diapause serves as biological mechanism for many insects, including the mosquito Culex pipiens, to overwinter in temperate climates. While Cx. pipiens diapause has been well-studied in the laboratory, the timing and environmental signals that promote diapause under natural conditions are less understood. In this study, we examine laboratory, semi-field, and mosquito surveillance data to define the approximate timeline and seasonal conditions that contribute to Cx. pipiens diapause across the United States. While confirming integral roles of temperature and photoperiod in diapause induction, we also demonstrate the influence of latitude, elevation, and mosquito population genetics in shaping Cx. pipiens diapause incidence across the country. Coinciding with the cessation of WNV activity, these data can have important implications for mosquito control, where targeted efforts prior to diapause induction can decrease mosquito populations and WNV overwintering to reduce mosquito-borne disease incidence the following season.
Similar content being viewed by others
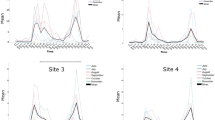
Introduction
Insects are one of the most diverse lifeforms on the planet, relying on a myriad of evolutionary adaptations to survive adverse ecological environments and climate conditions. This includes a state of dormancy known as diapause that is used to facilitate overwintering survival in temperate regions1,2, which depending on species can occur during the egg, larval, or adult stages3.
The northern house mosquito, Culex pipiens, is an important vector of mosquito-borne pathogens such as West Nile virus (WNV) and Saint Louis encephalitis virus (SLEV), and serves as an important model to understand diapause physiology in insects3,4,5. Evidence suggests that the immature life stages (larvae and pupae) respond to photoperiod and temperature cues to promote facultative diapause in adult female mosquitoes following eclosion6,7,8, where the resulting females forego blood-feeding9,10 and remain in an arrested reproductive state characterized by small primary ovarian follicles3,11,12. Additional physiological changes to the cuticle13,14 and reduced diuresis15 protect against desiccation, while alterations to host metabolism increase lipid and glycogen storage16,17,18,19,20 to help sustain overwintering survival.
Photoperiod and temperature are long-established components of mosquito facultative diapause induction, where short-day lengths and cool temperatures promote this physiological state6,8. Previous studies with Cx. pipiens suggest that day lengths under 15 h can elicit the diapause under laboratory conditions using cool temperatures (18–22 °C)6,8. Evidence suggests that diapause induction is stronger with shorter photophases and lower temperatures, where ~100% of a colony can be induced under 12 h of daylight and at 18 °C6. However, higher temperatures can revert diapausing individuals or suppress diapause entry, even at lower photophases5,7,21, demonstrating the combined importance of photoperiod and temperature in defining the diapause state.
Laboratory experiments to induce diapause using a short photoperiod (9:15) and cool temperature (19 °C) have produced significant insights into the hormonal regulation4,18,22,23 and molecular physiology of Cx. pipiens diapause17,24,25,26, yet these experimental conditions in the laboratory do not capture the natural fluctuations in daily temperature that encompass the realistic end-season conditions that promote diapause induction. Limited studies have addressed the diapause induction timeline in natural populations of Cx. pipiens. Semifield experiments in Ontario, Canada, recorded diapause incidence as early as July, with peak rates in mid-August21, while field-collected mosquito samples in Boston, USA revealed low levels of diapause incidence beginning in mid-August, with peak incidence in late September/early October27. In addition, evidence suggests that there is annual variation in diapause induction, with peak periods of induction varying by ~2 weeks between years27. Together, this geographic and yearly variability in diapause incidence highlight our limited understanding of Cx. pipiens diapause under natural conditions.
Herein, we perform laboratory and semifield studies to examine diapause induction in a laboratory population of Cx. pipiens, confirming the requirements of both photoperiod and temperature on diapause induction, as well as provide temporal evidence of the natural seasonal conditions that promote diapause in central Iowa, USA. To place these data in the context of diapause incidence in natural mosquito populations, we leverage gravid Cx. pipiens population data from Iowa and multiple locations across the United States to serve as a proxy for diapause incidence. These data suggest that temperature, latitude, elevation, and Culex population genetics significantly impact natural diapause ecology. Together, our data provide significant new insight into the complexity of Cx. pipiens diapause induction and its influence in end-of-season mosquito population trends. These results have important public health implications for mosquito-borne disease transmission, and increase our understanding of how a globally changing climate may extend mosquito activity and influence mosquito overwintering.
Results
Diapause induction requires both short-day lengths and cool temperatures
With the intention to use a laboratory colony of Culex pipiens originally isolated from Ames, Iowa and maintained for ~16 years in the laboratory (without selection for the diapause state), we first wanted to demonstrate diapause induction in this Cx. pipiens population through laboratory experiments. To approach this question, we reared Cx. pipiens from first-instar larvae to adults under different laboratory conditions (Fig. 1a) to examine the influence of temperature alone (cold; 16:8 L:D, 19 °C), photoperiod alone (dark; 9:15 L:D, 25 °C), and the combined effects of temperature and photoperiod (diapause; 9:15 L:D, 19 °C) to promote adult diapause10,18,28.
Culex pipiens first-instar larvae were either maintained under standard insectary conditions (control) or transferred to rearing conditions to examine the effects of cold temperature (cold), short photoperiod (dark), or known conditions to promote diapause (a). Adult females were collected from these respective conditions 6–8 days post-eclosion for downstream experiments. b Ovary dissections were performed on adult mosquitoes from each rearing condition to determine the average primary follicle size to confirm reproductive diapause. The average primary follicle size is depicted for each individual mosquito, with an average follicle size was <50 μm (dotted line) used to confirm reproductive diapause. Solid black lines represent the median values for each experimental condition, while pie charts display the percentage of mosquitoes in diapause under each condition. Additional confirmations of mosquito diapause were performed by examining differences in ovarian follicle morphology (c) and fat body lipid staining with Nile Red (d) between mosquitoes reared under control or diapause conditions. Scale bars denote 50 µm in (c) and 20 µm in (d). e Wing-length measurements on adult females were performed as a proxy body size for each condition. f Blood-feeding behavior was evaluated in adult female mosquitoes from each treatment by challenging with an artificial membrane feeder containing sheep blood (N = 3). Significance was determined in follicle size and wing-length experiments using Kruskal–Wallis with a Dunn’s post test. Blood-feeding experiments were analyzed using a one-way ANOVA test and Tukey post hoc comparisons to compare experimental treatments. Asterisks denote significance (*P < 0.05, ** P < 0.01, ***P < 0.001, ****P < 0.0001). n number of individual mosquitoes examined.
Using arrested ovarian development as a proxy for diapause induction29, we examined ovarian follicle length in individual female adult mosquitoes 6–8 days post-eclosion6,7 from each experimental condition (Fig. 1b). Interestingly, autogenous mosquitoes (displaying follicle maturation without a blood meal) were detected in all experimental conditions (Supplementary Fig. S1), suggesting that low rates of autogeny exist in our laboratory colony of Cx. pipiens. Across experimental conditions, average follicle size was comparable under the cold and dark conditions with standard laboratory rearing conditions (control), yet were significantly reduced under diapause conditions (Fig. 1b). Using a strict follicle size cutoff of ≤50 μm to designate individuals in diapause29, only the cool temperatures and short photoperiod of the “diapause condition” produced individuals in the diapause state (Fig. 1b), as demonstrated by the arrested follicle morphology in diapausing individuals (Fig. 1c). This confirms that diapause induction in our laboratory colony of Cx. pipiens requires both short photoperiod and cool temperatures similar to the previous studies6,11.
