Abstract
Ocean acidification is a global phenomenon, but it is overlaid by pronounced regional variability modulated by local physics, chemistry and biology. Recognition of its multifaceted nature and the interplay of acidification with other ocean drivers has led to international and regional initiatives to establish observation networks and develop unifying principles for biological responses. There is growing awareness of the threat presented by ocean acidification to ecosystem services and the socio-economic consequences are becoming increasingly apparent and quantifiable. In this higher-CO2 world, future challenges involve better design and rigorous testing of adaptation, mitigation and intervention options to offset the effects of ocean acidification at scales ranging from local to regional.
This is a preview of subscription content, access via your institution
Access options
Access Nature and 54 other Nature Portfolio journals
Get Nature+, our best-value online-access subscription
$29.99 / 30 days
cancel any time
Subscribe to this journal
Receive 12 print issues and online access
$209.00 per year
only $17.42 per issue
Buy this article
- Purchase on Springer Link
- Instant access to full article PDF
Prices may be subject to local taxes which are calculated during checkout
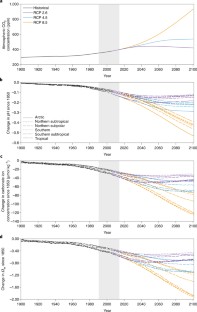




Similar content being viewed by others
References
IPCC Climate Change 2014: Impacts, Adaptation, and Vulnerability (eds Field, C. B. et al.) Part A (Cambridge Univ. Press, 2014).
Ocean Acidification Due to Increasing Atmospheric Carbon Dioxide Policy Document 12/05 (Royal Society, 2005).
Hönisch, B. et al. The geological record of ocean acidification. Science 335, 1058–1063 (2012).
Orr, J. C., Pantoja, S. & Pörtner, H.-O. Introduction to special section: the ocean in a high-CO2 world. J. Geophys. Res. 110, C09S01 (2005).
Cooley, S. #OHCO2What? New directions at the Ocean in a High-CO2 World Meeting. OCB Newslett. 5(3), 13–17 (2012).
Feeley, R. A. et al. Decadal changes in the aragonite and calcite saturation state of the Pacific Ocean. Glob. Biogeochem. Cycles 26, GB3001 (2012).
Bates, N. R. et al. A time-series view of changing surface ocean chemistry due to ocean uptake of anthropogenic CO2 and ocean acidification. Oceanography 27, 126–141 (2014). This paper provides a comprehensive assessment of changes in open ocean carbon chemistry related to the uptake of CO 2 from the atmosphere using decadal-scale time-series records.
Lauvset, S. K. et al. Trends and drivers in global surface ocean pH over the past 3 decades. Biogeosciences 12, 1285–1298 (2015).
IPCC Climate Change 2014: Synthesis Report (eds Core Writing Team, Pachauri, R. K. & Meyer, L. A.) (IPCC, 2014).
Lovenduski, N. S., Long, M. C. & Lindsay, K. Natural variability in the surface ocean carbonate ion concentration. Biogeosciences 12, 6321–6335 (2015).
Friedrich, T. et al. Detecting regional anthropogenic trends in ocean acidification against natural variability. Nat. Clim. Change 2, 167–171 (2012).
Mattsdotter, B., Fransson, A., Torstensson, A. & Chierici, M. Ocean acidification state in western Antarctic surface waters: controls and interannual variability. Biogeosciences 11, 57–73 (2014).
Qi, D. et al. Increase in acidifying water in the western Arctic Ocean. Nat. Clim. Change 7, 195–199 (2017).
Hauri, C., Friedrich, T. & Timmermann, A. Abrupt onset and prolongation of aragonite undersaturation events in the Southern Ocean. Nat. Clim. Change 6, 172–176 (2015).
Yamamoto, A. et al. Impact of rapid sea-ice reduction in the Arctic Ocean on the rate of ocean acidification. Biogeosciences 9, 2365–2375 (2012).
Waldbusser, G. G. & Salisbury, J. E. Ocean acidification in the coastal zone from an organism’s perspective: multiple system parameters, frequency domains, and habitats. Annu. Rev. Mar. Sci. 6, 221–247 (2014).
Mongin, M. et al. The exposure of the Great Barrier Reef to ocean acidification. Nat. Commun. 7, 10732 (2016).
Chan, F. et al. Persistent spatial structuring of coastal ocean acidification in the California Current System. Sci. Rep. 7, 2526–2533 (2017). This paper highlights the complexities of ocean acidification in coastal systems and the need to develop observational networks.
