Abstract
Maternal obesity is associated with prolonged and dysfunctional labour, potentially through decreased synthesis of prostaglandins that stimulate myometrial contractions. We assessed the impact of maternal obesity on concentrations of precursor fatty acids (FA) for prostaglandin synthesis and whether any changes could be reversed by improved nutrition post-conception. Wistar rats were fed control (CON) or High-Fat, High-cholesterol (HFHC) diets 6 weeks before mating. At conception half the dams switched diets providing 4 dietary groups: (1) CON, (2) HFHC, (3) CON-HFHC or (4) HFHC-CON. During parturition rats were euthanized and FA composition of plasma, liver and uterus determined. Visceral fat was doubled in rats exposed to the HFHC diet prior to and/or during pregnancy compared to CON. HFHC diet increased MUFAs but decreased omega-3 and omega-6 PUFAs in plasma and liver. Uterine omega-3 FA concentrations were halved in HFHC versus CON rats, but all other FAs were similar. Switching from HFHC to CON diet at conception restored all FA profiles to those seen in CON rats. The increased MUFA and decreased PUFA concentrations in obese HFHC dams may contribute to aberrant prostaglandin synthesis and dysfunctional myometrial activity and it may be possible to reverse these changes, and potentially improve labour outcomes, by improving nutrition at conception.
Similar content being viewed by others
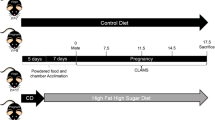
Introduction
Between the years 1975–2014 global obesity levels have increased from 105 to 641 million people1. The UK level of obesity in women of reproductive age currently stands at 20% and is estimated to reach 50% by 20502 and this is associated with a parallel increase in the number of women entering pregnancy with a high BMI (Centre for Maternal and Child Enquiries (3). Maternal obesity increases the risk of gestational diabetes, pre-eclampsia, post-partum haemorrhage and prolonged and dysfunctional labour resulting in emergency caesarean delivery3,4,5,6. Although the increased risks of adverse pregnancy outcomes in obese women is well established, very few studies have attempted to elucidate the underlying mechanism(s), and therefore identify potential strategies for intervention.
Prostaglandins PGF2α, PGE2 and PGI2 produced within intrauterine tissues play a central role in regulating uterine activity during pregnancy and parturition7, and increased production of these compounds is required for the initiation and progression of labour. PGE2 maintains uterine quiescence during pregnancy but stimulates myometrial contractions during labour via specific EP receptors8 whereas PGF2α stimulates myometrial contractions9 and PGI2 primes the myometrium for enhanced contractile response10. These 2 series prostaglandins are, in turn, derived from the omega-6 fatty acid arachidonic acid (AA 20:4 n-6). In contrast, the omega-3 fatty acid, eicosapentaenoic acid (EPA 20:5 n-3) gives rise to the 3 series prostaglandins, which are less biologically active and thus reduce uterine contractility and delay parturition. AA is either acquired from the diet or synthesized de novo from the precursor n-6 PUFA linoleic acid (LA, 18:2 n-6)11,12.
The fact that the omega-3 and omega-6 fats give rise to PGs with distinct biological activities has led to suggestions that the dietary balance of omega-6 and omega-3 fatty acids may play a key regulatory role in labour and parturition, particularly the physiological processes requiring prostaglandin synthesis. This is supported by human and animal studies that have shown a significant increase in the risk of premature labour when humans or animal consume diets rich in n-6 PUFA13,14. In contrast, diets high in n-3 PUFA significantly prolong gestation and labour15,16,17,18,19,20. Dietary alteration of prostaglandin synthesis has been proposed to occur through n-3 and n-6 PUFAs, such as DGLA (20:3, n-6) and EPA competing with AA for incorporation into phospholipids of the plasma membrane21,22 but also processing by desaturase23,24 and COX-2 enzymes that synthesise long chain PUFAs and prostaglandins respectively.
The role of altered fatty acid composition as a contributing factor to the increased risk of pregnancy complications in obese women has not been previously explored. This is despite clear evidence of the importance of dietary fatty acids in regulating the physiology and endocrinology of female reproduction and the timing and length of labour25, and evidence of altered prostaglandin production in the context of maternal obesity. We have recently established that plasma levels of PGF2α during labour are significantly lower in obese high-fat, high-cholesterol (HFHC) fed rats compared to lean controls26 suggesting that maternal obesity is associated with a decreased capacity for PGF2α synthesis. In addition, modulation of both monounsaturated fatty acids (MUFAs) and omega-3 and omega-6 polyunsaturated fatty acids (PUFAs) have been reported to affect the timing of parturition27,28,29 and may thus play a key role in the prolonged and dysfunctional labour often associated with maternal obesity4.