Additional previously defined physiological features of diapause10,18,30 were also explored to further validate diapause induction in our laboratory colony. This includes increased lipid accumulation (Fig. 1d)18,31, a larger body size (Fig. 1e)30, and reduced blood-feeding behavior (Fig. 1f)10, which together confirm the diapause state. Moreover, we demonstrate that our non-diapause rearing conditions can have additional influence on mosquito physiology, most notably the independent effects of temperature and short photoperiod on body size (Fig. 1e), and decreased feeding behavior under cool temperature conditions (Fig. 1f).
Evaluating natural diapause induction in semifield experiments
While laboratory studies are required to understand the physiological aspects of mosquito diapause, the environmental conditions used to promote diapause induction in the laboratory do not accurately depict the natural end-of-season conditions in temperate climates where temperatures are variable and photoperiods are less extreme than the 9-h photoperiod typically used in laboratory studies of mosquito diapause (Fig. 2a). In an effort to better understand the natural diapause conditions for Cx. pipiens, we performed a 2-year semifield study in Ames, Iowa, USA (Fig. 2b), enabling a structured approach to examine the life history conditions that result in diapause under natural conditions. To approach this question, we reared Cx. pipiens from our laboratory colony at semifield locations (Supplementary Fig. S2) from first-instar larvae to adults at different timepoints according to epidemiological week from July through late September (Fig. 2b). Initially in 2020, groups of lab-reared first-instar Cx. pipiens larvae were placed outside every 3 weeks from week 30 to week 39 (July to late September; Fig. 2b), with each group representing an approximate 1-h difference in photoperiod (Supplementary Fig. S3) ranging from ~15 to 12 h of daylight at the onset of larval development. A similar approach was employed in 2021, with experimental groups deployed at weeks 30, 33, 34, 37, 38, and 39 (Fig. 2b and Supplementary Fig. S3) to provide further resolution into the natural diapause induction timeline.
a Temperature (°C) and photoperiod (daylight) averages (2009–2019) are displayed for the study site location (Ames, IA) over the mosquito season (weeks 20–45). b Overview of the semifield experiments performed in 2020 and 2021 where first-instar larvae from a lab colony of Cx. pipiens were placed outside at weekly timepoints (between week 30 and 39). Larval groups were reared in a semifield environment, with resulting adult females used for downstream experiments 6–8 days post-eclosion. A total of 193 adult female mosquitoes were collected in 2020 (c) and 115 adult females from 2021 (d) to determine if mosquitoes were in reproductive diapause. For c and d, each dot represents the average follicle size for an individual mosquito, with a 50 μm threshold (dotted line) used to determine individuals in the diapause state. The mean follicle size (+/− SEM) is displayed for each experimental cohort. n number of individual mosquitoes examined. e The percentage of adult diapause incidence recorded by larval onset week is summarized for 2020 and 2021. The dashed line corresponds to when 50% of the mosquitoes in a given larval onset week result in reproductive diapause. From our experiments, we define an approximate period of diapause receptivity (f), where larval development under temperature and photoperiod conditions of 13.5 h of daylight and average temperatures under 20 °C may give rise to adult reproductive arrest, resulting in increasing diapause incidence (red gradient) as these environmental conditions continue to decrease over the course of the season.
To determine which mosquitoes had entered diapause, we examined ovarian follicle size as in Fig. 1. In 2020, a total of 193 adult female mosquitoes were examined from four experimental groups, of which diapause was detected in mosquitoes with a rearing onset initiated during weeks 36 and 39 (early- and late September; Fig. 2c and Supplementary Table S1). Similar results were obtained in 2021, where 115 mosquitoes across six experimental groups were examined, with diapause detected in groups with a rearing onset as early as week 34 (late August) and increasing in intensity through the remainder of the experimental timepoints initiated in September (Fig. 2d and Supplementary Table S1). For both years, diapause induction was strongest (≥50% of mosquitoes in diapause) after week 38 (late September; Fig. 2d), when immature mosquito development occurred with ~12 h of daylight and an average temperature of 15 °C (Fig. 2f), yet was readily detected in groups reared under 13.5 h of daylight and ~20 °C (Fig. 2f).
To further examine this receptive period able to promote diapause induction, we placed lab-reared pupae under semifield conditions each week from weeks 36–40 (September to early October) and evaluated their ability undergo reproductive diapause. While the transfer of lab-reared pupae to artificial diapause conditions (9:15 L:D, 19 °C) results in nearly 100% diapause induction6, under our semifield conditions, diapause induction was inefficient, with diapause only detected in low frequency (6-17%) from pupae placed outside in weeks 39 and 40 (Supplementary Fig. S4).This corresponds to the approximate environmental conditions in mid-to late September that result in ≥50% diapause induction in our larval groups (Fig. 2e), suggesting that the environmental signals during late September may be driving diapause induction in our semifield conditions. In addition, the low frequency of diapause induction at the pupal stages is suggestive that cumulative exposure over immature developmental stages may enhance diapause induction as previously suggested7.
Based on our results (Fig. 1) and other previous studies6,11, the temperature is an important, yet complex variable in the context of diapause induction. When examined between years, there is a slight variation in diapause induction (Fig. 2e), likely the result of temperature differences shifting the timing of diapause induction between years (Supplementary Figs. S3 and S5 and Supplementary Table S1). In addition, our data highlight the potential importance of diurnal fluctuations in temperature that occur during periods of diapause receptivity (Supplementary Fig. S6), where daily low temperatures may be able to sustain diapause induction signals even during the exposure to high daily temperatures (>30 °C) in our semifield studies that would typically “break” diapause induction6. Temperature also had a significant influence on mosquito development and survival as temperatures declined in late summer/early fall. As the season progressed, our experimental groups displayed increased developmental times, most notably slowing larval and pupal development, ultimately influencing adult eclosion (Supplementary Fig. S3). Moreover, in both years of our semifield study, larvae placed outside in late September (weeks 38 and 39) experienced significant mortality in immature stages due to suboptimal temperatures for mosquito development and survival, resulting in the low numbers of individual mosquitoes that contributed to our analysis of reproductive diapause (Fig. 2c). However, these impacts on survival were based only on observations and were not directly measured.
Mosquito surveillance data inform diapause induction in Iowa field populations
While our semifield study provides valuable new insight into the conditions and timing of natural diapause induction (Fig. 2), these experiments were performed using a laboratory-derived population of Cx. pipiens and may not fully capture diapause induction, such that natural field populations of mosquitoes may be more receptive to photoperiod and temperature. To measure the natural diapause timeline in populations of Cx. pipiens, we utilized long-term mosquito surveillance data from central Iowa to capture adult Cx. pipiens population trends in natural field settings.
Using different trap types to estimate general mosquito abundance (New Jersey light trap; NJLT) or blood-fed/reproductive mosquito populations (grass infusion-baited gravid traps; gravid), we examined Cx. pipiens population dynamics in central Iowa (Supplementary Fig. S7) from 2016 to 2021 as a proxy for diapause induction in natural field populations. NJLT data demonstrate early-season peaks (May/June) in Culex pipiens group32,33,34 abundance, which taper mid-summer (July) before a late-season rise in September (Fig. 3a). In contrast, gravid Cx. pipiens populations peak mid-summer (week 32), then decline by 61% by week 40 (Fig. 3b). Comparisons of NJLT and gravid population trends using a linear regression of annual slope values over weeks 30–40 were significant (P = 0.0079), supporting that only reproductive gravid Cx. pipiens populations are declining during the late summer (Supplementary Fig. S8). When placed in the context of diapause induction established in our semifield experiments (Fig. 2), gravid adult field populations experience significant declines during September, corresponding with the approximate environmental signals able to promote diapause in our semifield studies. Moreover, the cessation of mosquito surveillance after week 40 (October) tightly corresponds with the high rates of diapause in our semifield groups suggesting that after the beginning of October most emerging females will be in diapause (Fig. 3b).