Newton, J. A. et al. Global Ocean Acidification Observing Network: Requirements and Governance Plan 2nd edn (GOA-ON, 2015); http://www.goa-on.org/documents/general/GOA-ON_2nd_edition_final.pdf
Matear, R. & Lenton, A. Sensitivity of future ocean acidification to carbon climate feedbacks. Biogeosciences 15, 1721–1732 (2017).
Zhang, H. & Cao, L. Simulated effect of calcification feedback on atmospheric CO2 and ocean acidification. Sci. Rep. 6, 20284 (2016).
Cornwall, C. E. et al. Diffusion boundary layers ameliorate the negative effects of ocean acidification on the temperate coralline macroalga Arthrocardia corymbosa. PloS ONE 9, e97235 (2014).
Cornwall, C. E. et al. Diurnal fluctuations in seawater pH influence the response of a calcifying macroalga to ocean acidification. Proc. R. Soc. B 280, 20132201 (2013).
Wahl, M. et al. Macroalgae may mitigate ocean acidification effects on mussel calcification by increasing pH and its fluctuations. Limnol. Oceanogr. 63, 3–21 (2017). This paper illustrates how coastal macrophyte beds may locally mitigate the negative effects of OA on calcifying invertebrates.
Eriander, L., Wrange, A.-L. & Havenhand, J. N. Simulated diurnal pH fluctuations radically increase variance in—but not the mean of—growth in the barnacle Balanus improvisus. ICES J. Mar. Sci. 73, 596–603 (2016).
Kroeker, K. J. et al. Impacts of ocean acidification on marine biota: quantifying variation in sensitivity among organisms and life stages and at elevated temperature. Glob. Change Biol. 19, 1884–1896 (2013).
Hutchins, D. A., Fu, F. X., Webb, E. A., Walworth, N. & Tagliabue, A. Taxon-specific response of marine nitrogen fixers to elevated carbon dioxide concentrations. Nat. Geosci. 6, 790–795 (2013). This paper reveals distinct differences in the CO 2 affinities of Trichodesmium strains across oceanic provinces.
Ventura, A., Schulz, S. & Dupont, S. Maintained larval growth in mussel larvae exposed to acidified undersaturated seawater. Sci. Rep. 6, 23728 (2016).
Bach, L. T. et al. Dissecting the impact of CO2 and pH on the mechanisms of photosynthesis and calcification in the coccolithophore Emiliania huxleyi. New Phytol. 199, 121–134 (2013).
Comeau, S. et al. Coral calcifying fluid pH is modulated by seawater carbonate chemistry not solely seawater pH. Proc. R. Soc. B 284, 20161669 (2017).
Comeau, S., Cornwall, C. E. & McCulloch, M. T. Decoupling between the response of coral calcifying fluid pH and calcification to ocean acidification. Sci. Rep. 7, 7573 (2017).
Diaz-Pulido, G. et al. Greenhouse conditions induce mineralogical changes and dolomite accumulation in coralline algae on tropical reefs. Nat. Commun. 5, 3310 (2014).
Kamenos, N. A., Perna, G., Gambi, M. C., Micheli, F. & Kroeker, K. J. Coralline algae in a naturally acidified ecosystem persist by maintaining control of skeletal mineralogy and size. Proc. R. Soc. B 283, 20161159 (2016).
Gazeau, F., Parker, L. M., Comeau, S., Gattuso, J. P. & O’Connor, W. A. Impacts of ocean acidification on marine shelled molluscs. Mar. Biol. 160, 2207–2245 (2013).
Hildebrandt, N., Sartoris, F. J., Schulz, K. G., Riebesell, U. & Niehoff, B. Ocean acidification does not alter grazing in the calanoid copepods Calanus finmarchicus and Calanus glacialis. ICES J. Mar. Sci. 73, 927–936 (2016).
Gattuso, J. P. et al. Free-ocean CO2 enrichment (FOCE) systems: present status and future developments. Biogeosciences 11, 4057–4075 (2014).
Wahl, M. et al. A mesocosm concept for the simulation of near-natural shallow underwater climates: the Kiel Outdoor Benthocosms (KOB). Limnol. Oceanogr. Methods 13, 651–663 (2015).
Nagelkerken, I. et al. Species interactions drive fish biodiversity loss in a high-CO2 world. Curr. Biol. 27, 2177–2184 (2017).
