Abstract
Shape-selective monometallic nanocatalysts offer activity benefits based on structural sensitivity and high surface area. In bimetallic nanoalloys with well-defined shape, site-dependent metal surface segregation additionally affects the catalytic activity and stability. However, segregation on shaped alloy nanocatalysts and their atomic-scale evolution is largely unexplored. Exemplified by three octahedral PtxNi1−x alloy nanoparticle electrocatalysts with unique activity for the oxygen reduction reaction at fuel cell cathodes, we reveal an unexpected compositional segregation structure across the {111} facets using aberration-corrected scanning transmission electron microscopy and electron energy-loss spectroscopy. In contrast to theoretical predictions, the pristine PtxNi1−x nano-octahedra feature a Pt-rich frame along their edges and corners, whereas their Ni atoms are preferentially segregated in their {111} facet region. We follow their morphological and compositional evolution in electrochemical environments and correlate this with their exceptional catalytic activity. The octahedra preferentially leach in their facet centres and evolve into ‘concave octahedra’. More generally, the segregation and leaching mechanisms revealed here highlight the complexity with which shape-selective nanoalloys form and evolve under reactive conditions.
This is a preview of subscription content, access via your institution
Access options
Subscribe to this journal
Receive 12 print issues and online access
$259.00 per year
only $21.58 per issue
Buy this article
- Purchase on Springer Link
- Instant access to full article PDF
Prices may be subject to local taxes which are calculated during checkout





Similar content being viewed by others
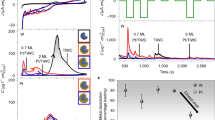
References
Arico, A. S., Bruce, P., Scrosati, B., Tarascon, J. M. & Van Schalkwijk, W. Nanostructured materials for advanced energy conversion and storage devices. Nature Mater. 4, 366–377 (2005).
Sasaki, K. et al. Highly stable Pt monolayer on PdAu nanoparticle electrocatalysts for the oxygen reduction reaction. Nature Commun. 3, 1–5 (2012).
Yu, W. T., Porosoff, M. D. & Chen, J. G. G. Review of Pt-based bimetallic catalysis: From model surfaces to supported catalysts. Chem. Rev. 112, 5780–5817 (2012).
Watanabe, M., Tsurumi, K., Mizukami, T., Nakamura, T. & Stonehart, P. Activity and stability of ordered and disordered Co-Pt alloys for phosphoric-acid fuel-cells. J. Electrochem. Soc. 141, 2659–2668 (1994).
Rabis, A., Rodriguez, P. & Schmidt, T. J. Electrocatalysis for polymer electrolyte fuel cells: Recent achievements and future challenges. ACS Catal. 2, 864–890 (2012).
Debe, M. K. Electrocatalyst approaches and challenges for automotive fuel cells. Nature 486, 43–51 (2012).
Wagner, F. T., Lakshmanan, B. & Mathias, M. F. Electrochemistry and the future of the automobile. J. Phys. Chem. Lett. 1, 2204–2219 (2010).
Stephens, I. E. L. et al. Tuning the activity of Pt(111) for oxygen electroreduction by subsurface alloying. J. Am. Chem. Soc. 133, 5485–5491 (2011).
Toda, T., Igarashi, H., Uchida, H. & Watanabe, M. Enhancement of the electroreduction of oxygen on Pt alloys with Fe, Ni, and Co. J. Electrochem. Soc. 146, 3750–3756 (1999).
Greeley, J. et al. Alloys of platinum and early transition metals as oxygen reduction electrocatalysts. Nature Chem. 1, 552–556 (2009).
Snyder, J., Fujita, T., Chen, M. W. & Erlebacher, J. Oxygen reduction in nanoporous metal–ionic liquid composite electrocatalysts. Nature Mater. 9, 904–907 (2010).
Liu, Z., Yu, C., Rusakova, I. A., Huang, D. & Strasser, P. Synthesis of Pt3Co alloy nanocatalyst via reverse micelle for oxygen reduction reaction in PEMFCs. Top. Catal. 49, 241–250 (2008).
Stamenkovic, V. R. et al. Improved oxygen reduction activity on Pt3Ni(111) via increased surface site availability. Science 315, 493–497 (2007).
Stamenkovic, V. R. et al. Trends in electrocatalysis on extended and nanoscale Pt-bimetallic alloy surfaces. Nature Mater. 6, 241–247 (2007).
Carpenter, M. K., Moylan, T. E., Kukreja, R. S., Atwan, M. H. & Tessema, M. M. Solvothermal synthesis of platinum alloy nanoparticles for oxygen reduction electrocatalysis. J. Am. Chem. Soc. 134, 8535–8542 (2012).