Mosquito surveillance from central Iowa display mosquito populations trends that examine general population abundance (a) or represent only reproductive populations (b). Data in (a) represent Cx. pipiens group abundance measured using New Jersey Light Traps (NJLT) from 14 sites in central Iowa, while data in (b) display Gravid trap data from 16 sites in central Iowa that monitor reproductive female Cx. pipiens populations. For both a and b, collections were performed from 2016–2021 from weeks 20 to 40. Individual gray dots represent yearly trap index averages by week, with the blue (NJLT) or red (Gravid) lines representing the loess-smoothed mean and 95% confidence intervals (gray-shaded area). The approximate period of diapause receptivity (as defined by our semifield experiments) is displayed by the red gradient.
Cx. pipiens diapause incidence shares similar timelines across the United States
Based on our observations of Cx. pipiens diapause induction in semifield (Fig. 2) and natural field conditions in Iowa (Fig. 3), we wanted to similarly examine potential diapause timelines across the United States. To approach this question, mosquito surveillance data was collected from across the country (California, Colorado, Connecticut, Illinois, Minnesota, Pennsylvania, and Virginia) to examine adult Cx. pipiens population dynamics, similar to that described in Iowa (Fig. 3).
For locations with both NJLT and gravid trap types, linear regressions of annual slopes by trap type confirm that gravid trends were distinct from general population trends (NJLT) from weeks 30 to 40 at each location (Iowa, P < 0.01; California, P < 0.001; Colorado, P < 0.05; Connecticut, P < 0.0001) (Supplementary Fig. S8). When historical gravid trapping data were used to compare end-season (weeks 30–40) across the country, there was a consistent decline in Cx. pipiens gravid populations from mid-summer (July, week 30) to late summer/early fall across the United States (Fig. 4a), with the exception of gravid populations from Suffolk, Virginia which displayed a slightly increased trend in Cx. pipiens abundance (Fig. 4a). These observations coincide with the decline of gravid populations in Iowa over August and September (Fig. 3), where gravid populations in most location undergo notable declines in gravid Cx. pipiens populations from week 30 to 40 ranging from ~32 to 90% (Fig. 4b).
a End-of-season Cx. pipiens gravid trap data (weeks 30–40) provided by locations across the United States. States included in our analysis are denoted in light gray, with individual county-level data included in the statewide analysis shown in dark gray. Iowa is denoted by the red star. For each state, red lines represent the loess-smoothed mean of the gravid (reproductive) population abundance, while gray lines display data from an individual year included in our analysis. The approximate period of diapause receptivity (as defined by our semi-filed experiments) is displayed by the red gradient. b Sites at higher latitudes demonstrated more pronounced declines in gravid trap averages from weeks 30–33 to week 40. c Map of the reported distributions of Cx. pipiens (yellow), Cx. quinquefasciatus (green), and areas of potential genetic hybridization between these species (olive). Site locations providing gravid trap data are shown by the gray dots, while Iowa is denoted by the red star.
Lower latitude sites (Suffolk, Virginia, and California) (Supplementary Fig. S9) had less pronounced population declines (and even increased Cx. pipiens gravid populations; Fig. 4b), highlighting the significant influence (R2 = 0.69, P = 0.01) of latitude on end-of-season Cx. pipiens dynamics (Fig. 4b). This coincides with similar effects of latitude and photoperiod on diapause induction in other insects35,36 and mosquito species37,38. Moreover, in both Virginia and California, evidence suggests that these regions are within hybridization zones between Cx. pipiens and Culex quinquefasciatus39,40,41 (Fig. 4c), a morphologically identical species that does not undergo diapause8,30,42. As a result, the absence or low incidence of diapause in these locations may be due to the respective misidentification of Cx. quinquefasciatus as Cx. pipiens, the hybridization of these species resulting in intermediate diapause phenotypes8,30, or the potential that these could be populations of Cx. pipiens f. molestus that do not undergo reproductive diapause43.
To make comparisons of diapause-relevant environmental factors across sites, average weekly day length and average temperature values were compiled from each location (or comparably close locations) (Supplementary Fig. S10). Temperatures across all study sites noticeably declined over the 10-week period, with Minnesota (Minneapolis/St. Paul) and Colorado (Larimer County) having the coldest average temperature (~10 °C) at week 40 (Supplementary Fig. S9). Connecticut, Illinois (Chicago), and Pennsylvania displayed comparable average temperatures (~15 °C) at week 40 (Supplementary Fig. S10), similar to the average temperatures in Iowa at this timepoint (Fig. 2f). Of note, the two locations (Northern California; Suffolk, VA) with the smallest changes in gravid trap numbers (Fig. 4b), which suggest little to no diapause, had much higher temperatures across the entire 10-week period, with week 40 averages of ~20 °C (Supplementary Fig. S10). Although average day lengths varied slightly at the start (week 30), with the highest latitude location (Minnesota) having slightly longer day lengths, all included locations converged to near identical levels (~12 h) by the fall equinox (week ~38).
Our nationwide gravid trap data also allude to the influence of elevation in Cx. pipiens diapause induction, where surveillance data from Colorado display sharp declines in gravid populations beginning in late August (week 34) and the termination of surveillance activities only weeks later (week 37; Fig. 4a). This accelerated timeline suggests that the high altitude of Larimer County, CO (Supplementary Fig. S9) enhanced the environmental signals that promote diapause induction, similar to that described in other mosquito species37,44.
Discussion
Although diapause is a critical component to the success of Culex pipiens overwintering survival in temperate regions, our understanding of the environmental signals that promote diapause induction under natural conditions has thus far been limited. Using laboratory and semifield experiments to inform historical mosquito surveillance trends from across the county, we provide a definitive diapause induction timeline broadly shared in true Cx. pipiens populations across the United States. While temperature and photoperiod are integral components of diapause induction in laboratory and field conditions, our data suggest that latitude and elevation can further amplify the effects of temperature and photoperiod under natural conditions, as well as highlight the importance of the influence Culex population genetics in defining diapause incidence (Fig. 5).
There are many physiological and behavioral changes associated with diapause10,11,13,31,45, yet the key feature to confirm reproductive diapause in Cx. pipiens is an arrested ovarian development phenotype11,46,47. Morphologically this corresponds to arrested ovarian follicle development, in which follicles lack yolk granulation and become stunted in size48. Arrested ovarian development has also been quantified using either direct measurements of the primary follicle5,18,25,29,30, or the size ratio of the primary follicle to the secondary follicle6,42,46,49 to establish diapause. In our experiments, we relied on measurements of the primary follicle to determine reproductive diapause. Previous experiments have defined diapause using this methodology with follicle lengths ranging from 30 to 70 µm5,18,25,29,30,49. However, our Cx. pipiens colony produced primary follicle lengths of ~75 µm under standard rearing conditions. As a result, a more conservative cutoff of ≤50 µm was used to confirm reproductive diapause in our lab colony at the risk of potentially excluding some individual mosquitoes that did not meet these strict criteria in our laboratory and semifield experiments.
