Abstract
Background/Objectives:
Exacerbated postprandial lipid responses are associated with an increased cardiovascular risk. Dietary proteins influence postprandial lipemia differently, and whey protein has a preferential lipid-lowering effect. We compared the effects of different whey protein fractions on postprandial lipid and hormone responses added to a high-fat meal in type 2 diabetic subjects.
Subjects/Methods:
A total of 12 type 2 diabetic subjects ingested four isocaloric test meals in randomized order. The test meals contained 100 g of butter and 45 g of carbohydrate in combination with 45 g of whey isolate (iso-meal), whey hydrolysate (hydro-meal), α-lactalbumin enhanced whey (lac-meal) or caseinoglycomacropeptide enhanced whey (CGMP-meal). Plasma concentrations of triglyceride, retinyl palmitate, free fatty acid, insulin, glucose, glucagon, glucagon-like peptide 1 and glucose-dependent insulinotropic peptide were measured before and at regular intervals until 8-h postprandially.
Results:
We found no statistical significant differences between meals on our primary variable triglyceride. The retinyl palmitate response was higher after the hydro-meal than after the iso- and lac-meal in the chylomicron-rich fraction (P=0.008) while no significant differences were found in the chylomicron-poor fraction. The hydro- and iso-meal produced a higher insulin response compared with the lac- and CGMP-meal (P<0.001). Otherwise no significant differences in the hormone responses were found in the incremental area under the curve over the 480-min period.
Conclusions:
A supplement of four different whey protein fractions to a fat-rich meal had similar effects on postprandial triglyceride responses in type 2 diabetic subjects. Whey isolate and whey hydrolysate caused a higher insulin response.
This is a preview of subscription content, access via your institution
Access options
Subscribe to this journal
Receive 12 print issues and online access
$259.00 per year
only $21.58 per issue
Buy this article
- Purchase on Springer Link
- Instant access to full article PDF
Prices may be subject to local taxes which are calculated during checkout


Similar content being viewed by others
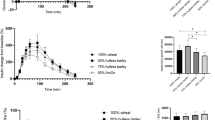
References
Turner RC, Millns H, Neil HA, Stratton IM, Manley SE, Matthews DR et al Risk factors for coronary artery disease in non-insulin dependent diabetes mellitus: United Kingdom Prospective Diabetes Study (UKPDS: 23). BMJ 1998; 316: 823–828.
Haffner SM, Lehto S, Ronnemaa T, Pyorala K, Laakso M . Mortality from coronary heart disease in subjects with type 2 diabetes and in nondiabetic subjects with and without prior myocardial infarction. N Engl J Med 1998; 339: 229–234.
Taskinen MR . Diabetic dyslipidaemia: from basic research to clinical practice. Diabetologia 2003; 46: 733–749.
Rivellese AA, De NC, Di ML, Patti L, Iovine C, Coppola S et al Exogenous and endogenous postprandial lipid abnormalities in type 2 diabetic patients with optimal blood glucose control and optimal fasting triglyceride levels. J Clin Endocrinol Metab 2004; 89: 2153–2159.
Karpe F, Steiner G, Uffelman K, Olivecrona T, Hamsten A . Postprandial lipoproteins and progression of coronary atherosclerosis. Atherosclerosis 1994; 106: 83–97.
Patsch JR, Miesenbock G, Hopferwieser T, Muhlberger V, Knapp E, Dunn JK et al Relation of triglyceride metabolism and coronary artery disease. Studies in the postprandial state. Arterioscler Thromb 1992; 12: 1336–1345.
Bansal S, Buring JE, Rifai N, Mora S, Sacks FM, Ridker PM . Fasting compared with nonfasting triglycerides and risk of cardiovascular events in women. JAMA 2007; 298: 309–316.
Nordestgaard BG, Benn M, Schnohr P, Tybjaerg-Hansen A . Nonfasting triglycerides and risk of myocardial infarction, ischemic heart disease, and death in men and women. JAMA 2007; 298: 299–308.
Dubois C, Beaumier G, Juhel C, Armand M, Portugal H, Pauli AM et al Effects of graded amounts (0–50 g) of dietary fat on postprandial lipemia and lipoproteins in normolipidemic adults. Am J Clin Nutr 1998; 67: 31–38.