Consequently, the aim of the present study was to investigate the effects of dietary HFHC induced maternal obesity on plasma, hepatic and uterine fatty acid composition during labour, with a particular focus on the precursor fatty acids for prostaglandin synthesis. A second aim was to ascertain whether changing diet at conception can improve the n-6 fatty acid status and increase the capacity for PGF2α synthesis, and thus identify a potential intervention for reducing the risk of prolonged and dysfunctional labour associated with maternal obesity.
Results
Body and Fat Depot Weights
Feeding a HFHC diet for 6 weeks prior to mating was associated with a significant increase in body weight at conception (Fig. 1A). There was no difference in gestational weight gain between the 4 treatment groups (Fig. 1B). At the time of parturition, however, rats fed the HFHC diet before mating were still significantly heavier than rats fed the CON diet before mating, independent of their diet post-conception (P < 0.05). Body weight of the rats fed the CON diet before mating, but switched to the HFHC diet at conception (CON-HFHC) at the end of gestation was not different to those in any other treatment group (Fig. 1C). Visceral fat mass (sum of perirenal and gonadal fat) was 2-fold higher in rats exposed to the HFHC diet either before and/or post-conception compared to the CON group (Fig. 2).
Bodyweight changes 6 weeks before mating (A) during pregnancy (B) and final weight at term pregnancy (C) in rats fed either a CON, HFHC, CON-HFHC or HFHC-CON diet. Values are means with SEM represented by vertical bars. Dietary treatment groups with different superscript letters are significantly different at the P < 0.05 level.
Fatty acid composition of the plasma
The fatty acid profile of the plasma collected from rats fed a CON or HFHC diet for 6 weeks prior to and during pregnancy and those whose diet was switched at conception is presented in Table 1.
Saturated and monounsaturated fatty acids
Total plasma saturated fatty acid levels at partition were higher in the HFHC-CON group compared to all other dietary treatments (P < 0.009), but were not different between the CON, HFHC and CON-HFHC groups. Analysis of the individual saturated fatty acids identified that exposure to a HFHC either during the entire period, or only post-conception, was associated with significant increases in levels of myristic acid (14:0) and pentadecylic acid (15:0) in comparison to the CON group. These increases were partially (in the case of pentadecylic acid) or completely (in the case of myristic acid) normalised by switching rats fed a HFHC diet before mating to a CON diet after conception. Conversely, plasma levels of stearic (18:0) and behenic Acid (22:0) were lower in the CON-HFHC and HFHC rats when compared to CON, but were not different between the HFHC-CON and CON treatment groups.
Total plasma monounsaturated fatty acid concentrations, as well as concentrations the major omega-9 and -7 monounsaturated fatty acids, oleic acid (18:1 n-9) and palmitoleic acid (16:1 n-7), were increased by 3-fold in rats exposed to HFHC vs CON diet during the post-conception period, independent of their diet prior to mating (Table 1). Plasma concentrations of vaccenic acid (18:1 n-7), however, were not different between CON, a HFHC, and CON-HFHC dietary groups, but were lower in the CON-HFHC and HFHC-CON fed rats compared to those exposed to the HFHC diet during both the pre-conception and post-conception periods.
Omega-3 and omega-6 polyunsaturated fatty acids
Plasma total omega-3 PUFA levels at parturition were lower in rats fed the HFHC diet post-conception, independent of their diet before mating (Table 1). The same pattern was also observed for the individual omega-3 LCPUFA, EPA and DHA. Thus, feeding rats the HFHC diet during gestation was associated with reduced plasma EPA and DHA contents, however switching rats from a HFHC diet to a CON diet post-conception ameliorated these effects (Table 1). In the case of ALA, there appeared to be a dose-response relationship between HFHC exposure and plasma concentrations, such that plasma levels were lowest in rats fed the HFHC diet both before and after conception, highest in rats fed the CON diet during both periods and intermediate in the other two treatment groups (Table 1).
Total Omega-6 PUFA concentrations and concentrations of the omega-6 LCPUFA, AA, followed a similar pattern, and were lower in rats exposed to the HFHC diet compared to the CON diet post-conception (All P ≤ 0.007). Plasma LA (18:2 n-6), concentrations were lower in rats fed the HFHC diet either pre- or post-conception compared to the CON group, and were not restored to CON levels by switching HFHC rats to the CON diet after mating.
Fatty acid composition of the liver
Saturated and monounsaturated fatty acids
The fatty acid composition of the liver followed a similar pattern to that of the plasma (Table 2). Total saturated fat content was lower in rats exposed to the HFHC diet post-conception, independent of their diet prior to mating. Exposure to the HFHC diet post-conception increased liver myristic (14:0) and pentadecyclic (15:0) acid contents ≥ 2-fold when compared to CON, and switching from a HFHC to control diet post-conception completely reversed this effect in HFHC-CON animals. Liver palmitic acid (C16:0) content was lower in rats in the CON-HFHC vs CON treatment groups, but was not different between the CON, HFHC and HFHC-CON groups. Stearic (18:0), behenic (22:0) and lignoceric (24:0) acid were also lower in rats fed a HFHC diet post-conception, but were not different between the HFHC-CON and CON groups.