In laboratory experiments using an established colony of Cx. pipiens, we observed a relatively low frequency of diapause induction when we applied our strict criteria for ovarian arrest. Although diapause is genetically determined (reviewed in ref. 48), our colony of Cx. pipiens has been maintained in artificial laboratory conditions after its initial colonization (~2005) without re-invigoration from wild-caught specimens or artificial diapause cycling29. As a result, our laboratory colony may have become desensitized to the thermal and photoperiod cues required to promote diapause, similar to the influence of artificial rearing conditions on diapause incidence in other insect species50,51,52. In addition, our laboratory diapause induction experiments used a fluorescent light source, which previous studies have suggested is less efficient than incandescent light at promoting diapause in Cx. pipiens8. While at present we cannot provide a clear explanation for the low rates of diapause induction in our laboratory experiments, the same lab colony was used in our semifield experiments where diapause incidence reached in excess of 50%, suggesting that the predisposition for diapause remains in our Cx. pipiens colony. Factors such as the length of crepuscular periods, more extreme low daily temperatures, or diurnal temperature fluctuations may be important natural variables that can overcome diapause de-sensitivity resulting from long-term colonization.
While short photoperiod (9:15 L:D) and cool temperatures (19 °C) are traditionally used to initiate diapause in the laboratory26,28,53, these experimental conditions do not accurately reflect the natural onset of diapause in the field. Photoperiod values only vaguely provide a timeline of diapause potential between the summer solstice (15 h of light) where low-level diapause is possible6,21 and the winter solstice (9 h of light) where temperatures prove impossible for mosquito survival and development in temperate climates. Similarly, as temperatures can subvert diapause induction6, the potential for natural fluctuations in the end-season may affect presumed diapause timelines derived from stable lab combinations of photoperiod and temperature. However, only a limited number of studies have examined Cx. pipiens diapause in field or semifield settings21,27,49.
Through our semifield studies conducted over a 2-year period (2020–2021), we systematically reared mosquitoes over weeks 30–40 to capture the natural conditions that promote diapause induction in Cx. pipiens. From these experiments, we define a timeline of brood receptivity to diapause beginning in late August (week 34) when immature mosquitoes have the potential to emerge in adult diapause. With increasing diapause incidence as the season progresses, our data support that there is a critical field photoperiod in mid-September (week 38) where ~50% of the immature Cx. pipiens population reared at this time emerged in the adult diapause state. This closely coincides with the results of a previous semifield study in Boston, USA, where peak diapause incidence occurred in late September and early October27. While diapause can be induced in pupae under artificial diapause conditions6, the low occurrence of pupal diapause in our semifield study during these peak periods of diapause induction suggests that cumulative environmental signals experienced over all immature stages may enhance diapause incidence as previously proposed7.
While our semifield experiments are informative in exploring natural diapause induction, factors such as the gaps between experimental cohorts and the reliance on a laboratory colony of Cx. pipiens are known limitations. To overcome this, we employed the use of Cx. pipiens surveillance data as a proxy for adult diapause incidence21. Through the use of a multi-year dataset that captured long-term weekly adult abundance, we demonstrate that gravid female mosquito populations begin to decline in August until they are effectively depleted by October. Although some of these trends will be in part from encroaching cold temperatures that reduce mosquito abundance54 and blood-feeding behavior (as demonstrated in our laboratory experiments), these observations closely align with the natural diapause conditions defined in our semifield study. Initially focused on surveillance data from Iowa, additional data from across the country provided an opportunity to study diapause timelines in a larger context, where surveillance data at the national scale revealed that latitude, elevation, and Culex population genetics may influence Culex diapause incidence in addition to temperature and photoperiod.
When our data are placed in the context of the wide geographic range within the United States, the importance of latitudinal patterns on diapause incidence begin to emerge for Cx. pipiens, where we identify a gradient in the effects of latitude and elevation on diapause incidence, similar to that described in other mosquito species37,44,55,56. Moreover, our data suggest that Culex population genetics may also have significant influence on diapause induction where Cx. pipiens hybridization with the morphologically indistinguishable Cx. quinquefasciatus may result in intermediate diapause phenotypes30. Together with the potential of non-diapausing Cx. pipiens f. molestus populations, the genetics of local Culex pipiens s.l. populations may contribute to the absence or low incidence of diapause in a given location, where only genetically pure populations of Cx. pipiens pipiens enter reproductive diapause6,49. However, only limited studies have examined Cx. pipiens s.l. population genetics in the United States39,57,58,59,60,61,62,63, highlighting the important need to better define these important vector species. Of note, few pure Cx. pipiens pipiens were detected in northern California58,64, which may account for the weak population declines observed in the gravid trap data from California. This coincides with previous observations in northern California that Cx. pipiens s.l. do not enter diapause49. The population genetics of Cx. pipiens s.l. in Virginia have not been previously examined, yet due to the geographic location, there is likely hybridization within the Cx. pipiens complex that may similarly partially account for the observations in the gravid trap data.
Although temperature is considered an important signal for diapause induction, its contributions to diapause have primarily been evaluated under stable conditions in the laboratory, with little insight into weekly temperature variability and daily temperature fluctuations that occur in nature. As a result, identifying periods of diapause receptivity to temperature alone is difficult, especially when temperature can influence Culex species immature development times65,66, as well as adult survival, blood-feeding, fecundity, and abundance54,66. Similar to these experiments, we observed extended larval development times with cooler average temperatures, which may allow for the increased accumulation of lipids in subsequent diapausing adults18. However, as temperatures continue to drop in late summer and early fall when developing larvae can emerge in diapause, there is a tight balance between accumulating enough resources to enter diapause and not being able to survive temperatures that do not allow for further development. As such, temperature can be a highly confounding variable, one that likely accounts for small variations in the timing of diapause induction between years.
An additional, often overlooked aspect of temperature is the influence of daily temperature fluctuations on mosquito physiology. In Aedes aegypti, large diurnal temperature ranges negatively impact mosquito development67, adult female fecundity67, and vector competence68. Given that diurnal temperature ranges are largest in temperate climates during the late summer and early fall when mosquitoes are receptive to diapause, we hypothesize that these daily temperature fluctuations may similarly influence Culex physiology and diapause induction. Moreover, daily temperature fluctuations may protect the commitment to diapause if diapausing adults are exposed to higher temperatures that would regularly break reproductive diapause under laboratory conditions5.
Although long inferred, the relationship of diapause induction to the cessation of West Nile virus (WNV) activity has yet to be fully explored. Our data provide strong support that the diapause incidence in the late summer/early fall coincides with the dramatic decline of human WNV cases and mosquito infection rates in late September and early October in temperate regions of the United States32,69,70. Moreover, since diapausing females do not blood feed10, it argues that the overwintering of WNV in Cx. pipiens71,72,73,74 occurs via vertical transmission as previously suggested75,76. Coincidently, the period of diapause receptivity in the late summer and early fall also corresponds the peak of WNV mosquito infection rates32. Although vertical transmission is an inefficient process71,72,73,74, the increased prevalence of WNV infection in mosquito populations during the approximate time when conditions are favorable for diapause induction may enhance WNV overwintering in diapausing female mosquitoes. As a result, measures to control Culex populations prior to diapause induction may not only reduce mosquito populations in the following spring as previously suggested77, but also limit WNV overwintering and subsequent disease burdens in the following season.
In summary, our findings provide a definitive examination of diapause induction in Cx. pipiens supported by laboratory, semifield, and field-collected surveillance data from across the United States. We demonstrate the dynamic nature of diapause ecology influenced by yearly variation in temperature, as well as the effects of latitude, elevation, and mosquito population genetics that ultimately determine the overall end-season population structure of Cx. pipiens and its role in WNV transmission. Taken together, these data demonstrate the importance of mosquito diapause in defining periods of mosquito-borne disease transmission in the United States. With evidence suggesting that rising global temperatures can alter diapause incidence78, the effects of climate change may extend transmission seasons and increase the incidence of mosquito-borne disease in temperate regions throughout the world78,79,80.