Fabricius, K. E., Kluibenschedl, A., Harrington, L., Noonan, S. & De’ath, G. In situ changes of tropical crustose coralline algae along carbon dioxide gradients. Sci. Rep. 5, 9537 (2015).
Shamberger, K. et al. Diverse coral communities in naturally acidified waters of a Western Pacific reef. Geophys. Res. Lett. 41, 499–504 (2014).
Albright, R. et al. Carbon dioxide addition to coral reef waters suppresses net community calcification. Nature 555, 516–519 (2018). This paper offers compelling evidence of ecosystem-wide responses by coral reefs to OA that can impact reef growth and reduce survivorship.
Waldbusser, G. G. et al. Saturation-state sensitivity of marine bivalve larvae to ocean acidification. Nat. Clim. Change 5, 273–280 (2015).
Bunse, C. et al. Response of marine bacterioplankton pH homeostasis gene expression to elevated CO2. Nat. Clim. Change 6, 483–487 (2016).
Raven, J. A., Beardall, J. & Giordano, M. Energy cost of carbon dioxide concentrating mechanisms of aquatic organisms. Photosynth. Res. 121, 111–24 (2014).
Cornwall, C. E. et al. Inorganic carbon physiology underpins macroalgal responses to elevated CO2. Sci. Rep. 7, 46297 (2017).
Peng, J. et al. Ocean acidification increases the accumulation of toxic phenolic compounds across trophic levels. Nat. Commun. 6, 8714 (2015).
Ferrari, M. C. O. et al. Effects of ocean acidification on learning in coral reef fishes. PLoS ONE 7, e31478 (2012).
Pörtner, H.-O. et al. in Climate Change 2014: Impacts, Adaptation, and Vulnerability (eds Field, C. B. et al.) 411–484 (IPCC, Cambridge Univ. Press, 2014).
Boyd, P. W. & Hutchins, D. A. Understanding the responses of ocean biota to a complex matrix of cumulative anthropogenic change. Mar. Ecol. Progr. Ser. 470, 125–135 (2012).
Gibbs, S. J. et al. Ocean warming, not acidification, controlled coccolithophore response during past greenhouse climate change. Geology 44, 59–62 (2016).
Guinotte, J. & Fabry, V. J. Ocean acidification and its potential effects on marine ecosystems. Ann. NY Acad. Sci. 1134, 320–342 (2008).
Breitburg, D. L. et al. And on top of all that … coping with ocean acidification in the midst of many stressors. Oceanography 28, 48–61 (2015).
Byrne, M. & Przeslawski, R. Multistressor impacts of warming and acidification of the ocean on marine invertebrates’ life histories. Integr. Compar. Biol. 53, 582–596 (2013).
Campbell, J. E., Fisch, J., Langdon, C. & Paul, V. J. Increased temperature mitigates the effects of ocean acidification in calcified green algae (Halimeda spp.). Coral Reefs 35, 357–368 (2016).
Kroeker, K. J., Kordas, R. A. & Harley, C. D. G. Embracing interactions in ocean acidification research: confronting multiple stressor scenarios and context dependence. Biol. Lett. 13, 20160802 (2017).
Fabricius, K. A. et al. Losers and winners in coral reefs acclimatized to elevated carbon dioxide concentrations. Nat. Clim. Change 1, 165–169 (2011).
Collins, S., Rost, B. & Rynearson, T. A. Evolutionary potential of marine phytoplankton under ocean acidification. Evol. Appl. 7, 140–155 (2013).
Feeley, R. A. et al. The combined effects of ocean acidification, mixing, and respiration on pH and carbonate saturation in an urbanized estuary. Estuar. Coast. Shelf Sci. 88, 442–449 (2010).
Gobler, C. J. & Baumann, H. Hypoxia and acidification in ocean ecosystems: coupled dynamics and effects on marine life. Biol. Lett. 12, 20150976 (2016).
Duarte, C. M. et al. Is ocean acidification an open-ocean syndrome? Understanding anthropogenic impacts on seawater pH. Estuar. Coasts 36, 221–236 (2013).
De’ath, G. et al. The 27 year decline of coral cover on the Great Barrier Reef and its causes. Proc. Natl Acad. Sci. USA 109, 17995–17999 (2012).
Boyd, P. W. et al. Experimental strategies to assess the biological ramifications of multiple drivers of global ocean change—a review. Glob. Change Biol. 24, 2239–2261 (2018).