The most substantial shifts in hepatic fatty acid composition were observed in the monounsaturated fatty acid fraction, with total hepatic monounsaturated fatty acid content increased more than 4-fold in rats fed the HFHC diet post-conception, independent of their diet before mating (Table 2). Hepatic monounsaturated fatty acid content in rats switched from a HFHC diet to a control diet at conception was, however, not different to the CON group. Hepatic omega-9 and omega-7 monounsaturated fatty acid contents within the liver followed a similar pattern to total monounsaturated fatty acid contents. The individual omega-9 and omega-7 monounsaturated fatty acids significantly altered by diet in this way were oleic (18:1 n-9) and palmitoleic acids (16:1 n-7). Interestingly, the omega 7 fatty acid vaccenic acid (18:1, n-7) did not follow the same trend, and concentrations of this fatty acid were higher in HFHC rats in comparison to the CON, CON-HFHC, and HFHC-CON dietary groups.
Omega-3 and omega-6 polyunsaturated fatty acids
Hepatic total omega-3 content was 4-fold lower in rats fed the HFHC diet post-conception, compared to both the CON and HFHC-CON groups (Table 2). Rats switched from the control to HFHC diet at conception (CON-HFHC) had a hepatic omega-3 content that was intermediate between the HFHC-CON, CON and HFHC groups (Table 2). There was no effect of the HFHC diet on hepatic ALA concentrations, but concentrations of EPA, DPA and DHA were all lower in rats exposed to the HFHC diet after conception compared to the CON treatment group. In particular, DHA levels were reduced approximately 5-fold in HFHC fed rats compared to the CON group. Rats that were switched from a CON to HFHC diet at conception had lower EPA, DHA and DPA contents than CON rats, but DPA and DHA levels were still significantly higher than in rats fed the HFHC diets both before and after conception (HFHC). Conversely, EPA, DPA and DHA concentrations in rats switched from a HFHC diet to a CON diet at conception were similar to those in CON animals.
Total hepatic omega 6 polyunsaturated fatty acid levels of the liver were approximately 5% lower in HFHC rats compared to the CON group, but was not different between the CON, CON-HFHC, and HFHC-CON groups. In terms of individual omega-6 fatty acids, gamma linoleic (18:3 n-6) and di-homo-gamma-linoleic (20:3 n-6) acid were not significantly different among the 4 dietary groups, while other major omega-6 PUFA were significantly altered. Thus, hepatic LA content was increased in rats exposed to a HFHC diet post-conception when compared to CON rats, but was not different between the HFHC-CON and CON groups. In contrast, exposure to the HFHC diet during pregnancy significantly decreased hepatic levels of both eicosadienoic (20:2, n-6) and AA when compared to the CON group, but were not different between the HFHC-CON and CON groups. Interestingly there also appeared to be a dose-response relationship between the duration of HFHC exposure and hepatic AA concentrations, such that levels were lowest in rats fed the HFHC diet both before and after conception, highest in rats fed the CON diet during both periods and intermediate in the CON-HFHC and HFHC-CON groups.
Fatty acid composition of the uterus
Uterine composition of fatty acids are presented in Table 3. The uterus was particularly resistant to any change in fatty acid composition, and there were no differences in the content of saturated, monounsaturated or omega-6 polyunsaturated fatty acids between the 4 treatment groups. Total omega-3 fatty acid content in the uterine horn was, however, ~2-fold lower in the HFHC group compared to the CON group (P < 0.022). Importantly, rats switched from a HFHC diet to a control diet at conception had omega-3 fatty acid concentrations in their uterine horn that were not different from the CON group. The shifts in uterine omega-3 concentrations appeared to be driven primarily by changes in DHA, which tended (P = 0.095) to follow a similar pattern to that for total omega-3 concentration (Table 3).
Discussion
The overall aim of the current study was to investigate the effects of HFHC feeding to induce obesity and its subsequent effects on the fatty acid composition of the plasma, liver and uterus. As expected, feeding the HFHC diet significantly increased the weight of the dams both pre-gestation and during pregnancy. The increases in bodyweight was attributed to significant increases in the accumulation of visceral fat, which was twice as high in rats exposed to the HFHC diet either before or after conception compared to rats fed a control diet during this entire period.
With confirmation that the HFHC diet causes maternal obesity, we wanted to determine its impact on plasma, hepatic and uterine fatty acid composition and whether it can be reversed by a dietary change at conception. What is evident from the current study is that exposure to the HFHC diet significantly alters the fatty acid profile of the plasma, liver and, to a more limited extent, the uterus. The fatty acid profile of both the plasma and liver from HFHC fed rats was characterised by significant increases in MUFAs with parallel decreases in total omega-3 and omega-6 PUFAs when compared to CON fed rats, findings consistent with previous studies utilising maternal high fat diets in rats and non-human primates30,31 as well as the fatty acid status of obese adults32,33 and obese pregnant women at mid-gestation34. Critically, we have established that switching diets at conception from a CON or HFHC diet and feeding a HFHC or CON diet during pregnancy respectively, reversed the plasma fatty acid profiles, such that these mirrored those of the post-conception diets. This suggests that alterations in circulating and hepatic fatty acid composition in rats consuming an obesogenic HFHC diet before conception, can be favourably altered by switching to a CON diet during pregnancy.
