Abstract
Recombinant adeno-associated virus (AAV)-2 has significant potential as a delivery vehicle of therapeutic genes to retinal ganglion cells (RGCs), which are key interventional targets in optic neuropathies. Here we show that when injected intravitreally, AAV2 engineered with a reporter gene driven by cytomegalovirus (CMV) enhancer and chicken β-actin (CBA) promoters, displays ubiquitous and high RGC expression, similar to its synthetic derivative AAV8BP2. A novel AAV2 vector combining the promoter of the human RGC-selective γ-synuclein (hSNCG) gene and woodchuck hepatitis post-transcriptional regulatory element (WPRE) inserted upstream and downstream of a reporter gene, respectively, induces widespread transduction and strong transgene expression in RGCs. High transduction efficiency and selectivity to RGCs is further achieved by incorporating in the vector backbone a leading CMV enhancer and an SV40 intron at the 5’ and 3’ ends, respectively, of the reporter gene. As a delivery vehicle of hSIRT1, a 2.2-kb therapeutic gene with anti-apoptotic, anti-inflammatory and anti-oxidative stress properties, this recombinant vector displayed improved transduction efficiency, a strong, widespread and selective RGC expression of hSIRT1, and increased RGC survival following optic nerve crush. Thus, AAV2 vector carrying hSNCG promoter with additional regulatory sequences may offer strong potential for enhanced effects of candidate gene therapies targeting RGCs.
This is a preview of subscription content, access via your institution
Access options
Subscribe to this journal
Receive 12 print issues and online access
$259.00 per year
only $21.58 per issue
Buy this article
- Purchase on Springer Link
- Instant access to full article PDF
Prices may be subject to local taxes which are calculated during checkout





Similar content being viewed by others
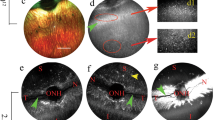
Data availability
The datasets generated and analyzed during the course of this study are available from the corresponding authors on reasonable request.
References
Wong KA, Benowitz LI. Retinal ganglion cell survival and axon regeneration after optic nerve injury: role of inflammation and other factors. Int J Mol Sci. 2022;23:10179.
Sanz-Morello B, Ahmadi H, Vohra R, Saruhanian S, Freude KK, Hamann S, et al. Oxidative stress in optic neuropathies. Antioxidants. 2021;10:1538.
Newman NJ, Yu-Wai-Man P, Biousse V, Carelli V. Understanding the molecular basis and pathogenesis of hereditary optic neuropathies: towards improved diagnosis and management. Lancet Neurol. 2023;22:172–88.
Quigley HA. Glaucoma. Lancet. 2011;377:1367–77.
Shu DY, Chaudhary S, Cho KS, Lennikov A, Miller WP, Thorn DC, et al. Role of oxidative stress in ocular diseases: a balancing act. Metabolites. 2023;13:187.
Fague L, Liu YA, Marsh-Armstrong N. The basic science of optic nerve regeneration. Ann Transl Med. 2021;9:1276.
Levin LA, Patrick C, Choudry NB, Sharif NA, Goldberg JL. Neuroprotection in neurodegenerations of the brain and eye: lessons from the past and directions for the future. Front Neurol. 2022;13:964197.
Wang D, Tai PWL, Gao G. Adeno-associated virus vector as a platform for gene therapy delivery. Nat Rev Drug Discov. 2019;18:358–78.
Zinn E, Vandenberghe LH. Adeno-associated virus: fit to serve. Curr Opin Virol. 2014;8:90–7.
Cwerman-Thibault H, Augustin S, Ellouze S, Sahel JA, Corral-Debrinski M. Gene therapy for mitochondrial diseases: leber hereditary optic neuropathy as the first candidate for a clinical trial. C R Biol. 2014;337:193–206.
Martin KR, Quigley HA. Gene therapy for optic nerve disease. Eye. 2004;18:1049–55.
Mak KY, Rajapaksha IG, Angus PW, Herath CB. The adeno-associated virus - a safe and promising vehicle for liverspecific gene therapy of inherited and non-inherited disorders. Curr Gene Ther. 2017;17:4–16.
Bennett J. Taking stock of retinal gene therapy: looking back and moving forward. Mol Ther. 2017;25:1076–94.
Gao J, Hussain RM, Weng CY. Voretigene neparvovec in retinal diseases: a review of the current clinical evidence. Clin Ophthalmol. 2020;14:3855–69.
Russell S, Bennett J, Wellman JA, Chung DC, Yu ZF, Tillman A, et al. Efficacy and safety of voretigene neparvovec (AAV2-hRPE65v2) in patients with RPE65-mediated inherited retinal dystrophy: a randomised, controlled, open-label, phase 3 trial. Lancet. 2017;390:849–60.