Zhang, J., Yang, H., Fang, J. & Zou, S. Synthesis and oxygen reduction activity of shape-controlled Pt3Ni nanopolyhedra. Nano Lett. 10, 638–644 (2010).
Wu, J. B., Gross, A. & Yang, H. Shape and composition-controlled platinum alloy nanocrystals using carbon monoxide as reducing agent. Nano Lett. 11, 798–802 (2011).
Wu, J. et al. Truncated octahedral Pt3Ni oxygen reduction reaction electrocatalysts. J. Am. Chem. Soc. 132, 4984–4985 (2010).
Wu, Y., Cai, S., Wang, D., He, W. & Li, Y. Syntheses of water-soluble octahedral, truncated octahedral, and cubic Pt–Ni nanocrystals and their structure–activity study in model hydrogenation reactions. J. Am. Chem. Soc. 134, 8975–8981 (2012).
Gasteiger, H. A. & Markovic, N. M. Just a dream-or future reality? Science 324, 48–49 (2009).
Gan, L., Heggen, M., Rudi, S. & Strasser, P. Core-shell compositional fine structures of dealloyed PtxNi1−x nanoparticles and their impact on oxygen reduction catalysis. Nano Lett. 12, 5423–5430 (2012).
Strasser, P. et al. Lattice-strain control of the activity in dealloyed core-shell fuel cell catalysts. Nature Chem. 2, 454–460 (2010).
Van der Vliet, D. F. et al. Mesostructured thin films as electrocatalysts with tunable composition and surface morphology. Nature Mater. 11, 1051–1058 (2012).
Colon-Mercado, H. R. & Popov, B. N. Stability of platinum based alloy cathode catalysts in PEM fuel cells. J. Power Sources 155, 253–263 (2006).
Wang, D. S., Zhao, P. & Li, Y. D. General preparation for Pt-based alloy nanoporous nanoparticles as potential nanocatalysts. Sci. Rep. 1, 1–5 (2011).
Snyder, J., McCue, I., Livi, K. & Erlebacher, J. Structure/processing/properties relationships in nanoporous nanoparticles as applied to catalysis of the cathodic oxygen reduction reaction. J. Am. Chem. Soc. 134, 8633–8645 (2012).
Wu, Y. et al. A strategy for designing a concave Pt–Ni alloy through controllable chemical etching. Angew. Chem. Int. Ed. 51, 12524–12528 (2012).
Oezaslan, M., Heggen, M. & Strasser, P. Size-dependent morphology of dealloyed bimetallic catalysts: linking the nano to the macro scale. J. Am. Chem. Soc. 134, 514–524 (2011).
Strasser, P. Dealloyed core-shell fuel cell electrocatalysts. Rev. Chem. Eng. 25, 255–295 (2009).
Wang, C. et al. Correlation between surface chemistry and electrocatalytic properties of monodisperse PtxNi1−x nanoparticles. Adv. Funct. Mater. 21, 147–152 (2011).
Wang, G. F., Van Hove, M. A., Ross, P. N. & Baskes, M. I. Monte Carlo simulations of segregation in Pt–Ni catalyst nanoparticles. J. Chem. Phys. 122, 024706 (2005).
Urban, K. W. Studying atomic structures by aberration-corrected transmission electron microscopy. Science 321, 506–510 (2008).
Van der Vliet, D. F. et al. Unique electrochemical adsorption properties of Pt-skin surfaces. Angew. Chem. Int. Ed. 51, 3139–3142 (2012).
Colon-Mercado, H. R., Kim, H. & Popov, B. N. Durability study of Pt3Ni1 catalysts as cathode in PEM fuel cells. Electrochem. Commun. 6, 795–799 (2004).
Antolini, E., Salgado, J. R. C. & Gonzalez, E. R. The stability of Pt-M (M = first row transition metal) alloy catalysts and its effect on the activity in low temperature fuel cells—a literature review and tests on a Pt–Co catalyst. J. Power Sources 160, 957–968 (2006).
Chen, S., Gasteiger, H. A., Hayakawa, K., Tada, T. & Shao-Horn, Y. Platinum-alloy cathode catalyst degradation in proton exchange membrane fuel cells: Nanometer-scale compositional and morphological changes. J. Electrochem. Soc. 157, A82–A97 (2010).
Snyder, J. & Erlebacher, J. Kinetics of crystal etching limited by terrace dissolution. J. Electrochem. Soc. 157, C125–C130 (2010).