One intriguing finding was that exposure to a HFHC diet significantly altered the fatty acid profiles of the plasma and liver, but induced very little change in the uterus. The uterus only differed in total omega-3 fatty acids, which was reduced by half by the HFHC diet, and this effect was reversed when rats were switched to a CON diet post-conception. It is plausible that the uterus could be resistant to changes in dietary fatty acids, similar to the brain35. A further possibility could be that by collecting tissue from labouring animals the fatty acids within the uterus necessary for labour had been utilised, and differences may only be apparent prior to the onset of labour. Nevertheless, a decrease in omega-3 fatty acids in the uterus following feeding of the HFHC diet has the potential to compromise uterine CAP expression by altering the fluidity of the plasma membrane4. Fatty acids play a vital role in the formation of the plasma membrane lipid bilayer, and increases in membrane PUFA content is associated with increased membrane fluidity, which in turn affects the expression, function, and downstream signalling of integral proteins35. This theory is supported by the findings of de Jonge et al.36 where the membrane fluidity of cultured rat ventricular myocytes decreases following incorporation of the omega-3 fatty acid EPA and that this is associated with increases in the regularity of their spontaneous contractions. It was also evident in the same study that ventricular myocytes treated with endothelin 1 to stimulate a phospholipase-C β response (PLC- β) was significantly increased following EPA treatment when compared to myocytes grown in control medium. These research findings are significant in respect to the current study because endothelin-1 causes potent constriction of myometrial strips from term human pregnancies37 and the PLC signalling pathway plays an important role in regulating uterine contractile activity. As a result, a plausible deduction would be that the halving of the total omega-3 fatty acids within the uterus following exposure to the HFHC diet could be playing a key role in the un-coordinated ex vivo contractions previously observed in our maternal obesity model38.
An additional mechanism through which fatty acids can impact on the physiology of female reproduction is through alterations in prostaglandin synthesis, particularly synthesis of the 2 series prostaglandins PGF2α and PGE2 which can both stimulate8,39 and relax8 myometrial contractions respectively. The omega-6 PUFA AA is the direct precursor for synthesis of the 2 series prostaglandins and there is vast evidence to show that dietary manipulation of omega-6 PUFAs can impact on PGF2α and PGE2 production during pregnancy. Pregnant sheep fed a diet high in the essential omega-6 PUFA linoleic acid (LA) significantly increased the level of AA within plasma and placental tissues which was associated with a significant increases in both PGF2α and PGE2 synthesis29 and an increased risk of premature labour13. In contrast, dietary supplementation with omega-3 PUFAs the fatty acid precursors for synthesis of the less potent 3-series prostaglandins PGF3α and PGE3 reduces premature birth rates40. Furthermore, continual intravenous infusion of fish oil concentrate to pregnant ewes has been shown to delay and occasionally prevent glucocorticoid-induced preterm delivery15. Not surprisingly there has long been an interest in the use of n-3 PUFA as an effective tocolytic agent in human pregnancy. Using our model of maternal obesity we have provided evidence that a HFHC diet causes a significant reduction in plasma levels of PGF2α during labour26 and poorly coordinated myometrial contractions ex vivo38. This significant decrease in plasma PGF2α is a result of HFHC animals consuming a diet 33% lower in the availability of LA, which translates to the lower circulating levels of the prostaglandin precursor AA identified in this report (CON 18.39% ± 1.20 vs HFHC 11.57% ± 0.81; P < 0.0001). Essentially, the current study provides evidence that changing diet at conception from a HFHC to CON diet can reverse these effects and increase the plasma level of AA (HFHC-CON 19.63% ± 0.70) thereby increasing the capacity for prostaglandin synthesis and potentially improvement of labour outcomes with maternal obesity.
One of most dramatic changes observed in the current study was that following exposure to a HFHC diet there was a significant 3- & 5-fold increase in the plasma and hepatic levels of the MUFA oleic acid (18:1 n-9) respectively. Very little research has investigated the specific effects of oleic acid on prostaglandin production. However, one recently published study suggests oleic acid could also contribute to the decrease in plasma PGF2α observed in our maternal obesity model26. Primary culture of endometrial cells from late pregnant ewes showed that oleic acid supplementation favoured PGE2 production and attenuated PGF2α synthesis even in the presence of the prostaglandin synthesis stimulator oxytocin27. Interestingly, the authors speculated that raised circulating levels of oleic acid may affect the initiation and progress of parturition and could also offer a further potential mechanism behind the dysfunctional labour associated with maternal obesity. If future research can confirm inhibitory effects of oleic acid, on PGF2α production then the current study shows clearly that high circulatory and hepatic levels of oleic acid caused by consumption of a HFHC diet can be decreased very easily by changing the composition of the diet consumed during pregnancy. This in turn could increase the capacity for PGF2α synthesis.