Methods
Mosquito rearing
A laboratory colony of Cx. pipiens mosquitoes originally isolated from field collections in Ames, Iowa has been constantly maintained in Iowa State University’s Insectary at 25 °C, 85% RH, and 16:8 (L:D) on 10% sucrose ad libitum since ~2005. Larvae were fed using a 50/50 mix of crushed Milk-Bone® and Tetramin® fish food, while commercial sheep blood (Hemostat Laboratories) was used for egg production.
Laboratory diapause induction experiments
Laboratory experiments to examine diapause induction were performed by placing newly hatched first-instar mosquitoes in Percival incubators where they were reared under different experimental conditions (Control: 25 °C, 16:8 (L:D); Cold: 19 °C, 16:8 (L:D); Dark: 25 °C, 9:15 (L:D); Diapause: 19 °C, 9:15 (L:D)) to examine the independent and combined influence of temperature and photoperiod on diapause induction. Experimental conditions were selected based on the previous studies10,26,30 in which diapause was induced by a short photoperiod (9:15) and cool temperatures (19 °C). Data from all experiments were collected from three or more independent biological replicates.
Confirmation of reproductive diapause
Ovaries were dissected from females aged 6–8 days in 1% PBS solution and mounted with Aqua-Poly/Mount (Polysciences Inc). To confirm reproductive diapause, primary follicle lengths were measured under ×200 magnification using an Olympus BX40 compound microscope according to ovary morphology as previously defined28,29. Ten measurements per ovary were recorded to calculate an average follicle length per individual. Individuals with average follicle size under 50 μm were defined as being in diapause29.
Wing-length measurements
Measurements of wing length served as a proxy for mosquito body size81,82, where the right wing was dissected and measured from the alula to the most distal tip of the wing under ×10 magnification using a dissecting microscope and Nikon imaging software (NIS Elements D 3.2).
Blood-feeding behavior
Approximately 20–30 adult females (6–8 days post-eclosion) from each experimental rearing condition, as described above, were challenged with defibrinated sheep blood (Hemostat Laboratories) using an artificial membrane system. After approximately 1 h, the number of mosquitoes with a visible blood meal were recorded (of the total) to calculate the percentage of mosquitoes taking a blood meal. Experiments were performed in three independent biological experiments.
Lipid staining
Nile Red (Thermo Fisher Scientific) was used to visualize differences in lipid stores of adult females (6–9 days old) reared under diapause (19 °C, 9:15 L:D) and control conditions (25 °C, 16:8 L:D). Fat bodies were dissected in 4% paraformaldehyde and stained using a 1:100 PBS dilution of 500 μg/ml Nile Red powder in acetone stock as previously described18,31. After incubating tissues for 10 min, samples were imaged using a Nikon 50i fluorescent microscope and processed with Nikon imaging software (NIS Elements D 3.2).
Semifield studies of diapause induction
To examine diapause induction in a natural setting, first-instar larvae from our laboratory colony were concurrently placed at three locations in Ames, Iowa, in 2020 (three replicates) and two locations in 2021 (two replicates, summarized in Supplementary Fig. S2) to mimic the emergence of mosquito populations at different timepoints throughout the season. In 2020, batches were placed outside every 3 weeks according to epidemiological week, from week 30 (July 19th) to week 39 (September 20th), approximately corresponding to a 1-h loss in daylight between each group. In 2021, batches were again placed outside at weeks 30, 33, and 39 to replicate experiments from the previous year. Unfortunately, larvae were not available to repeat the week 36 timepoint in 2021, however additional groups were included from weeks 34, 37, and 38 to provide additional resolution to diapause induction. Larval density in each batch was ~300–400 per tray in 1 L of distilled water. Mosquitoes were fed daily with 50 mg of a 50/50 mixture of Milk-Bone® mix and Tetramin® fish food. Upon pupation, pupae were placed into mosquito breeder eclosion chambers (BioQuip), with adults were provided with 10% sucrose ad libitum for 6–8 days before collections to determine diapause incidence. Samples from each site location (replicates) were pooled for each experimental cohort (week) to examine follicle size and diapause induction. In addition, lab-reared pupae were placed outside in mosquito breeder eclosion chambers in 2021 from weeks 37 to 40 to compare diapause induction rates between mosquito life stages.
Mosquito population trends in Iowa
Mosquito surveillance was performed in two central Iowa counties (Polk, Story) by Iowa State University personnel or local public health partners from mid-May (week 20) through the first week of October (week 40). Mosquito collections were performed using infusion-baited Frommer Updraft Gravid Traps (John W. Hock Company) targeting gravid adult female mosquitoes at 16 sites over a six-year period (2016–2021; Supplementary Fig. S6). In addition, a total of 14 New Jersey light traps (NJLTs) were used to measure mosquito abundance (2016–2021). Trapping sites (gravid or NJLT) with less than three years of mosquito data were excluded from the study. All mosquito samples were identified using morphological keys40,83 where possible. Due to damage to morphological features that help to define Culex species84, mosquito identifications of Culex pipiens and Culex restuans from NJLT traps were defined as Culex pipiens group as previously32,34. Gravid trap specimens were identified to species (Culex pipiens)83. To normalize trapping efforts, raw mosquito counts were converted to a trap index (defined as the number of mosquitoes collected/number of trapping nights) and then averaged by week.
Iowa climate and photoperiod data
Daily temperature data (°C) was collected and averaged into weekly values by year using the Iowa Environmental Mesonet (https://mesonet.agron.iastate.edu) for the Southeast Ames station (IA0203). Photoperiod data for the duration of the study period was collected as hours of daylight for Des Moines, Iowa from daylight tables provided from an online Sunrise and Sunset table (www.timeanddate.com).
National trends in diapause induction, temperature, and Culex species hybridization
Mosquito surveillance data were provided from additional locations across the United States (California; https://vectorsurv.org/, Colorado, Connecticut, Illinois, Minnesota, Pennsylvania, and Virginia), representing the temperate range of Cx. pipiens in the United States40. The years included from each dataset are as follows: California (2005–2020), Colorado (2015–2020), Connecticut (2006–2020), Illinois (2016–2020), Minnesota (2006–2020), Pennsylvania (2007–2017), and Virginia (2010–2020). To reflect the end-of-season population trends, data was trimmed to reflect weeks 30–40 where applicable (Colorado ends at week 37, Chicago ended most weeks at 39), with raw mosquito counts normalized using trap index averages to account for differences in trapping efforts (number of mosquitoes/numbers of trapping nights) as previously performed for the Iowa dataset. Data from Illinois and Minnesota represent Culex pipiens group (a combination of the morphologically similar Culex restuans and Cx. pipiens species)32,33, while records from other states were morphologically identified as Cx. pipiens.
Daily high- and low temperatures for all site locations were compiled using the Iowa Environmental Mesonet (https://mesonet.agron.iastate.edu). All locations reflect 10-year averages (2010–2020) where environmental data were paired to trapping locations as follows: California (Sacramento, CATSAC), Connecticut (statewide average, CT0000), Colorado (east Fort Collins, CO3006), Illinois (Chicago O’Hare International airport, ILTORD), Minnesota (Minneapolis-St. Paul, MNTMSP), Pennsylvania (southeastern PA, PAC003), and Virginia (Suffolk, VA8192). Photoperiod data was collected from an online Sunrise and Sunset table (www.timeanddate.com) for field locations in California (Sacramento), Colorado (Fort Collins), Connecticut (New Haven), Illinois (Chicago), Minnesota (Minneapolis), Pennsylvania (Philadelphia), and Virginia (Chesapeake) for 2021.
