« Prev Next »
Have you ever looked into the night sky, and been lucky enough to spot another planet? Our solar system has 8 planets, many with their own moons and sometimes with battalions of accompanying asteroids. But how did they all get there, and are they different from our own home planet? What makes them different?
We can observe currently active processes in our solar system to unlock
the puzzles of how planets were formed. We know most about Earth and the
processes that shaped it, and we can apply this to studies of surface features
(such as glaciation, volcanism, river and lake features), rock composition,
atmospheres and magnetic fields of other planets to give us a better idea of their
origin, as well as possible future pathways for Earth and other planets.
Studies of these planets reveal to us hidden secrets, such as that Mercury is
similar to the Earth on the inside, Mars is like the Earth that didn't quite
make it, and Venus is a version of the Earth gone horribly wrong. So, let's
look at this comparative approach to planetary geoscience and see what we can
learn about our own home planet in the process.
First, let’s take a brief tour of the different types of planet we find
in our solar system:
Jovian planets
Jupiter-like, or Jovian planets (gas giants) were the first to form many
billions of years ago. Condensation of noble gases (unreactive ones that sit on
the far right of the periodic table of elements) and volatile (explosive)
elements such as hydrogen or helium came together beyond the 'snow line', a
point in our solar system where it is cold enough for these elements to form
solid ice grains. As they rapidly grew, gravity became strong enough to hold
the elements in place and form these enormous gas behemoths.
Rocky (terrestrial) planets
Successive collisions of rocky masses in space formed the rocky planets,
such as Earth. These collisions and sticking together of different objects
eventually differentiated into the layers we are familiar with on Earth due to
the different weights of the elements; iron- and nickel-rich matter sunk to the
centre of planets to form their cores, the lightest rocks formed the external crusts
of the planets and in between sits the mantle. The asteroid belt between Mars
and Jupiter may actually be an example of the failed formation of a terrestrial
planet!
Surface features
These structures are commonly found on planets include impact craters,
evidence of volcanism, tectonics and erosion.
All of these processes can also be seen on Earth, however evidence of
impact craters is rarer on Earth due to constant renewal of the surface of the
planet by plate tectonics and erosion by wind, ice, and water. Bombardment rate
(the amount of meteorites that hit planets in a given time) has also slowly
decreased, as most large meteorites have burned up in the atmospheres of
planets or already impacted in the almost 14 billion year history of our
universe.
Craters
Craters indicate impact of meteorites on the surfaces of planets, and are very useful as indicators of geological characteristics such as the extent of volcanism, erosion and tectonics.

On the moon,
the crater density – the number of craters in a specific area – varies: highlands
(on the moon, the ‘highlands’ are the areas near the north and south poles) have
remarkably high numbers of impact craters compared to the rest of the rocky
marble. Mercury has lots of craters, yet Venus has very few craters, due to its
dense atmosphere (containing lots of ‘heavy’ gases), meaning that many
meteorites burn up due to friction in the atmosphere and never complete their
mission to the planet’s surface. Jupiter’s moons mostly have high crater
densities because they have a strong gravitational pull, but Europa and Io have
relatively little due to their ice cover and volcanism, respectively.
Volcanoes
Volcanism
involves the melting of a planet’s interior and eruption of molten (liquid) rock
at the surface. Lots of heat is required to melt these rocks, which often can
have extremely high melting temperatures of up to 1200 degrees Celsius. This
heat comes from the initial heat of planetary formation, radioactive decay of
elements within the planet, or tidal friction – the gravitational pull that
occurs between planets and their moons. Typically, a planet increases in
temperature by about 25 degrees Celsius for each kilometre you descend below
the surface (called a ‘geothermal gradient’), so magmas form at many different
depths depending on what they’re made from, their chemical composition.