It is important to consider that changes in fatty acid composition may compromise uterine contractile activity through mechanisms other than prostaglandin synthesis. One potential mechanism is the direct effect of fatty acids on uterine expression of key contraction-associated proteins (CAPs). One such protein is connexin-43 (Cx-43), which is essential for formation of gap junctions that act as intracellular conduits to facilitate cell-cell electro-coupling (synchronisation of muscular contractions) and metabolite transfer41. Loss of expression of Cx-43 is associated with dysfunctional myometrial contractile activity and prolonged labour41,42,43,44. Our previous research identified that exposure to a HFHC significantly decreases uterine expression of Cx-43 during labour26,38. Quantification of the phosphorylated form of Cx-43 (pCX-43, phosphorylation at serine 368) was significantly higher in labouring HFHC fed rats compared to controls38. This is an important observation as phosphorylation of CX-43 compromises gap junction assembly, trafficking, and intracellular communication45. Interestingly, cardiomyocyte contractile activity is significantly decreased when exposed to oleic acid in vitro by inducing gap junction disassembly as a result of phosphorylation of CX-43 by protein kinase C (PKC)46. Thus, the increased circulating and hepatic oleic acid levels observed in rats fed the HFHC diet in the present study could potentially contribute to the decrease in Cx-43 and increase in pCx-43 previously observed in the labouring uteri of our HFHC fed rats38.
To summarize, exposure to a HFHC diet to induce obesity resulted in significant shifts in plasma and hepatic fatty acid composition, but only altered levels of omega-3 fats in the uterus. HFHC feeding was associated with significant increases in MUFAs and parallel decreases in total omega-3 and omega-6 PUFAs in both the plasma and liver. In contrast the uterus was more resistant to changes in dietary fatty acid incorporation, but the uteri from HFHC exposed rats did exhibit a significant decrease in the total omega-3 fatty acids. What is very clear from the fatty acid profile of HFHC fed animals is that the diet has the capacity to detrimentally alter prostaglandin synthesis, membrane fluidity, and uterine contractile protein expression. All of the aforementioned mechanisms may play a role in prolonged and dysfunctional labour associated with maternal obesity. Of key importance is the evidence that changing from a HFHC to CON diet at conception with the absence of any reduction in maternal fat mass, reverses the fatty acid profile associated with obesity and has the potential to improve uterine contractile activity and labour outcome.
Although change of diet at conception to improve labour outcomes in obese pregnancies may be an effective approach, further research is required to determine the effects of the HFHC diet and change of diet at conception on (1) prostaglandin synthesis, (2) uterine expression of the key contractile associated proteins and (3) myometrial excitation-contraction coupling and contractility both ex vivo and in vivo.
Materials and Methods
Animals and Experimental Design
All animal experiments were performed in accordance with the relevant laws and institutional guidelines and were approved by the University of Nottingham Animal Welfare Ethical Review Board (AWERB) and the UK Home Office [Project licence code (PPL) 40/3598]. All procedures were carried out by licenced researchers under the Animals in Scientific Procedures Act (ASPA) of 1986 within the animal facilities of the University of Nottingham.
A total of 22 weanling virgin female Wistar rats (Rattus rattus) weighing approximately 60 g (Charles River, UK) were pair housed under normal conditions (12 hour light: dark photoperiod, 21 °C ± 5 °C room temperature, 55% ± 5% relative humidity, food and water access ad libitum) and randomly assigned to be fed either a standard control laboratory chow (CON, n = 11) (Harlan Laboratories, UK) or High-Fat, High-Cholesterol diet (HFHC, n = 11) as detailed in Table 4. Nutritional breakdown and fatty acid composition of the CON and HFHC diets are also provided in Tables 5 and 6. Rats were pair housed and maintained on their respective diets for 6 weeks prior to mating with stud Wistar males (Charles River, UK). Pregnancy was confirmed upon discovery of a semen plug and was recorded as gestational day 0. Once conception was confirmed half of the rat dams from each dietary group were switched to the alternate diet throughout pregnancy, such that CON fed rats were switched to a HFHC diet and HFHC fed rats to a CON chow diet respectively. This provided 4 experimental treatment groups: (1) CON (n = 5), (2) HFHC (n = 6), (3) CON-HFHC (n = 6) or (4) HFHC-CON (n = 5) diet.