Elevation data for all site locations are provided as a county-level average value collected using https://en-gb.topographic-map.com/.
Graphical mapping
The topographical map of the United States highlighting counties with contributing mosquito abundance data was generated in R version 4.1.3 (R Development Core Team) using the “usmap” and “ggplot” packages.
The proposed ranges of Cx. pipiens and Culex quinquefasciatus, as well as proposed areas of hybridization, were created from a base map of the United States obtained in ArcGIS (https://www.arcgis.com/home/index.html), with mosquito ranges adapted from Darsie and Ward40 and illustrated using Inkscape (https://inkscape.org/).
Statistics and reproducibility
Laboratory comparisons comparing ovarian follicle size and wing length were analyzed using Kruskal–Wallis with a Dunn’s post test, while the percentage of blood-feeding across experimental groups was examined using a one-way ANOVA with a Tukey post hoc analysis. All statistical analyses were performed using GraphPad Prism 7.0. Average weekly temperature data were visualized using loess smoothing in R (version 3.6.3). Gravid and NJLT population trends for weeks 30–40 were examined for the Iowa dataset and other locations where NJLT data were provided using yearly slope values and negative binomial regressions with an unpaired t test to demonstrate significant differences in the dynamics of gravid populations. Mosquito population trends were also evaluated by calculating the difference in the average trap index at weeks 30–33 and week 40, and displaying these trends as a percent change ((week 40 − weeks 30–33)/weeks 30–33) ×100) value to demonstrate changing temporal trends in gravid populations from mid-season to end-season timepoints.
Reporting summary
Further information on research design is available in the Nature Portfolio Reporting Summary linked to this article.
References
Way, M. J., Hopkins, B. & Smith, P. M. Photoperiodism and diapause in insects. Nature 164, 615 (1949).
Beck, S. Photoperiod induction of diapause in an insect. Biol. Bull. 122, 1–12 (1962).
Denlinger, D. L. & Armbruster, P. A. Mosquito diapause. Annu. Rev. Entomol. 59, 73–93 (2014).
Readio, J., Chen, M. H. & Meola, R. Juvenile hormone biosynthesis in diapausing and nondiapausing Culex pipiens (Diptera: Culicidae). J. Med. Entomol. 36, 355–360 (1999).
Eldridge, B. F. & Bailey, C. L. Experimental hibernation studies in Culex pipiens (Diptera: Culicidae): reactivation of ovarian development and blood-feeding in prehibernating females. J. Med Entomol. 15, 462–467 (1979).
Spielman, A. & Wong, J. Environmental control of ovarian diapause in Culex pipiens. Ann. Entomol. Soc. Am. 66, 905–907 (1973).
Sanburg, L. L. & Larsen, J. R. Effect of photoperiod and temperature on ovarian development in Culex pipiens pipiens. J. Insect Physiol. 19, 1173–1190 (1973).
Eldridge, B. F. The effect of temperature and photoperiod on blood-feeding and ovarian development in mosquitoes of the Culex pipiens complex. Am. J. Trop. Med. Hyg. 17, 133–140 (1968).
Bowen, M. F. Patterns of sugar feeding in diapausing and nondiapausing Culex pipiens (Diptera: Culicidae) females. J. Med. Entomol. 29, 843–849 (1992).
Robich, R. M. & Denlinger, D. L. Diapause in the mosquito Culex pipiens evokes a metabolic switch from blood feeding to sugar gluttony. Proc. Natl Acad. Sci. USA 102, 15912–15917 (2005).
Eldridge, B. F. Environmental control of ovarian development in mosquitoes of the Culex pipiens complex. Am. Assoc. Adv. Sci. 151, 826–828 (1966).
Vinogradova, A. B. Culex pipiens Pipiens Mosquitoes: Taxonomy, Distribution, Ecology, Physiology, Genetics, Applied Importance And Control (Pensoft, 2000).
Benoit, J. B. & Denlinger, D. L. Suppression of water loss during adult diapause in the northern house mosquito, Culex pipiens. J. Exp. Biol. 210, 217–226 (2007).
Li, A. & Denlinger, D. L. Pupal cuticle protein is abundant during early adult diapause in the mosquito Culex pipiens. J. Med. Entomol. 46, 1382–1386 (2009).
Yang, L., Denlinger, D. L. & Piermarini, P. M. The diapause program impacts renal excretion and molecular expression of aquaporins in the northern house mosquito, Culex pipiens. J. Insect Physiol. 98, 141–148 (2017).
King, B., Li, S., Liu, C., Kim, S. J. & Sim, C. Suppression of glycogen synthase expression reduces glycogen and lipid storage during mosquito overwintering diapause. J. Insect Physiol. 120, 103971 (2020).
Sim, C. & Denlinger, D. L. Transcription profiling and regulation of fat metabolism genes in diapausing adults of the mosquito Culex pipiens. Physiol. Genomics 39, 202–209 (2009).
Sim, C. & Denlinger, D. L. Insulin signaling and FOXO regulate the overwintering diapause of the mosquito Culex pipiens. Proc. Natl Acad. Sci. USA 105, 6777–6781 (2008).
Zhou, G. & Miesfeld, R. L. Energy metabolism during diapause in Culex pipiens mosquitoes. J. Insect Physiol. 55, 40–46 (2009).
Chang, J. et al. Solid-state NMR reveals differential carbohydrate utilization in diapausing Culex pipiens. Sci. Rep. 6, 37350 (2016).
Madder, D. J., Surgeoner, G. A. & Helson, B. V. Induction of diapause in Culex pipiens and Culex restuans (Diptera: Culicidae) in Southern Ontario. Can. Entomol. 115, 877–883 (1983).
Spielman, A. Effect of synthetic juvenile hormone on ovarian diapause of Culex pipiens mosquitoes. J. Med. Entomol. 11, 223–225 (1974).
Sim, C. & Denlinger, D. L. Insulin signaling and the regulation of insect diapause. Front. Physiol. 4, 189 (2013).
Robich, R. M., Rinehart, J. P., Kitchen, L. J. & Denlinger, D. L. Diapause-specific gene expression in the northern house mosquito, Culex pipiens L., identified by suppressive subtractive hybridization. J. Insect Physiol. 53, 235–245 (2007).
Sim, C., Kang, D. S., Kim, S., Bai, X. & Denlinger, D. L. Identification of FOXO targets that generate diverse features of the diapause phenotype in the mosquito Culex pipiens. Proc. Natl Acad. Sci. USA 112, 3811–3816 (2015).
Kang, D. S., Cotten, M. A., Denlinger, D. L. & Sim, C. Comparative transcriptomics reveals key gene expression differences between diapausing and non-diapausing adults of Culex pipiens. PLoS ONE 11, e0154892 (2016).
Spielman, A. Structure and seasonality of nearctic Culex pipiens populations. Ann. N. Y. Acad. Sci. 951, 220–234 (2001).
Wilton, D. P. & Smith, G. C. Ovarian diapause in three geographic strains of Culex pipiens (Diptera: Culicidae). J. Med. Entomol. 22, 524–528 (1985).
Eldridge, B. F. Diapause and related phenomena in Culex mosquitoes: their relation to arbovirus disease ecology. In: Current Topics in Vector Research (ed. Harris, K. F.) 1–28 (Springer, 1987).
