Abstract
Objectives:
To examine the associations of antibiotic exposures during the first 2 years of life and the development of body mass over the first 7 years of life.
Design:
Longitudinal birth cohort study.
Subjects:
A total of 11 532 children born at ⩾2500 g in the Avon Longitudinal Study of Parents and Children (ALSPAC), a population-based study of children born in Avon, UK in 1991–1992.
Measurements:
Exposures to antibiotics during three different early-life time windows (<6 months, 6–14 months, 15–23 months), and indices of body mass at five time points (6 weeks, 10 months, 20 months, 38 months and 7 years).
Results:
Antibiotic exposure during the earliest time window (<6 months) was consistently associated with increased body mass (+0.105 and +0.083 s.d. unit, increase in weight-for-length Z-scores at 10 and 20 months, P<0.001 and P=0.001, respectively; body mass index (BMI) Z-score at 38 months +0.067 s.d. units, P=0.009; overweight OR 1.22 at 38 months, P=0.029) in multivariable, mixed-effect models controlling for known social and behavioral obesity risk factors. Exposure from 6 to 14 months showed no association with body mass, while exposure from 15 to 23 months was significantly associated with increased BMI Z-score at 7 years (+0.049 s.d. units, P=0.050). Exposures to non-antibiotic medications were not associated with body mass.
Conclusions:
Exposure to antibiotics during the first 6 months of life is associated with consistent increases in body mass from 10 to 38 months. Exposures later in infancy (6–14 months, 15–23 months) are not consistently associated with increased body mass. Although effects of early exposures are modest at the individual level, they could have substantial consequences for population health. Given the prevalence of antibiotic exposures in infants, and in light of the growing concerns about childhood obesity, further studies are needed to isolate effects and define life-course implications for body mass and cardiovascular risks.
This is a preview of subscription content, access via your institution
Access options
Subscribe to this journal
Receive 12 print issues and online access
$259.00 per year
only $21.58 per issue
Buy this article
- Purchase on Springer Link
- Instant access to full article PDF
Prices may be subject to local taxes which are calculated during checkout


Similar content being viewed by others
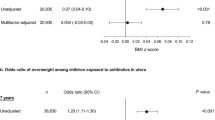
References
Jayachandran S, Lleras-Muney A, Smith KV . Modern Medicine and the 20th Century Decline in Mortality: Evidence on the Impact of Sulfa Drugs 2009. The National Bureau of Economic Research. NBER Working Paper Series No. 15089.
McCaig LF, Besser RE, Hughes JM . Trends in antimicrobial prescribing rates for children and adolescents. JAMA 2002; 287: 3096–3102.
Finkelstein JA, Metlay JP, Davis RL, Rifas-Shiman SL, Dowell SF, Platt R . Antimicrobial use in defined populations of infants and young children. Arch Pediatr Adolesc Med 2000; 154: 395–400.
Hicks LA, Chien YW, Taylor TH, Haber M, Klugman KP . Outpatient antibiotic prescribing and nonsusceptible Streptococcus pneumoniae in the United States, 1996-2003. Clin Infect Dis 2011; 53: 631–639.
Paul IM, Maselli JH, Hersh AL, Boushey HA, Nielson DW, Cabana MD . Antibiotic prescribing during pediatric ambulatory care visits for asthma. Pediatrics 2011; 127: 1014–1021.
Coco A, Vernacchio L, Horst M, Anderson A . Management of acute otitis media after publication of the 2004 AAP and AAFP clinical practice guideline. Pediatrics 2010; 125: 214–220.
Finkelstein JA, Stille C, Nordin J, Davis R, Raebel MA, Roblin D et al. Reduction in antibiotic use among US children, 1996-2000. Pediatrics 2003; 112: 620–627.
Grijalva CG, Nuorti JP, Griffin MR . Antibiotic prescription rates for acute respiratory tract infections in US ambulatory settings. JAMA 2009; 302: 758–766.
Thompson PL, Gilbert RE, Long PF, Saxena S, Sharland M, Wong IC et al. guidance affected general practitioner antibiotic prescribing for otitis media in children? J Public Health 2008; 30: 479–486.