Bach, L. T., Riebesell, U., Gutowska, M. A., Federwisch, L. & Schulz, K. G. A unifying concept of coccolithophore sensitivity to changing carbonate chemistry embedded in an ecological framework. Progr. Oceanogr. 135, 125–138 (2015). This paper attempted to reconcile differences in the observed responses of coccolithophore species by constructing a unifying conceptual physiological framework.
Mollica, N. R. et al. Ocean acidification affects coral growth by reducing skeletal density. Proc. Natl Acad. Sci. USA 115, 1754–1759 (2018).
Kroeker, K. J., Kordas, R. L., Crim, R. N. & Singh, G. G. Meta-analysis reveals negative yet variable effects of ocean acidification on marine organisms. Ecol. Lett. 13, 1419–1434 (2010).
Sokolova, I. M. Energy-limited tolerance to stress as a conceptual framework to integrate the effects of multiple stressors. Integr. Compar. Biol. 53, 597–608 (2013).
Litchman, E. et al. Global biogeochemical impacts of phytoplankton in the past, present, and future: a trait-based perspective. J. Ecol. 103, 1384–1396 (2015).
Magnan, A. K., Colombier, M. & Gattuso, J.-P. Implications of the Paris Agreement for the ocean. Nat. Clim. Change 6, 732–735 (2015).
Gattuso, J.-P. et al. Contrasting futures for ocean and society from different anthropogenic CO2 emissions scenarios. Science 349, aac4722 (2015).
Alsterberg, C., Eklöf, J. S., Gamfeldt, L., Havenhand, J. N. & Sundbäck, K. Consumers mediate the effects of experimental ocean acidification and warming on primary producers. Proc. Natl Acad. Sci. USA 110, 8603–8608 (2013).
Marzloff, M. P. et al. Modelling marine community responses to climate-driven species redistribution to guide monitoring and adaptive ecosystem-based management. Glob. Change Biol. 22, 2462–2474 (2016).
Colt, S. G. & Knapp, G. P. Economic effects of an ocean acidification catastrophe. Am. Econ. Rev. 106, 615–619 (2016).
Schmutter, K., Nash, M. & Dovey, L. Ocean acidification: assessing the vulnerability of socioeconomic systems in Small Island Developing States. Reg. Environ. Change 17, 973–987 (2017).
Mathis, J. T. et al. Ocean acidification risk assessment for Alaska’s fishery sector. Progr. Oceanogr. 136, 71–91 (2015).
Ekstrom, J. A. et al. Vulnerability and adaptation of US shellfisheries to ocean acidification. Nat. Clim. Change 5, 207–214 (2015). This paper assesses the exposure of regional shellfisheries around the United States to OA and a range of issues related to community and policy engagement to address the problem.
Strong, A., Kroeker, K. J., Teneva, L., Mease, L. A. & Kelly, R. Ocean Acidification 2.0: managing our changing coastal ocean chemistry. BioScience 64, 581–592 (2014).
Gelcich, S. et al. Navigating transformations in governance of Chilean marine coastal resources. Proc. Natl Acad. Sci. USA 107, 16794–16799 (2010).
Senate Bill 5603 (State of Washington, 2013); https://go.nature.com/2lGLSNp
Talbert, J. & Niemi, E. in The Encyclopedia of the Anthropocene Vol. 2 (eds DellaSala, D. A. & Goldstein, M. I.) 409–418 (Elsevier, Oxford, 2018).
Billé, R. et al. Taking action against ocean acidification: a review of management and policy options. Environ. Manag. 52, 761–779 (2013).
Gallo, N. D., Victor, D. G. & Levin, L. A. Ocean commitments under the Paris Agreement. Nat. Clim. Change 7, 833–838 (2017).
Transforming Our World: The 2030 Agenda for Sustainable Development A70/1 (United Nations, 2015); http://www.un.org/ga/search/view_doc.asp?symbol=A/RES/70/1
Vierros, M. & Buonomo, R. In-Depth Analysis of Ocean Conference Voluntary Commitments to Support and Monitor Their Implementation (Department of Economic and Social Affairs, United Nations, 2017); https://go.nature.com/2tOzYEQ
Boyd, P. W. et al. Biological responses to environmental heterogeneity under future ocean conditions. Glob. Change Biol. 22, 2633–2650 (2016).