All pregnant rats were then individually housed and maintained on their respective diet throughout gestation. Daily food intake and weight gain measurements were recorded prior to and during pregnancy. At gestational day 22, hourly checks were made for signs of parturition and following birth of the 5th pup each rat dam was immediately euthanized by CO2 asphyxiation and cervical dislocation. Pups were euthanased by overdose of pentobarbitone and cervical dislocation. Maternal blood was immediately collected via cardiac puncture into EDTA coated tubes (Sarstedt, Nümbrecht, Germany), and subsequently centrifuged at 13,000 rpm and the plasma snap frozen in liquid nitrogen and stored at −80 °C. The uterus, liver, gonadal and perineal fat depots were dissected out, weighed, snap frozen in liquid nitrogen and stored at −80 °C for future analysis.
Lipid Extraction
Prior to lipid extraction, liver and uterine tissue was crushed into a fine powder under liquid nitrogen, using a mortar and pestle. A total of 300 mg of crushed rat liver or uterine horn was homogenised in 2 mls of ice-cold 0.9% saline in 30-second bursts. The homogenate was then vortexed in 3 ml 99.9% Propan-2-ol, and left to incubate at room temperature for 5 minutes. After the 5 min incubation period, 6 ml of 98% chloroform was added to the solution, inverted, and centrifuged at 3000 rpm for 10 minutes. Following centrifugation, the chloroform layer was transferred into a fresh tube using a disposable glass pipette and dried under nitrogen gas at 37 °C. Once evaporated, samples were then reconstituted in either 500 μl (liver) or 100 μl (uterine horn) 9:1 Chloroform: Methanol, and 20 μl of this solution was then pipetted onto PUFA COATTM collection cards47 for fatty acid analysis.
Lipid Analysis
All samples were analysed for their fatty acid profile using a gas chromatography flame ionisation detector (GC-FID) as described previously by Liu et al.47.
Statistical Analysis
All the data were analysed using the Statistical Package for Social Science (Version 21; SPSS Inc, Chicago, IL, USA). The homogeneity of the data was assessed and if not normally distributed appropriately transformed to achieve normal distribution. To determine the effect of maternal obesity and change of diet at conception on the plasma, hepatic and uterine fatty acid composition during labour the statistical test used was one-way ANOVA, with LSD post-hoc tests to determine difference between individual treatment groups where the ANOVA revealed significant differences between groups. All data are expressed as the mean value ± SEM and P = ≤0.05 was considered statistically significant.
Change history
27 September 2018
A correction to this article has been published and is linked from the HTML and PDF versions of this paper. The error has not been fixed in the paper.
References
NCD Risk Factor Collaboration. Trends in adult body-mass index in 200 countries from 1975 to 2014: a pooled analysis of 1698 population-based measurement studies with 19·2 million participants. Lancet 387, 1377–1396 (2016).
Butland, B. et al. Tackling Obesities: Future Choices – Project report. Foresight Report (2007).
Centre for Maternal and Child Enquiries (CMACE). Maternal obesity in the UK: Findings from a national project. Pediatric diabetes. London, UK (2010).
Bogaerts, A., Witters, I., Van den Bergh, B. R. H., Jans, G. & Devlieger, R. Obesity in pregnancy: altered onset and progression of labour. Midwifery 29, 1303–13 (2013).
Kominiarek, M. A. et al. Contemporary labor patterns: the impact of maternal body mass index. Am. J. Obstet. Gynecol 205, 244.e1–244.e8 (2011).
Poobalan, A. S., Aucott, L. S., Gurung, T., Smith, W. C. S. & Bhattacharya, S. Obesity as an independent risk factor for elective and emergency caesarean delivery in nulliparous women–systematic review and meta-analysis of cohort studies. Obes Rev 10, 28–35 (2009).
Zuo, J., Lei, Z. M., Rao, C. V., Pietrantoni, M. & Cook, V. D. Differential cyclooxygenase-1 and -2 gene expression in human myometria from preterm and term deliveries. J Clin Endocrinol Metab 79(3), 894–9 (1994).
Kandola, M. K. et al. EP2 Receptor Activates Dual G Protein Signalling Pathways that Mediate Contrasting Proinflammatory and Relaxatory Responses in Term Pregnant Human Myometrium. Endocrinology 155(2), 605–617 (2014).
Challis, J. & Lye, S. Parturition. In The Physiology of Reproduction, vol 2, pp 985–1031 (1994).
Fetalvero, K. M. et al. Prostacyclin primes pregnant human myometrium for an enhanced contractile response in parturition. J Clin Invest 118(12), 3966–79 (2008).
Lands, W. E. Biochemistry and physiology of n-3 fatty acids. FASEB J 6(8), 2530–6 (1992).
Fischer, S. Dietary polyunsaturated fatty acids and eicosanoid formation in humans. Adv Lipid Res 23, 169–98 (1989).
Elmes, M. et al. Raised dietary n-6 polyunsaturated fatty acid intake increases 2-series prostaglandin production during labour in the ewe. J. Physiol 562, 583–592 (2005).