Jukes TH . Antibiotics in animal feeds and animal production. Bioscience 1972; 22: 526–534.
Millar MR, Walsh TR, Linton CJ, Zhang S, Leeming JP, Bennett PM . Carriage of antibiotic-resistant bacteria by healthy children. J Antimicrob Chemother 2001; 47: 605–610.
Sjolund M, Wreiber K, Andersson DI, Blaser MJ, Engstrand L . Long-term persistence of resistant Enterococcus species after antibiotics to eradicate Helicobacter pylori. Ann Intern Med 2003; 139: 483–487.
Blaser MJ, Falkow S . What are the consequences of the disappearing human microbiota? Nat Rev Microbiol 2009; 7: 887–894.
Blaser M . Antibiotic overuse: stop the killing of beneficial bacteria. Nature 2011; 476: 393–394.
Dethlefsen L, Relman DA . Incomplete recovery and individualized responses of the human distal gut microbiota to repeated antibiotic perturbation. Proc Natl Acad Sci USA 2011; 108: 4554–4561.
Strachan DP . Family size, infection and atopy: the first decade of the "hygiene hypothesis". Thorax 2000; 55: S2–10.
Taylor AL, Dunstan JA, Prescott SL . Probiotic supplementation for the first 6 months of life fails to reduce the risk of atopic dermatitis and increases the risk of allergen sensitization in high-risk children: a randomized controlled trial. J Allergy Clin Immunol 2007; 119: 184–191.
Viljanen M, Savilahti E, Haahtela T, Juntunen-Backman K, Korpela R, Poussa T et al. Probiotics in the treatment of atopic eczema/dermatitis syndrome in infants: a double-blind placebo-controlled trial. Allergy 2005; 60: 494–500.
Kozyrskyj AL, Ernst P, Becker AB . Increased risk of childhood asthma from antibiotic use in early life. Chest 2007; 131: 1753–1759.
Wickens K, Pearce N, Crane J, Beasley R . Antibiotic use in early childhood and the development of asthma. Clin Exp Allergy 1999; 29: 766–771.
Hviid A, Svanstrom H, Frisch M . Antibiotic use and inflammatory bowel diseases in childhood. Gut 2011; 60: 49–54.
Dominguez-Bello MG, Costello EK, Contreras M, Magris M, Hidalgo G, Fierer N et al. Delivery mode shapes the acquisition and structure of the initial microbiota across multiple body habitats in newborns. Proc Natl Acad Sci USA 2010; 107: 11971–11975.
Palmer C, Bik EM, DiGiulio DB, Relman DA, Brown PO . Development of the human infant intestinal microbiota. PLoS Biol 2007; 5: e177.
Spor A, Koren O, Ley R . Unravelling the effects of the environment and host genotype on the gut microbiome. Nat Rev Microbiol 2011; 9: 279–290.
Dubos R, Savage D, Schaedler R . Biological Freudianism: lasting effects of early environmental influences. 1966. Int J Epidemiol 2005; 34: 5–12.
Dubos R, Schaedler RW, Stephens M . The effect of antibacterial drugs on the fecal flora of mice. J Exp Med 1963; 117: 231–243.
Bedford Russell AR, Murch SH . Could peripartum antibiotics have delayed health consequences for the infant? BJOG 2006; 113: 758–765.
Ley RE, Lozupone CA, Hamady M, Knight R, Gordon JI . Worlds within worlds: evolution of the vertebrate gut microbiota. Nat Rev Microbiol 2008; 6: 776–788.
Cromwell GL . Why and how antibiotics are used in swine production. Anim Biotechnol 2002; 13: 7–27.
Gaskins HR, Collier CT, Anderson DB . Antibiotics as growth promotants: mode of action. Anim Biotechnol 2002; 13: 29–42.
Libby DA, Schaible PJ . Observations on growth responses to antibiotics and arsonic acids in poultry feeds. Science 1955; 121: 733–734.
Lassiter CA . Antibiotics as growth stimulant for dairy cattle: a review. J Dairy Science 1955; 38: 1102–1138.