Buning H, Perabo L, Coutelle O, Quadt-Humme S, Hallek M. Recent developments in adeno-associated virus vector technology. J Gene Med. 2008;10:717–33.
Srivastava A. In vivo tissue-tropism of adeno-associated viral vectors. Curr Opin Virol. 2016;21:75–80.
O’Donnell J, Taylor KA, Chapman MS. Adeno-associated virus-2 and its primary cellular receptor–Cryo-EM structure of a heparin complex. Virology. 2009;385:434–43.
Hadaczek P, Mirek H, Bringas J, Cunningham J, Bankiewicz K. Basic fibroblast growth factor enhances transduction, distribution, and axonal transport of adeno-associated virus type 2 vector in rat brain. Hum Gene Ther. 2004;15:469–79.
Kashiwakura Y, Tamayose K, Iwabuchi K, Hirai Y, Shimada T, Matsumoto K, et al. Hepatocyte growth factor receptor is a coreceptor for adeno-associated virus type 2 infection. J Virol. 2005;79:609–14.
Asokan A, Hamra JB, Govindasamy L, Agbandje-McKenna M, Samulski RJ. Adeno-associated virus type 2 contains an integrin alpha5beta1 binding domain essential for viral cell entry. J Virol. 2006;80:8961–9.
Kurzeder C, Koppold B, Sauer G, Pabst S, Kreienberg R, Deissler H. CD9 promotes adeno-associated virus type 2 infection of mammary carcinoma cells with low cell surface expression of heparan sulphate proteoglycans. Int J Mol Med. 2007;19:325–33.
Van Vliet KM, Blouin V, Brument N, Agbandje-McKenna M, Snyder RO. The role of the adeno-associated virus capsid in gene transfer. Methods Mol Biol. 2008;437:51–91.
Rabinowitz JE, Rolling F, Li C, Conrath H, Xiao W, Xiao X, et al. Cross-packaging of a single adeno-associated virus (AAV) type 2 vector genome into multiple AAV serotypes enables transduction with broad specificity. J Virol. 2002;76:791–801.
Ramachandran PS, Lee V, Wei Z, Song JY, Casal G, Cronin T, et al. Evaluation of dose and safety of AAV7m8 and AAV8BP2 in the non-human primate retina. Hum Gene Ther. 2017;28:154–67.
Ross AG, Chaqour B, McDougald DS, Dine KE, Duong TT, Shindler RE, et al. Selective upregulation of sirt1 expression in retinal ganglion cells by AAV-mediated gene delivery increases neuronal cell survival and alleviates axon demyelination associated with optic neuritis. Biomolecules. 2022;12:830.
Yue J, Khan RS, Duong TT, Dine KE, Cui QN, O’Neill N, et al. Cell-specific expression of human sirt1 by gene therapy reduces retinal ganglion cell loss induced by elevated intraocular pressure. Neurotherapeutics. 2023;20:896–907.
McDougald DS, Dine KE, Zezulin AU, Bennett J, Shindler KS. SIRT1 and NRF2 gene transfer mediate distinct neuroprotective effects upon retinal ganglion cell survival and function in experimental optic neuritis. Invest Ophthalmol Vis Sci. 2018;59:1212–20.
Alam F, Syed H, Amjad S, Baig M, Khan TA, Rehman R. Interplay between oxidative stress, SIRT1, reproductive and metabolic functions. Curr Res Physiol. 2021;4:119–24.
Singh V, Ubaid S. Role of silent information regulator 1 (SIRT1) in regulating oxidative stress and inflammation. Inflammation. 2020;43:1589–98.
Li L, Zhi D, Cheng R, Li J, Luo C, Li H. The neuroprotective role of SIRT1/PGC-1alpha signaling in limb postconditioning in cerebral ischemia/reperfusion injury. Neurosci Lett. 2021;749:135736.
Nita M, Grzybowski A. Interplay between reactive oxygen species and autophagy in the course of age-related macular degeneration. EXCLI J. 2020;19:1353–71.
Samarin J, Wessel J, Cicha I, Kroening S, Warnecke C, Goppelt-Struebe M. FoxO proteins mediate hypoxic induction of connective tissue growth factor in endothelial cells. J Biol Chem. 2010;285:4328–36.
Chaffiol A, Caplette R, Jaillard C, Brazhnikova E, Desrosiers M, Dubus E, et al. A new promoter allows optogenetic vision restoration with enhanced sensitivity in macaque retina. Mol Ther. 2017;25:2546–60.