Meuti, M. E., Short, C. A. & Denlinger, D. L. Mom matters: diapause characteristics of Culex pipiens-Culex quinquefasciatus (Diptera: Culicidae) hybrid mosquitoes. J. Med. Entomol. 52, 131–137 (2015).
Zhang, C. et al. Understanding the regulation of overwintering diapause molecular mechanisms in Culex pipiens pallens through comparative proteomics. Sci. Rep. 9, 6845 (2019).
Dunphy, B. M. et al. Long-term surveillance defines spatial and temporal patterns implicating Culex tarsalis as the primary vector of West Nile virus. Sci. Rep. 9, 6637 (2019).
Dunphy, B. M., Rowley, W. A. & Bartholomay, L. C. A Taxonomic checklist of the mosquitoes of Iowa. J. Am. Mosq. Control Assoc. 30, 119–121 (2014).
Sucaet, Y., Van Hemert, J., Tucker, B. & Bartholomay, L. C. A web-based relational database for monitoring and analyzing mosquito population dynamics. J. Med. Entomol. 45, 775–784 (2008).
Ryan, S. F., Valella, P., Thivierge, G., Aardema, M. L. & Scriber, J. M. The role of latitudinal, genetic and temperature variation in the induction of diapause of Papilio glaucus (Lepidoptera: Papilionidae). Insect Sci. 25, 328–336 (2018).
Huang, L. et al. Diapause incidence and critical day length of Asian corn borer (Ostrinia furnacalis) populations exhibit a latitudinal cline in both pure and hybrid strains. J. Pest Sci. 93, 559–568 (2020).
Bradshaw, W. E. Geography of photoperiodic response in diapausing mosquito. Nature 262, 384–386 (1976).
Bradshaw, W. E. & Lounibos, L. P. Evolution of dormancy and its photoperiodic control in pitcher-plant mosquitoes. Nature 31, 546–567 (1977).
Kothera, L., Zimmerman, E. M., Richards, C. M. & Savage, H. M. Microsatellite characterization of subspecies and their hybrids in Culex pipiens complex (Diptera: Culicidae) mosquitoes along a North-South transect in the central United States. J. Med. Entomol. 46, 236–248 (2009).
Darsie, R. F. R. & Ward, R. A. R. Identification and Geographical Distribution of the Mosquitoes of North America, North of Mexico (University Press of Florida, 2005).
Huang, S., Molaei, G. & Andreadis, T. G. Reexamination of Culex pipiens hybridization zone in the eastern United States by ribosomal DNA-based single nucleotide polymorphism markers. Am. J. Trop. Med. Hyg. 85, 434–441 (2011).
Reisen, W. K. Overwintering studies on Culex tarsalis (Diptera: Culicidae) in Kern County, California: life stages sensitive to diapause induction cues. Ann. Entomol. Soc. Am. 79, 674–676 (1986).
Haba, Y. & McBride, L. Origin and status of Culex pipiens mosquito ecotypes. Curr. Biol. 32, R237–R246 (2022).
Holzapfel, C. M. & Bradshaw, W. E. Geography of larval dormancy in the tree-hole mosquito, Aedes triseriatus (Say). Can. J. Zool. 59, 1014–1021 (1981).
Rinehart, J. P., Robich, R. M. & Denlinger, D. L. Enhanced cold and desiccation tolerance in diapausing adults of Culex pipiens, and a role for Hsp70 in response to cold shock but not as a component of the diapause program. J. Med. Entomol. 43, 713–722 (2006).
Faraji, A. & Gaugler, R. Experimental host preference of diapause and non-diapause induced Culex pipiens pipiens (Diptera: Culicidae). Parasit. Vectors 8, 389 (2015).
Washino, R. K. The physiological ecology of gonotrophic dissociation and related phenomena in mosquitoes. J. Med. Entomol. 13, 381–388 (1977).
Christophers, S. The development of the egg follicle in Anophelines. Paludism 1, 73–88 (1911).
Nelms, B. M., Macedo, P. A., Kothera, L., Savage, H. M. & Reisen, W. K. Overwintering biology of Culex (Diptera: Culicidae) mosquitoes in the Sacramento Valley of California. J. Med. Entomol. 50, 773–790 (2013).
Diniz, D. F. A., De Albuquerque, C. M. R., Oliva, L. O., De Melo-Santos, M. A. V. & Ayres, C. F. J. Diapause and quiescence: dormancy mechanisms that contribute to the geographical expansion of mosquitoes and their evolutionary success. Parasites Vectors 10, 1–13 (2017).
Kingsolver, J. G. & Nagle, A. Evolutionary divergence in thermal sensitivity and diapause of field and laboratory populations of Manduca sexta. Physiol. Biochem. Zool. 80, 473–479 (2007).
Brent, C. S. & Spurgeon, D. W. Diapause response of laboratory reared and native lygus hesperus knight (Hemiptera: Miridae). Environ. Entomol. 40, 455–461 (2011).
Rinehart, J. P., Yocum, G. D., Leopold, R. A. & Robich, R. M. Cold storage of Culex pipiens in the absence of diapause. J. Med. Entomol. 47, 1071–1076 (2014).
Arora, A. K., Sim, C., Severson, D. W. & Kang, D. S. Random forest analysis of impact of abiotic factors on Culex pipiens and Culex quinquefasciatus occurrence. Front. Ecol. Evol. 9, 773360 (2022).
Focks, D. A., Linda, S. B., Craig Jnr, G. B., Hawley, W. A. & Pumpuni, C. B. Aedes albopictus (Diptera: Culicidae): a statistical model of the role of temperature, photoperiod, and geography in the induction of egg diapause. J. Med. Entomol. 31, 278–286 (1994).
Urbanski, J. et al. Rapid adaptive evolution of photoperiodic response during invasion and range expansion across a climatic gradient. Am. Nat. 179, 490–500 (2012).
Kothera, L., Godsey, M. S., Doyle, M. S. & Savage, H. M. Characterization of Culex pipiens complex (Diptera: Culicidae) populations in Colorado, USA using microsatellites. PLoS ONE 7, e0047602 (2012).
Kothera, L., Nelms, B. M., Reisen, W. K. & Savage, H. M. Population genetic and admixture analyses of Culex pipiens complex (Diptera: Culicidae) populations in California, United States. Am. J. Trop. Med. Hyg. 89, 1154–1167 (2013).
Kothera, L. et al. Bloodmeal, Host selection, and genetic admixture analyses of Culex pipiens Complex (Diptera: Culicidae) mosquitoes in Chicago, IL. J. Med. Entomol. 57, 78–87 (2020).
Huang, S., Molaei, G. & Andreadis, T. G. Genetic insights into the population structure of Culex pipiens (Diptera: Culicidae) in the Northeastern United States by using microsatellite analysis. Am. J. Trop. Med Hyg. 79, 518–527 (2008).
Barr, A. R. The Distribution of Culex p. pipiens and Cp quinquefasciatus in North America. Am. J. Trop. Med. Hyg. 6, 153–165 (1957).
Iltis, W. G. Biosystematics of the Culex pipiens Complex in Northern California. Thesis, University of California, Davis. (1966).
Urbanelli, S., Silvestrini, F., Reisen, W. K., De Vito, E. & Bullini, L. Californian hybrid zone between Culex pipiens pipiens and Cx. p. quinquefasciatus revisited (Diptera: Culicidae). J. Med. Entomol. 34, 116–127 (1997).
Nelms, B. M. et al. Phenotypic variation among Culex pipiens complex (Diptera: Culicidae) populations from the Sacramento Valley, California: Horizontal and vertical transmission of West Nile virus, diapause potential, autogeny, and host selection. Am. J. Trop. Med. Hyg. 89, 1168–1178 (2013).