Across the surface of Mars, features termed
‘sinuous ridges’ can be found that are hundreds of kilometres in length and
hundreds of metres wide. These ridges can be thought of as volcanic river
‘trace fossils’. Imagine a fast flowing river in which lots of sediment is
deposited on the river bed, including large boulders. This is characteristic of
any river, from the Thames to the Yangtze! Eventually, the river dries up and
millions of years of erosion go by. Next to the river are soft sediments like
clays and muds, while inside the river are sands and boulders. The clays and
muds erode away faster than the bigger river deposits, so that the old river
bed is now a long hill, just like a country scale trace fossil! These same
ridge styles can also form from lava flows, and populate the surface of Mars!
Mars is also home to the largest volcano in the entire Solar System at 27,000m high – Olympus Mons.
Mars has not been visibly active in our lifetimes, although it is assumed that
there has been recent volcanic activity (geologically speaking!). The main form
of volcanism is basaltic, but more gas accumulates in the lava than expected due
to a lack of atmospheric pressure. This means that normally magmas that are runny
and passive on Earth can erupt violently on Mars. The lack of gravity also
means that magmas are less buoyant (do not float), so they stay in the mantle
for longer in large magma chambers. So, when the magma eventually gets hot
enough to escape, the eruptions last much longer with larger volumes of lava
(lava is magma that has breached a planetary surface). Therefore, with larger
eruptions, Mars can build larger volcanoes!
Venus has extensive evidence lava flows, with vast plains and channels from constant floods of basalt remoulding and resurfacing of the planet, like a really hot face mask that doesn’t so much leave the planet refreshed as excessively burnt. Io is the most volcanically active body in the solar system and has constant eruptions of toxic sulphur dioxide (SO2). These correspond with extremely hot localised surface areas and the plumes of erupting SO2 are so big they can even be seen from space.

Plate tectonics
Tectonics is
the process that involves the motions of the outside crustal layer of rocks on
a planet. Tectonics involves lithospheric (Earth’s crust and uppermost mantle)
deformation by internal (volcanic, radioactive) or external (meteorite impact)
forces and leads to distinctive landscape features such as faults, fractures
and folds in crustal and lithospheric rocks. In this, the Earth is probably unique,
as we have numerous tectonic plates of different sizes and compositions. These
interact along plate boundaries, of which there are three main types such as
collisional (where mountains form by plates pushing together), extensional
(where oceans form by plates tearing apart) and conservative (where plates
slide past one another) and are composed of the three main rock types: igneous,
sedimentary and metamorphic.
Other rocky planets
that do not have plate tectonics as we know it on Earth are smaller, therefore
they cooled quicker and formed a thicker crust. Mercury and Mars, because of
this, have faults in rocks – where rocks have moved in relation to one another
– but not plate tectonics. Faulting occurs here because there has to be
movement to accommodate the massive inter-planetary forces caused by
gravitational pull.
Venus is the
most similar to Earth regarding tectonics, but has a very different tectonic
style due to possible lack of water on the planet causing a change in the rheology
(elasticity) of the rocks to make them more likely to fault than develop
tectonics. More water in the chemical make-up of rocks means the rocks move
more easily in response to forces upon them, meaning they do not break so
easily, and they are more elastic. Mars has a single plate that is thick and
old but geologically diverse (containing lots of rock types).
Decoding the system
The story of the evolution of our solar system is scarred in the faces of the planets, and hidden in their unfathomable rocky depths. We’re just beginning to see what is out there, but we are pushing ever further. It’s only a matter of time until we know about the evolution of other planets, and can use this to decipher the geological past and the future of our own home planet, Earth.
Take a look at the NASA website, and the satellite images taken of various planets, including Earth. Can you see any surface structures of other planets such as Mars comparable to those on Earth? Do you think these structures were caused by the same thing? If not, why? What do you think is different about the structures’ formation? Leave your ideas in the comments below, and if you have questions then ask us in the comments or via email or Twitter (Jane, Jon).
By Jane and Jon
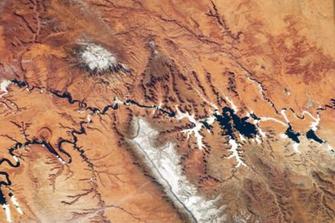