Bennicelli J, Wright JF, Komaromy A, Jacobs JB, Hauck B, Zelenaia O, et al. Reversal of blindness in animal models of Leber congenital amaurosis using optimized AAV2-mediated gene transfer. Mol Ther. 2008;16:458–65.
Ross AG, McDougald DS, Khan RS, Duong TT, Dine KE, Aravand P, et al. Rescue of retinal ganglion cells in optic nerve injury using cell-selective AAV mediated delivery of SIRT1. Gene Ther. 2021;28:256–64.
Zuo L, Khan RS, Lee V, Dine K, Wu W, Shindler KS. SIRT1 promotes RGC survival and delays loss of function following optic nerve crush. Invest Ophthalmol Vis Sci. 2013;54:5097–102.
Cronin T, Vandenberghe LH, Hantz P, Juttner J, Reimann A, Kacso AE, et al. Efficient transduction and optogenetic stimulation of retinal bipolar cells by a synthetic adeno-associated virus capsid and promoter. EMBO Mol Med. 2014;6:1175–90.
Xu L, Daly T, Gao C, Flotte TR, Song S, Byrne BJ, et al. CMV-beta-actin promoter directs higher expression from an adeno-associated viral vector in the liver than the cytomegalovirus or elongation factor 1 alpha promoter and results in therapeutic levels of human factor X in mice. Hum Gene Ther. 2001;12:563–73.
Boye SE, Alexander JJ, Witherspoon CD, Boye SL, Peterson JJ, Clark ME, et al. Highly efficient delivery of adeno-associated viral vectors to the primate retina. Hum Gene Ther. 2016;27:580–97.
Loeb JE, Cordier WS, Harris ME, Weitzman MD, Hope TJ. Enhanced expression of transgenes from adeno-associated virus vectors with the woodchuck hepatitis virus posttranscriptional regulatory element: implications for gene therapy. Hum Gene Ther. 1999;10:2295–305.
Popa I, Harris ME, Donello JE, Hope TJ. CRM1-dependent function of a cis-acting RNA export element. Mol Cell Biol. 2002;22:2057–67.
Graveley BR, Hertel KJ, Maniatis T. The role of U2AF35 and U2AF65 in enhancer-dependent splicing. RNA. 2001;7:806–18.
Xu DH, Wang XY, Jia YL, Wang TY, Tian ZW, Feng X, et al. SV40 intron, a potent strong intron element that effectively increases transgene expression in transfected Chinese hamster ovary cells. J Cell Mol Med. 2018;22:2231–9.
Rotondaro L, Mele A, Rovera G. Efficiency of different viral promoters in directing gene expression in mammalian cells: effect of 3’-untranslated sequences. Gene. 1996;168:195–8.
Xu ZL, Mizuguchi H, Ishii-Watabe A, Uchida E, Mayumi T, Hayakawa T. Optimization of transcriptional regulatory elements for constructing plasmid vectors. Gene. 2001;272:149–56.
Hermonat PL, Quirk JG, Bishop BM, Han L. The packaging capacity of adeno-associated virus (AAV) and the potential for wild-type-plus AAV gene therapy vectors. FEBS Lett. 1997;407:78–84.
Tenenbaum L, Chtarto A, Lehtonen E, Velu T, Brotchi J, Levivier M. Recombinant AAV-mediated gene delivery to the central nervous system. J Gene Med. 2004;6:S212–22.
Prosch S, Stein J, Staak K, Liebenthal C, Volk HD, Kruger DH. Inactivation of the very strong HCMV immediate early promoter by DNA CpG methylation in vitro. Biol Chem Hoppe Seyler. 1996;377:195–201.
Chintalapudi SR, Morales-Tirado VM, Williams RW, Jablonski MM. Multipronged approach to identify and validate a novel upstream regulator of Sncg in mouse retinal ganglion cells. FEBS J. 2016;283:678–93.
Claes M, Moons L. Retinal ganglion cells: global number, density and vulnerability to glaucomatous injury in common laboratory mice. Cells. 2022;11:2689.
Rodriguez AR, de Sevilla Muller LP, Brecha NC. The RNA binding protein RBPMS is a selective marker of ganglion cells in the mammalian retina. J Comp Neurol. 2014;522:1411–43.
Wang Q, Zhuang P, Huang H, Li L, Liu L, Webber HC, et al. Mouse gamma-synuclein promoter-mediated gene expression and editing in mammalian retinal ganglion cells. J Neurosci. 2020;40:3896–914.
Ge J, Jin L, Tang X, Gao D, An Q, Ping W. Optimization of eGFP expression using a modified baculovirus expression system. J Biotechnol. 2014;173:41–6.