Reece, M. S., McGregor, J. A., Allen, K. G. & Harris, M. A. Maternal and perinatal long-chain fatty acids: possible roles in preterm birth. Am. J. Obstet. Gynecol 176, 907–14 (1997).
Baguma-Nibasheka, M., Brenna, J. T. & Nathanielsz, P. W. Delay of preterm delivery in sheep by omega-3 long-chain polyunsaturates. Biol. Reprod 60, 698–701 (1999).
Leat, W. M. F. & Northrop, C. A. Effect of linolenic acid on gestation and parturition in the rat. Prog. Lipid Res 20, 819–821 (1981).
Ma, X. H., Wu, W. X., Brenna, J. T. & Nathanielsz, P. W. Maternal intravenous administration of long chain n-3 polyunsaturates to the pregnant ewe in late gestation results in specific inhibition of prostaglandin H synthase (PGHS) 2, but not PGHS1 and oxytocin receptor mRNA in myometrium during betamethasone-in. J. Soc. Gynecol. Investig 7, 233–237 (2000).
Olsen, S. F., Hansen, H. S. & Jensen, B. Fish oil versus arachis oil food supplementation in relation to pregnancy duration in rats. Prostaglandins, Leukot. Essent. Fat. Acids 40, 255–260 (1990).
Olsen, S. F. et al. Intake of marine fat, rich in (n-3)-polyunsaturated fatty acids, may increase birthweight by prolonging gestation. Lancet 2, 367–369 (1986).
Waltman, R., Tricomi, V., Shabanah, E. H. & Arenas, R. Prolongation of gestation time in rats by unsaturatted fatty acids. Am. J. Obstet. Gynecol 127, 626–627 (1977).
Trujillo, E. P. & Broughton, K. S. Ingestion of n-3 polyunsaturated fatty acids and ovulation in rats. J Reprod Fertil 105(2), 197–203 (1995).
Howie, A., Leaver, H. A., Wilson, N. H., Yap, P. L. & Aitken, I. D. The influence of dietary essential fatty acids on uterine C20 and C22 fatty acid composition. Prostaglandins Leukot Essent Fatty Acids 46(2), 111–21 (1992).
Sprecher, H. Biochemistry of essential fatty acids. Prog Lipid Res 20, 13–22 (1981).
Emken, E. A., Adlof, R. O., Rakoff, H., Rohwedder, W. K. & Gulley, R. M. Metabolism in vivo of deuterium-labelled linolenic and linoleic acids in humans. Biochem Soc Trans 18(5), 766–9 (1990).
Wathes, D. C., Abayasekara, D. R. E. & Aitken, R. J. Polyunsaturated fatty acids in male and female reproduction. Biol Reprod 77, 190–201 (2007).
Elmes, M. J., Tan, D. S., Cheng, Z., Wathes, D. C. & Mcmullen, S. The effects of a high-fat, high-cholesterol diet on markers of uterine contractility during parturition in the rat. Reproduction 141, 283–290 (2011).
Cheng, Z., Abayasekara, D. R., Elmes, M., Kirkup, S. & Wathes, D. C. Effect of oleic acid supplementation on prostaglandin production in maternal endometrial and fetal allantochorion cells isolated from late gestation ewes. Placenta 36(9), 1011–7 (2015).
Kar, S., Wong, M., Rogozinska, E. & Thangaratinam, S. Effects of omega-3 fatty acids in prevention of early preterm delivery: a systematic review and meta-analysis of randomized studies. Eur J Obstet Gynecol Reprod Biol 198, 40–6 (2016).
Elmes, M. et al. The effect of dietary supplementation with linoleic acid to late gestation ewes on the fatty acid composition of maternal and fetal plasma and tissues and the synthetic capacity of the placenta for 2-series prostaglandins. Biochim Biophys Acta 1686(1-2), 139–47 (2004).
Cerf, M. E. & Herrera, E. High fat diet administration during specific periods of pregnancy alters maternal fatty acid profiles in the near-term rat. Nutrients 8 (2016).
Grant, W. F. et al. Maternal high fat diet is associated with decreased plasma n-3 fatty acids and fetal hepatic apoptosis in nonhuman primates. PLoS One 6 (2011).
Karlsson, M. et al. Serum phospholipid fatty acids, adipose tissue, and metabolic markers in obese adolescents. Obesity (Silver Spring) 14(11), 1931–9 (2006).
Micallef, M., Munro, I., Phang, M. & Garg, M. Plasma n-3 Polyunsaturated Fatty Acids are negatively associated with obesity. Br J Nutr. 102(9), 1370–4 (2009).
Vidakovic, A. J. et al. Body mass index, gestational weight gain and fatty acid concentrations during pregnancy: the Generation R Study. Eur J Epidemiol 30(11), 1175–85 (2015).