Thomsen C, Rasmussen O, Lousen T, Holst JJ, Fenselau S, Schrezenmeir J et al Differential effects of saturated and monounsaturated fatty acids on postprandial lipemia and incretin responses in healthy subjects. Am J Clin Nutr 1999; 69: 1135–1143.
Thomsen C, Storm H, Holst JJ, Hermansen K . Differential effects of saturated and monounsaturated fats on postprandial lipemia and glucagon-like peptide 1 responses in patients with type 2 diabetes. Am J Clin Nutr 2003; 77: 605–611.
Lairon D, Play B, Jourdheuil-Rahmani D . Digestible and indigestible carbohydrates: interactions with postprandial lipid metabolism. J Nutr Biochem 2007; 18: 217–227.
Graf S, Egert S, Heer M . Effects of whey protein supplements on metabolism: evidence from human intervention studies. Curr Opin Clin Nutr Metab Care 2011; 14: 569–580.
Brader L, Holm L, Mortensen L, Thomsen C, Astrup A, Holst JJ et al Acute effects of casein on postprandial lipemia and incretin responses in type 2 diabetic subjects. Nutr Metab Cardiovasc Dis 2010; 20: 101–109.
Westphal S, Kastner S, Taneva E, Leodolter A, Dierkes J, Luley C . Postprandial lipid and carbohydrate responses after the ingestion of a casein-enriched mixed meal. Am J Clin Nutr 2004; 80: 284–290.
Mortensen LS, Hartvigsen ML, Brader LJ, Astrup A, Schrezenmeir J, Holst JJ et al Differential effects of protein quality on postprandial lipemia in response to a fat-rich meal in type 2 diabetes: comparison of whey, casein, gluten, and cod protein. Am J Clin Nutr 2009; 90: 41–48.
Pal S, Ellis V, Ho S . Acute effects of whey protein isolate on cardiovascular risk factors in overweight, post-menopausal women. Atherosclerosis 2010; 212: 339–344.
Nilsson M, Stenberg M, Frid AH, Holst JJ, Bjorck IM . Glycemia and insulinemia in healthy subjects after lactose-equivalent meals of milk and other food proteins: the role of plasma amino acids and incretins. Am J Clin Nutr 2004; 80: 1246–1253.
Power O, Hallihan A, Jakeman P . Human insulinotropic response to oral ingestion of native and hydrolysed whey protein. Amino Acids 2009; 37: 333–339.
Verges B . New insight into the pathophysiology of lipid abnormalities in type 2 diabetes. Diabetes Metab 2005; 31: 429–439.
Schwartz EA, Koska J, Mullin MP, Syoufi I, Schwenke DC, Reaven PD . Exenatide suppresses postprandial elevations in lipids and lipoproteins in individuals with impaired glucose tolerance and recent onset type 2 diabetes mellitus. Atherosclerosis 2010; 212: 217–222.
Krissansen GW . Emerging health properties of whey proteins and their clinical implications. J Am Coll Nutr 2007; 26: 713S–723S.
Blomhoff R, Green MH, Green JB, Berg T, Norum KR . Vitamin A metabolism: new perspectives on absorption, transport, and storage. Physiol Rev 1991; 71: 951–990.
Andersen L, Dinesen B, Jorgensen PN, Poulsen F, Roder ME . Enzyme immunoassay for intact human insulin in serum or plasma. Clin Chem 1993; 39: 578–582.
Krarup T, Madsbad S, Moody AJ, Regeur L, Faber OK, Holst JJ et al Diminished immunoreactive gastric inhibitory polypeptide response to a meal in newly diagnosed type I (insulin-dependent) diabetics. J Clin Endocrinol Metab 1983; 56: 1306–1312.
Orskov C, Rabenhoj L, Wettergren A, Kofod H, Holst JJ . Tissue and plasma concentrations of amidated and glycine-extended glucagon-like peptide I in humans. Diabetes 1994; 43: 535–539.
Holst JJ . Evidence that enteroglucagon (II) is identical with the C-terminal sequence (residues 33-69) of glicentin. Biochem J 1982; 207: 381–388.
Schrezenmeir J, Weber P, Probst R, Biesalski HK, Luley C, Prellwitz W et al Postprandial pattern of triglyceride-rich lipoprotein in normal-weight humans after an oral lipid load: exaggerated triglycerides and altered insulin response in some subjects. Ann Nutr Metab 1992; 36: 186–196.