Dubau, L., Maillard, F., Chatenet, M., Andre, J. & Rossinot, E. Nanoscale compositional changes and modification of the surface reactivity of Pt3Co/C nanoparticles during proton-exchange membrane fuel cell operation. Electrochim. Acta 56, 776–783 (2010).
Dubau, L. et al. Further insights into the durability of Pt3Co/C electrocatalysts: Formation of ‘hollow’ Pt nanoparticles induced by the Kirkendall effect. Electrochim. Acta 56, 10658–10667 (2011).
Wang, C. et al. Design and synthesis of bimetallic electrocatalyst with multilayered Pt-Skin surfaces. J. Am. Chem. Soc. 133, 14396–14403 (2011).
Wang, D. et al. Structurally ordered intermetallic platinum–cobalt core–shell nanoparticles with enhanced activity and stability as oxygen reduction electrocatalysts. Nature Mater. 12, 81–87 (2013).
Zhang, J., Vukmirovic, M. B., Xu, Y., Mavrikakis, M. & Adzic, R. R. Controlling the catalytic activity of platinum-monolayer electrocatalysts for oxygen reduction with different substrates. Angew. Chem. Int. Ed. 44, 2132–2135 (2005).
Cui, C. H., Li, H. H., Liu, X. J., Gao, M. R. & Yu, S. H. Surface composition and lattice ordering-controlled activity and durability of CuPt electrocatalysts for oxygen reduction reaction. ACS Catal. 2, 916–924 (2012).
Kolb, D. M. Reconstruction phenomena at metal-electrolyte interfaces. Prog. Surf. Sci. 51, 109–173 (1996).
Cui, C. et al. Octahedral PtNi nanoparticle catalysts: Exceptional oxygen reduction activity by tuning the alloy particle surface composition. Nano Lett. 12, 5885–5889 (2012).
Heggen, M., Oezaslan, M., Houben, L. & Strasser, P. Formation and analysis of core–shell fine structures in Pt bimetallic nanoparticle fuel cell electrocatalysts. J. Phys. Chem. C 116, 19073–19083 (2012).
Mayrhofer, K. J. J., Juhart, V., Hartl, K., Hanzlik, M. & Arenz, M. Adsorbate-induced surface segregation for core-shell nanocatalysts. Angew. Chem. Int. Ed. 48, 3529–3531 (2009).
Acknowledgements
We thank the Zentraleinrichtung für Elektronenmikroskopie (Zelmi) of the Technical University Berlin for their support with TEM and energy-dispersive X-ray spectra techniques. This work was supported by US DOE EERE award DE-EE0000458 via subcontract through General Motors. P.S. acknowledges financial support through the cluster of excellence in catalysis (UniCat).
Author information
Authors and Affiliations
Contributions
P.S. and C.C. conceived and designed the experiments. C.C. carried out the chemical synthesis and the electrochemical experiments and analysed the results. L.G. and M.H. performed the HRTEM and STEM-EELS experiments, evaluated and analysed the results. P.S. and C.C. aggregated the figures and co-wrote the manuscript. All authors discussed the results, drew conclusions and commented on the manuscript.
Corresponding author
Ethics declarations
Competing interests
The authors declare no competing financial interests.
Supplementary information
Supplementary Information
Supplementary Information (PDF 1623 kb)
Rights and permissions
About this article
Cite this article
Cui, C., Gan, L., Heggen, M. et al. Compositional segregation in shaped Pt alloy nanoparticles and their structural behaviour during electrocatalysis. Nature Mater 12, 765–771 (2013). https://doi.org/10.1038/nmat3668
Received:
Accepted:
Published:
Issue Date:
DOI: https://doi.org/10.1038/nmat3668
This article is cited by
-
Identifying the distinct roles of dual dopants in stabilizing the platinum-nickel nanowire catalyst for durable fuel cell
Nature Communications (2024)
-
Nickel Sulfide Modified NiCu Nanoalloy with Excellent Oxygen Evolution Reaction Properties Prepared through Electrospinning and Heat Treatment
Journal of Wuhan University of Technology-Mater. Sci. Ed. (2024)
-
Low-temperature non-equilibrium synthesis of anisotropic multimetallic nanosurface alloys for electrochemical CO2 reduction
Nature Synthesis (2023)
-
Grooved electrodes for high-power-density fuel cells
Nature Energy (2023)
-
Shape-Controlled Synthesis of Platinum-Based Nanocrystals and Their Electrocatalytic Applications in Fuel Cells
Nano-Micro Letters (2023)