Martin KR, Quigley HA, Zack DJ, Levkovitch-Verbin H, Kielczewski J, Valenta D, et al. Gene therapy with brain-derived neurotrophic factor as a protection: retinal ganglion cells in a rat glaucoma model. Invest Ophthalmol Vis Sci. 2003;44:4357–65.
Powell SK, Rivera-Soto R, Gray SJ. Viral expression cassette elements to enhance transgene target specificity and expression in gene therapy. Discov Med. 2015;19:49–57.
Wang L, Wang Z, Zhang F, Zhu R, Bi J, Wu J, et al. Enhancing transgene expression from recombinant AAV8 vectors in different tissues using woodchuck hepatitis virus post-transcriptional regulatory element. Int J Med Sci. 2016;13:286–91.
Gruh I, Wunderlich S, Winkler M, Schwanke K, Heinke J, Blomer U, et al. Human CMV immediate-early enhancer: a useful tool to enhance cell-type-specific expression from lentiviral vectors. J Gene Med. 2008;10:21–32.
Ghazal P, Lubon H, Hennighausen L. Multiple sequence-specific transcription factors modulate cytomegalovirus enhancer activity in vitro. Mol Cell Biol. 1988;8:1809–11.
Le Hir H, Nott A, Moore MJ. How introns influence and enhance eukaryotic gene expression. Trends Biochem Sci. 2003;28:215–20.
Huang MT, Gorman CM. Intervening sequences increase efficiency of RNA 3’ processing and accumulation of cytoplasmic RNA. Nucleic Acids Res. 1990;18:937–47.
Fonseca-Kelly Z, Nassrallah M, Uribe J, Khan RS, Dine K, Dutt M, et al. Resveratrol neuroprotection in a chronic mouse model of multiple sclerosis. Front Neurol. 2012;3:84.
Shindler KS, Ventura E, Rex TS, Elliott P, Rostami A. SIRT1 activation confers neuroprotection in experimental optic neuritis. Invest Ophthalmol Vis Sci. 2007;48:3602–9.
Acknowledgements
AAV vectors used in this study were produced and packaged by the Research Vector Core at the Center for Advanced Retinal and Ocular Therapeutics (University of Pennsylvania).
Funding
This study is supported, at least in part, by grants from Gyroscope Therapeutics Limited, a Novartis Company; National Institutes of Health Grants (EY019014, EY030163); Department of Defense (USAMRAA) award number HT9425-23-1-0725; RWJ-Harold Amos Faculty Development; Linda Pechenik Montague Investigator Award; Research to Prevent Blindness; Paul and Evanina Mackall Foundation Trust; Center for Advanced Retinal and Ocular Therapeutics; and the F. M. Kirby Foundation.
Author information
Authors and Affiliations
Contributions
BC: data curation, formal analysis, supervision, writing original draft, review and editing. TTD: investigation, methodology. JPY: methodology, data extraction, formal data analysis. TL: methodology, validation. DC: data curation. KED: methodology, formal analysis, investigation. JER: conceptualization, review. SE: conceptualization, review. JB: conceptualization, review. KSS: conceptualization, data curation, formal analysis, funding acquisition, investigation, project administration, resources, supervision, review and editing. AGR: project administration, validation, visualization, investigation, methodology, funding acquisition, resources, review and editing.
Corresponding authors
Ethics declarations
Competing interests
AGR and KSS hold intellectual property relevant to this study and received research funding from Gyroscope Therapeutics Limited, a Novartis Company. JE-R and SE are full time employees of Gyroscope. Other authors declare no conflict of interests.
Ethical approval
All experiments were performed in accordance with relevant guidelines of the ARVO Statement for the Use of Animals in Ophthalmic and Vision Research, as well as with institutional and federal regulations.
Additional information
Publisher’s note Springer Nature remains neutral with regard to jurisdictional claims in published maps and institutional affiliations.
Rights and permissions
Springer Nature or its licensor (e.g. a society or other partner) holds exclusive rights to this article under a publishing agreement with the author(s) or other rightsholder(s); author self-archiving of the accepted manuscript version of this article is solely governed by the terms of such publishing agreement and applicable law.
About this article
Cite this article
Chaqour, B., Duong, T.T., Yue, J. et al. AAV2 vector optimization for retinal ganglion cell-targeted delivery of therapeutic genes. Gene Ther 31, 175–186 (2024). https://doi.org/10.1038/s41434-023-00436-8
Received:
Revised:
Accepted:
Published:
Issue Date:
DOI: https://doi.org/10.1038/s41434-023-00436-8