Dodson, B. L., Kramer, L. D. & Rasgon, J. L. Effects of larval rearing temperature on immature development and West Nile virus vector competence of Culex tarsalis. Parasit. Vectors 5, 199 (2012).
Ciota, A. T., Matacchiero, A. C., Marm Kilpatrick, A. & Kramer, L. D. The effect of temperature on life history traits of Culex mosquitoes. J. Med Entomol. 51, 55–62 (2014).
Carrington, L. B., Seifert, S. N., Willits, N. H., Lambrechts, L. & Scott, T. W. Large diurnal temperature fluctuations negatively influence Aedes aegypti (Diptera: Culicidae) life-history traits. J. Med. Entomol. 50, 43–51 (2013).
Lambrechts, L. et al. Impact of daily temperature fluctuations on dengue virus transmission by Aedes aegypti. Proc. Natl Acad. Sci. USA 108, 7460–7465 (2011).
Karki, S., Brown, W. M., Uelmen, J., O’Hara Ruiz, M. & Smith, R. L. The drivers of West Nile virus human illness in the Chicago, Illinois, USA area: fine scale dynamic effects of weather, mosquito infection, social, and biological conditions. PLoS ONE 15, e0227160 (2020).
Andreadis, T. G., Anderson, J. F., Vossbrinck, C. R. & Main, A. J. Epidemiology of West Nile virus in Connecticut: a five-year analysis of mosquito data 1999–2003. Vector-Borne Zoonotic Dis. 4, 360–378 (2004).
Anderson, J. F. & Main, A. J. Importance of vertical and horizontal transmission of West Nile virus by Culex pipiens in the northeastern United States. J. Infect. Dis. 194, 1577–1579 (2006).
Nasci, R. S. et al. West Nile virus in overwintering Culex mosquitoes, New York City, 2000. Emerg. Infect. Dis. 7, 742–744 (2001).
Kampen, H., Tews, B. A. & Werner, D. First evidence of West Nile virus overwintering in mosquitoes in Germany. Viruses 13, 1–7 (2021).
Farajollahi, A. et al. Detection of West Nile viral RNA from an overwintering pool of Culex pipens pipiens (Diptera: Culicidae) in New Jersey, 2003. J. Med. Entomol. 42, 490–494 (2005).
Baqar, S., Hayes, C. G., Murphy, J. R. & Watts, D. M. Vertical transmission of West Nile virus by Culex and Aedes species mosquitoes. Am. J. Trop. Med. Hyg. 48, 757–762 (1993).
Miller, B. R. et al. First field evidence for natural vertical transmission of West Nile virus in Culex univittatus complex mosquitoes from Rift Valley Province, Kenya. Am. J. Trop. Med. Hyg. 62, 240–246 (2000).
Peffers, C. S., Pomeroy, L. W. & Meuti, M. E. Critical photoperiod and its potential to predict mosquito distributions and control medically important pests. J. Med. Entomol. 58, 1610–1618 (2021).
Bradshaw, W. E. & Holzapfel, C. M. Genetic shift in photoperiodic response correlated with global warming. Proc. Natl Acad. Sci. USA 98, 14509–14511 (2001).
Reiter, P. Climate change and mosquito-borne disease. Environ. Health Perspect. 109, 141–161 (2001).
Colón-González, F. J. et al. Projecting the risk of mosquito-borne diseases in a warmer and more populated world: a multi-model, multi-scenario intercomparison modelling study. Lancet Planet. Heal. 5, e404–e414 (2021).
Barreaux, A. M. G., Stone, C. M., Barreaux, P. & Koella, J. C. The relationship between size and longevity of the malaria vector Anopheles gambiae (s.s.) depends on the larval environment. Parasites Vectors 11, 485 (2018).
Van Handel, E. & Day, J. F. Correlation between wing length and protein content of mosquitoes. J. Am. Mosq. Control Assoc. 5, 180–182 (1989).
Ferreira-De-Freitas, L., Thrun, N. B., Tucker, B. J., Melidosian, L. & Bartholomay, L. C. An evaluation of characters for the separation of two Culex species (Diptera: Culicidae) based on material from the Upper Midwest. J. Insect Sci. 20, 21 (2020).
Harrington, L. C. & Poulson, R. L. Considerations for accurate identification of adult Culex restuans (Diptera: Culicidae) in field studies. J. Med. Entomol. 45, 1–8 (2008).
Acknowledgements
We would like to thank Julie Coughlin of the Iowa Department of Public Health and the many local public health partners that contributed to our mosquito trapping efforts. We would like to thank Dr. Philip Dixon for assistance in our statistical analysis, Dr. James Klimavicz for coding and statistics help, and Dr. Ryan Tokarz for creating the initial map of Culex species distributions. Data from California were provided through CalSurv data request #48 (https://vectorsurv.org). This research was supported by the USDA National Institute of Food and Agriculture, Hatch Project 101071, the Epidemiology and Laboratory Capacity for Infectious Diseases (ELC) Program through the Iowa Department of Public Health, and the Midwest Center of Excellence for Vector-Borne Disease. This publication was supported by Cooperative Agreement #U01 CK000505, funded by the Centers for Disease Control and Prevention. CMB acknowledges support from the Pacific Southwest Center of Excellence in Vector-Borne Diseases funded by the U.S. Centers for Disease Control and Prevention (#1U01CK000516). Its contents are solely the responsibility of the authors and do not necessarily represent the official views of the Centers of Disease Control and Prevention or the Department of Health and Human Services.
Author information
Authors and Affiliations
Contributions
E.N.F. and R.C.S. designed the research, E.N.F. and R.C.S. performed the research, analysis, and data visualization, J.J.S., M.C.E., K.J.P., B.J.W., K.J., B.B., C.A., G.D.E., P.M.A., C.M.B., and R.C.S. contributed surveillance data, E.N.F. and R.C.S. wrote the initial draft of the manuscript, with all authors contributing to revisions of the final manuscript.
Corresponding author
Ethics declarations
Competing interests
The authors declare no competing interests.
Peer review
Peer review information
Communications Biology thanks the anonymous reviewers for their contribution to the peer review of this work. Primary Handling Editor: Luke R. Grinham. Peer reviewer reports are available.
Additional information
Publisher’s note Springer Nature remains neutral with regard to jurisdictional claims in published maps and institutional affiliations.
Rights and permissions
Open Access This article is licensed under a Creative Commons Attribution 4.0 International License, which permits use, sharing, adaptation, distribution and reproduction in any medium or format, as long as you give appropriate credit to the original author(s) and the source, provide a link to the Creative Commons license, and indicate if changes were made. The images or other third party material in this article are included in the article’s Creative Commons license, unless indicated otherwise in a credit line to the material. If material is not included in the article’s Creative Commons license and your intended use is not permitted by statutory regulation or exceeds the permitted use, you will need to obtain permission directly from the copyright holder. To view a copy of this license, visit http://creativecommons.org/licenses/by/4.0/.
About this article
Cite this article
Field, E.N., Shepard, J.J., Clifton, M.E. et al. Semi-field and surveillance data define the natural diapause timeline for Culex pipiens across the United States. Commun Biol 5, 1300 (2022). https://doi.org/10.1038/s42003-022-04276-x
Received:
Accepted:
Published:
DOI: https://doi.org/10.1038/s42003-022-04276-x
Comments
By submitting a comment you agree to abide by our Terms and Community Guidelines. If you find something abusive or that does not comply with our terms or guidelines please flag it as inappropriate.