Carstensen M, Thomsen C, Hermansen K . Incremental area under response curve more accurately describes the triglyceride response to an oral fat load in both healthy and type 2 diabetic subjects. Metabolism 2003; 52: 1034–1037.
Matthews JN, Altman DG, Campbell MJ, Royston P . Analysis of serial measurements in medical research. BMJ 1990; 300: 230–235.
Pal S, Ellis V . The chronic effects of whey proteins on blood pressure, vascular function, and inflammatory markers in overweight individuals. Obesity 2010; 18: 1354–1359.
Ballard KD, Bruno RS, Seip RL, Quann EE, Volk BM, Freidenreich DJ et al Acute ingestion of a novel whey-derived peptide improves vascular endothelial responses in healthy individuals: a randomized, placebo controlled trial. Nutr J 2009; 8: 34.
Karpe F, Bell M, Bjorkegren J, Hamsten A . Quantification of postprandial triglyceride-rich lipoproteins in healthy men by retinyl ester labeling and simultaneous measurement of apolipoproteins B-48 and B-100. Arterioscler Thromb Vasc Biol 1995; 15: 199–207.
Lithell H, Boberg J, Hellsing K, Lundqvist G, Vessby B . Lipoprotein-lipase activity in human skeletal muscle and adipose tissue in the fasting and the fed states. Atherosclerosis 1978; 30: 89–94.
Eckel RH . Lipoprotein lipase. A multifunctional enzyme relevant to common metabolic diseases. N Engl J Med 1989; 320: 1060–1068.
Manders RJ, Wagenmakers AJ, Koopman R, Zorenc AH, Menheere PP, Schaper NC et al Co-ingestion of a protein hydrolysate and amino acid mixture with carbohydrate improves plasma glucose disposal in patients with type 2 diabetes. Am J Clin Nutr 2005; 82: 76–83.
Holst JJ, Orskov C . Incretin hormones—an update. Scand J Clin Lab Invest Suppl 2001; 234: 75–85.
Calbet JA, Holst JJ . Gastric emptying, gastric secretion and enterogastrone response after administration of milk proteins or their peptide hydrolysates in humans. Eur J Nutr 2004; 43: 127–139.
Acknowledgements
We thank Tove Skrumsager and Lene Trudsø for excellent technical assistance. This work was carried out as a part of the research programme of the Danish Obesity Research Centre (DanORC, http://www.danorc.dk) and was supported by Nordic Centre of Excellence (NCoE) programme (Systems biology in controlled dietary interventions and cohort studies—SYSDIET, P no. 070014) and supported by a grant from Arla Foods Ingredients amba.
Author information
Authors and Affiliations
Corresponding author
Ethics declarations
Competing interests
Arne Astrup is a scientific member of Global Dairy Platform (Chicago) and receives speaker’s honoraria and research funding from the Danish Dairy Foundation, Arla and Danish Meat Association. Vivian K Jensen is employed by Arla Foods Ingredients amba. The rest of the authors declare no conflict of interest.
Rights and permissions
About this article
Cite this article
Mortensen, L., Holmer-Jensen, J., Hartvigsen, M. et al. Effects of different fractions of whey protein on postprandial lipid and hormone responses in type 2 diabetes. Eur J Clin Nutr 66, 799–805 (2012). https://doi.org/10.1038/ejcn.2012.48
Received:
Revised:
Accepted:
Published:
Issue Date:
DOI: https://doi.org/10.1038/ejcn.2012.48
Keywords
This article is cited by
-
A pre-meal of whey proteins induces differential effects on glucose and lipid metabolism in subjects with the metabolic syndrome: a randomised cross-over trial
European Journal of Nutrition (2019)
-
Whey protein lowers systolic blood pressure and Ca-caseinate reduces serum TAG after a high-fat meal in mildly hypertensive adults
Scientific Reports (2018)
-
Fortification of the whey protein isolate antioxidant and antidiabetic activity with fraction rich in phenolic compounds obtained from Stevia rebaudiana (Bert.). Bertoni leaves
Journal of Food Science and Technology (2017)
-
Emerging trends in nutraceutical applications of whey protein and its derivatives
Journal of Food Science and Technology (2015)
-
Vitamin D and L-cysteine levels correlate positively with GSH and negatively with insulin resistance levels in the blood of type 2 diabetic patients
European Journal of Clinical Nutrition (2014)