Ajslev TA, Andersen CS, Gamborg M, Sorensen TI, Jess T . Childhood overweight after establishment of the gut microbiota: the role of delivery mode, pre-pregnancy weight and early administration of antibiotics. Int J Obes 2011; 35: 522–529.
Headley J, Northstone K . Medication administered to children from 0 to 7.5 years in the Avon Longitudinal Study of Parents and Children (ALSPAC). Eur J Clin Pharmacol 2007; 63: 189–195.
Golding J, Pembrey M, Jones R . ALSPAC--the Avon Longitudinal Study of Parents and Children. I. Study methodology. Paediatr Perinat Epidemiol 2001; 15: 74–87.
Cole TJ, Bellizzi MC, Flegal KM, Dietz WH . Establishing a standard definition for child overweight and obesity worldwide: international survey. BMJ 2000; 320: 1240–1243.
de Onis M . WHO Multicentre Growth Reference Study Group WHO Child Growth Standards based on length/height, weight and age. Acta Paediatr 2006; 95: 76–85.
Barker DJ, Godfrey KM, Osmond C, Bull A . The relation of fetal length, ponderal index and head circumference to blood pressure and the risk of hypertension in adult life. Paediatr Perinat Epidemiol 1992; 6: 35–44.
Grummer-Strawn LM, Reinold C, Krebs NF . Use of World Health Organization and CDC growth charts for children aged 0–59 months in the United States. MMWR Recomm Rep 2010; 59: 1–15.
Ogden CL, Kuczmarski RJ, Flegal KM, Mei Z, Guo S, Wei R et al. Centers for Disease Control and Prevention 2000 growth charts for the United States: improvements to the 1977 National Center for Health Statistics version. Pediatrics 2002; 109: 45–60.
Cole TJ, Freeman JV, Preece MA . British 1990 growth reference centiles for weight, height, body mass index and head circumference fitted by maximum penalized likelihood. Stat Med 1998; 17: 407–429.
Reilly JJ, Armstrong J, Dorosty AR, Emmett PM, Ness A, Rogers I et al. Early life risk factors for obesity in childhood: cohort study. BMJ 2005; 330: 1357.
OPCS Standard Occupational Classification vol. 3. HMSO: London, 1991.
Office of Qualifications and Examinations Regulations Explaining the National Qualifications Framework. Available at http://www.ofqual.gov.uk/ (accessed 23 December 2011).
North K, Emmett P . Multivariate analysis of diet among three-year-old children and associations with socio-demographic characteristics. The Avon Longitudinal Study of Pregnancy and Childhood (ALSPAC) Study Team. Eur J Clin Nutr 2000; 54: 73–80.
O'Brien RMA . Caution regarding rules of thumb for variance inflation factors. Qual Quant 2006; 41: 673–690.
von Elm E, Altman DG, Egger M, Pocock SJ, Gotzsche PC, Vandenbroucke JP . The Strengthening the Reporting of Observational Studies in Epidemiology (STROBE) statement: guidelines for reporting observational studies. J Clin Epidemiol 2008; 61: 344–349.
Zaninotto P, Head J, Stamatakis E, Wardle H, Mindell J . Trends in obesity among adults in England from 1993 to 2004 by age and social class and projections of prevalence to 2012. J Epidemiol Community Health 2009; 63: 140–146.
Cole TJ, Freeman JV, Preece MA . Body mass index reference curves for the UK, 1990. Arch Dis Child 1995; 73: 25–29.
Thuny F, Richet H, Casalta J-P, Angelakis E, Habib G, Raoult D . Vancomycin treatment of infective endocarditis is linked with recently acquired obesity. PLoS ONE 2010; 5: e9074.
Cho I, Yamanishi S, Cox LM, Methe B, Zavadil J, Li K et al. Early-life antibiotics alter the murine colonic microbiome and adiposity. Nature (in press).