Murphy, M. G. Dietary fatty acids and membrane protein function. J. Nutr. Biochem 1, 68–79 (1990).
de Jonge, H. W. et al. Eicosapentaenoic acid incorporation in membrane phospholipids modulates receptor-mediated phospholipase C and membrane fluidity in rat ventricular myocytes in culture. J Mol Cell Cardiol 28(5), 1097–108 (1996).
Wolff, K. et al. Contractile effects of endothelin 1 and endothelin 3 on myometrium and small intramyometrial arteries of pregnant women at term. Gynecol Obstet Invest 36(3), 166–71 (1993).
Muir., R. et al. Modelling maternal obesity: the effects of a chronic high-fat, high-cholesterol diet on uterine expression of contractile-associated proteins and ex vivo contractile activity during labour in the rat. Clin Sci (Lond) 130(3), 183–92 (2016).
Challis, J. R., Lye, S. J. & Gibb, W. Prostaglandins and parturition. Ann. N. Y. Acad. Sci 828, 254–67 (1997).
Salvig, J. D. & Lamont, R. F. Evidence regarding an effect of marine n-3 fatty acids on preterm birth: a systematic review and meta-analysis. Acta Obstet Gynecol Scand 90(8), 825–38 (2011).
Sims, S. M., Daniel, E. E. & Garfield, R. E. Improved Electrical Coupling in Uterine Smooth Muscle Is Associated with Increased Numbers of Gap junctions at Parturition. J. Gen. Physiol 80, 353–375 (1982).
Döring, B. et al. Ablation of connexin43 in uterine smooth muscle cells of the mouse causes delayed parturition. J. Cell Sci 119, 1715–22 (2006).
Cluff, A. H. et al. Prolonged labour associated with lower expression of syndecan 3 and connexin 43 in human uterine tissue. Reprod Biol Endocrinol 4(4), 24 (2006).
Tong, D. et al. A dominant loss-of-function GJA1 (Cx43) mutant impairs parturition in the mouse. Biol. Reprod 80, 1099–106 (2009).
Su, V. & Lau, A. F. Connexins: Mechanisms regulating protein levels and intercellular communication. FEBS Lett. 588, 1212–1220 (2014).
Huang, Y. S., Tseng, Y. Z., Wu, J. C. & Wang, S. M. Mechanism of oleic acid-induced gap junctional disassembly in rat cardiomyocytes. J. Mol. Cell. Cardiol. 37, 755–766 (2004).
Liu, G., Mühlhäusler, B. S. & Gibson, R. A. A method for long term stabilisation of long chain polyunsaturated fatty acids in dried blood spots and its clinical application. Prostaglandins Leukot Essent Fatty Acids 91(6), 251–60 (2014).
Acknowledgements
We would like to acknowledge the expert technical assistance of Mr R Plant and Mr Mick Baker. We have no conflict of interest. This work was funded by the University of Nottingham BBSRC-DTP PhD studentship, University Hospitals Coventry & Warwickshire NHS Trust studentship [grant number BB/J014508/1] and Rosetrees Trust [grant number M449] and the United Arab Emirates University [Research Grant number 31M311].
Author information
Authors and Affiliations
Contributions
Study design and management was performed by Matthew Elmes. Daily running of the animal study was done by Ronan Muir. Animal trial assistance and collection of tissue was carried out by Ronan Muir and Matthew Elmes. Laboratory work was carried out by Ronan Muir and Ge Liu. The manuscript was written and reviewed by Matthew Elmes, Raheela Khan, Anatoly Shmygol, Siobhan Quenby, Robert Alan Gibson, and Beverly Muhlhausler.
Corresponding author
Ethics declarations
Competing Interests
The authors declare no competing interests.
Additional information
Publisher's note: Springer Nature remains neutral with regard to jurisdictional claims in published maps and institutional affiliations.
Rights and permissions
Open Access This article is licensed under a Creative Commons Attribution 4.0 International License, which permits use, sharing, adaptation, distribution and reproduction in any medium or format, as long as you give appropriate credit to the original author(s) and the source, provide a link to the Creative Commons license, and indicate if changes were made. The images or other third party material in this article are included in the article’s Creative Commons license, unless indicated otherwise in a credit line to the material. If material is not included in the article’s Creative Commons license and your intended use is not permitted by statutory regulation or exceeds the permitted use, you will need to obtain permission directly from the copyright holder. To view a copy of this license, visit http://creativecommons.org/licenses/by/4.0/.
About this article
Cite this article
Muir, R., Liu, G., Khan, R. et al. Maternal obesity-induced decreases in plasma, hepatic and uterine polyunsaturated fatty acids during labour is reversed through improved nutrition at conception. Sci Rep 8, 3389 (2018). https://doi.org/10.1038/s41598-018-21809-9
Received:
Accepted:
Published:
DOI: https://doi.org/10.1038/s41598-018-21809-9
Keywords
Comments
By submitting a comment you agree to abide by our Terms and Community Guidelines. If you find something abusive or that does not comply with our terms or guidelines please flag it as inappropriate.