Henson, S. A. et al. Rapid emergence of climate change in environmental drivers of marine ecosystems. Nat. Commun. 8, 14682 (2017).
Visbeck, M. Ocean science research is key for a sustainable future. Nat. Commun. 9, 690 (2018).
Lenton, A., Matear, R. J., Keller, D. P., Scott, V. & Vaughan, N. E. Assessing carbon dioxide removal through global and regional ocean alkalization under high and low emission pathways. Earth Syst. Dynam. 9, 339–357 (2018).
Matear, R. & Lenton, A. Restoration of the oceans. Nat. Clim. Change 5, 1028–1029 (2015).
Hurd, C. L. Slow‐flow habitats as refugia for coastal calcifiers from ocean acidification. J. Phycol. 50, 599–605 (2015).
Allbright, R. et al. Reversal of ocean acidification enhances net coral reef calcification. Nature 531, 362–365 (2016). This paper quantifies the impact of ocean acidification on coral calcification rates since the pre-industrial period, and demonstrates that local ocean alkalinity addition can reverse these changes.
Mucci, A. The solubility of calcite and aragonite in seawater at various salinities, temperatures, and one atmosphere total pressure. Am. J. Sci. 283, 780–799 (1983).
Lenton, A., McInnes, K. L. & O’Grady, J. G. Marine projections of warming and ocean acidification in the Australasian Region. Austr. Meteorol. Oceanogr. J. 65, S1–S28 (2015).
Hofmann, G. E. et al. High-frequency dynamics of ocean pH: a multi-ecosystem comparison. PLoS ONE 6, e28983 (2011).
Hales, B., Suhrbier, A., Waldbusser, G. G., Feely, R. A. & Newton, J. A. The carbonate chemistry of the ‘fattening line” Willapa Bay, 2011–2014. Estuar. Coasts 40, 173–186 (2017).
Hurd, C. L. et al. Metabolically-induced pH fluctuations by some coastal calcifiers exceed projected 22nd century ocean acidification: a mechanism for differential susceptibility? Glob. Change Biol. 17, 2488–2497 (2011).
Wahl, M., Saderne, V. & Sawall, Y. How good are we at assessing the impact of ocean acidification in coastal systems? Limitations, omissions and strengths of commonly used experimental approaches with special emphasis on the neglected role of fluctuations. Mar. Freshwat. Res. 67, 25–36 (2016).
Jiang, L.-Q. et al. Climatological distribution of aragonite saturation state in the global oceans. Glob. Biogeochem. Cycles 29, 1656–1673 (2015).
Acknowledgements
We acknowledge the sponsors and participants of the 4th International Symposium on the Ocean in a High CO2 World and the 3rd Global Ocean Acidification Observing Network workshop that helped define the research needs and directions. This project was supported under Australian Research Council’s Special Research Initiative for Antarctic Gateway Partnership (Project ID SR140300001). P.W.B. acknowledges support from the Australian Research Council (Laureate Fellowship FL160100131). B.T. and P.W.B were supported by Antarctic Climate and Ecosystem Co-operative Research Centre.
Author information
Authors and Affiliations
Contributions
All authors contributed equally.
Corresponding author
Ethics declarations
Competing interests
The authors declare no competing interests.
Additional information
Publisher’s note: Springer Nature remains neutral with regard to jurisdictional claims in published maps and institutional affiliations.
Rights and permissions
About this article
Cite this article
Hurd, C.L., Lenton, A., Tilbrook, B. et al. Current understanding and challenges for oceans in a higher-CO2 world. Nature Clim Change 8, 686–694 (2018). https://doi.org/10.1038/s41558-018-0211-0
Received:
Revised:
Accepted:
Published:
Issue Date:
DOI: https://doi.org/10.1038/s41558-018-0211-0
This article is cited by
-
Habitat sensitivity in the West African coastal area: inferences and implications for regional adaptations to climate change and ocean acidification
Environmental Monitoring and Assessment (2024)
-
Water motion and pH jointly impact the availability of dissolved inorganic carbon to macroalgae
Scientific Reports (2022)
-
Conversion of CO2 to Multi-carbon Compounds over a CoCO3 Supported Ru-Pt Catalyst Under Mild Conditions
Chemical Research in Chinese Universities (2022)
-
Water at charged interfaces
Nature Reviews Chemistry (2021)
-
Influence of Acidification and Warming of Seawater on Biofouling by Bacteria Grown over API 5L Steel
Indian Journal of Microbiology (2021)