Mangrio E, Wremp A, Moghaddassi M, Merlo J, Bramhagen AC, Rosvall M . Antibiotic use among 8-month-old children in Malmo, Sweden--in relation to child characteristics and parental sociodemographic, psychosocial and lifestyle factors. BMC Pediatr 2009; 9: 31.
Thrane N, Olesen C, Schonheyder HC, Sorensen HT . Socioeconomic factors and prescription of antibiotics in 0- to 2-year-old Danish children. J Antimicrob Chemother 2003; 51: 683–689.
Billewicz WZ, Kemsley WF, Thomson AM . Indices of adiposity. Br J Prev Soc Med 1962; 16: 183–188.
Howe LD, Tilling K, Benfield L, Logue J, Sattar N, Ness AR et al. Changes in ponderal index and body mass index across childhood and their associations with fat mass and cardiovascular risk factors at age 15. PLoS One 2010; 5: e15186.
Gutin B, Litaker M, Islam S, Manos T, Smith C, Treiber F . Body-composition measurement in 9-11-y-old children by dual-energy X-ray absorptiometry, skinfold-thickness measurements, and bioimpedance analysis. Am J Clin Nutr 1996; 63: 287–292.
Toschke AM, Martin RM, von Kries R, Wells J, Smith GD, Ness AR . Infant feeding method and obesity: body mass index and dual-energy X-ray absorptiometry measurements at 9-10 y of age from the Avon Longitudinal Study of Parents and Children (ALSPAC). Am J Clin Nutr 2007; 85: 1578–1585.
Oken E, Gillman MW . Fetal origins of obesity. Obes Res 2003; 11: 496–506.
Schneider-Lindner V, Quach C, Hanley JA, Suissa S . Secular trends of antibacterial prescribing in UK paediatric primary care. J Antimicrob Chemother 2011; 66: 424–433.
Trasande L . How much should we invest in preventing childhood obesity? Health Aff 2010; 29: 372–378.
Acknowledgements
We are extremely grateful to all the families who took part in this study, the midwives for their help in recruiting them and the whole ALSPAC team, which includes interviewers, computer and laboratory technicians, clerical workers, research scientists, volunteers, managers, receptionists and nurses. The UK Medical Research Council, the Wellcome Trust (Grant ref: 092731) and the University of Bristol provide core support for ALSPAC. This publication is the work of the authors and Drs Trasande and Blustein will serve as guarantors for the contents of this paper and does not reflect the views of the ALSPAC executive. Support for our preliminary work with the ALPSAC database was provided through a pilot grant from the NYU Global Public Health Research Challenge Fund, and by NIH grants RO1GM090989 and 1UL1RR029893. We are grateful for support of Joseph Conigliaro, John Billings and Teresa Attina for their critical review of early results of our analysis.
Author information
Authors and Affiliations
Corresponding author
Ethics declarations
Competing interests
The authors declare no conflict of interest.
Additional information
Supplementary Information accompanies the paper on International Journal of Obesity website
Supplementary information
Rights and permissions
About this article
Cite this article
Trasande, L., Blustein, J., Liu, M. et al. Infant antibiotic exposures and early-life body mass. Int J Obes 37, 16–23 (2013). https://doi.org/10.1038/ijo.2012.132
Received:
Revised:
Accepted:
Published:
Issue Date:
DOI: https://doi.org/10.1038/ijo.2012.132
Keywords
This article is cited by
-
Clinical and economic impacts of a modified-observational screening approach to well-appearing infants born to mothers with chorioamnionitis
Journal of Perinatology (2023)
-
Mechanisms linking bariatric surgery to adipose tissue, glucose metabolism, fatty liver disease and gut microbiota
Langenbeck's Archives of Surgery (2023)
-
Early-life antibiotic exposure increases the risk of childhood overweight and obesity in relation to dysbiosis of gut microbiota: a birth cohort study
Annals of Clinical Microbiology and Antimicrobials (2022)
-
Small molecule modulation of microbiota: a systems pharmacology perspective
BMC Bioinformatics (2022)
-
Bone loss is ameliorated by fecal microbiota transplantation through SCFA/GPR41/ IGF1 pathway in sickle cell disease mice
Scientific Reports (2022)