Abstract
Precise control of transcriptional programmes underlying metazoan development is modulated by enzymatically active co-regulatory complexes, coupled with epigenetic strategies. One thing that remains unclear is how specific members of histone modification enzyme families, such as histone methyltransferases and demethylases, are used in vivo to simultaneously orchestrate distinct developmental gene activation and repression programmes. Here, we report that the histone lysine demethylase, LSD1—a component of the CoREST-CtBP co-repressor complex—is required for late cell-lineage determination and differentiation during pituitary organogenesis. LSD1 seems to act primarily on target gene activation programmes, as well as in gene repression programmes, on the basis of recruitment of distinct LSD1-containing co-activator or co-repressor complexes. LSD1-dependent gene repression programmes can be extended late in development with the induced expression of ZEB1, a Krüppel-like repressor that can act as a molecular beacon for recruitment of the LSD1-containing CoREST-CtBP co-repressor complex, causing repression of an additional cohort of genes, such as Gh, which previously required LSD1 for activation. These findings suggest that temporal patterns of expression of specific components of LSD1 complexes modulate gene regulatory programmes in many mammalian organs.
This is a preview of subscription content, access via your institution
Access options
Subscribe to this journal
Receive 51 print issues and online access
$199.00 per year
only $3.90 per issue
Buy this article
- Purchase on Springer Link
- Instant access to full article PDF
Prices may be subject to local taxes which are calculated during checkout





Similar content being viewed by others
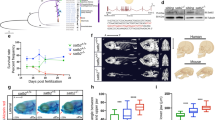
References
Jaenisch, R. & Bird, A. Epigenetic regulation of gene expression, how the genome integrates intrinsic and environmental signals. Nature Genet. 33, (Suppl.). 245–254 (2003)
Fischle, W., Wang, Y. & Allis, C. D. Binary switches and modification cassettes in histone biology and beyond. Nature 425, 475–479 (2003)
Martin, C. & Zhang, Y. The diverse functions of histone lysine methylation. Nature Rev. Mol. Cell Biol. 6, 838–849 (2005)
Chong, J. A. et al. REST, a mammalian silencer protein that restricts sodium channel gene expression to neurons. Cell 80, 949–957 (1995)
Schoenherr, C. J. & Anderson, D. J. The neuron-restrictive silencer factor (NRSF), a coordinate repressor of multiple neuron-specific genes. Science 267, 1360–1363 (1995)
Andres, M. E. et al. CoREST, a functional corepressor required for regulation of neural-specific gene expression. Proc. Natl Acad. Sci. USA 96, 9873–9878 (1999)
Ballas, N. et al. Regulation of neuronal traits by a novel transcriptional complex. Neuron 31, 353–365 (2001)
Lunyak, V. V. et al. Corepressor-dependent silencing of chromosomal regions encoding neuronal genes. Science 298, 1747–1752 (2002)
Shi, Y. et al. Coordinated histone modifications mediated by a CtBP co-repressor complex. Nature 422, 735–738 (2003)
You, A., Tong, J. K., Grozinger, C. M. & Schreiber, S. L. CoREST is an integral component of the CoREST–human histone deacetylase complex. Proc. Natl Acad. Sci. USA 98, 1454–1458 (2001)
Humphrey, G. W. et al. Stable histone deacetylase complexes distinguished by the presence of SANT domain proteins CoREST/kiaa0071 and Mta-L1. J. Biol. Chem. 276, 6817–6824 (2001)
Nakamura, T. et al. ALL-1 is a histone methyltransferase that assembles a supercomplex of proteins involved in transcriptional regulation. Mol. Cell 10, 1119–1128 (2002)
Hakimi, M. A. et al. A core-BRAF35 complex containing histone deacetylase mediates repression of neuronal-specific genes. Proc. Natl Acad. Sci. USA 99, 7420–7425 (2002)
Hakimi, M. A. et al. A candidate X-linked mental retardation gene is a component of a new family of histone deacetylase-containing complexes. J. Biol. Chem. 278, 7234–7239 (2003)
Shi, Y. et al. Histone demethylation mediated by the nuclear amine oxidase homolog LSD1. Cell 119, 941–953 (2004)
Metzger, E. et al. LSD1 demethylates repressive histone marks to promote androgen-receptor-dependent transcription. Nature 437, 436–439 (2005)
Tsukada, Y. et al. Histone demethylation by a family of JmjC domain-containing proteins. Nature 439, 811–816 (2005)
Yamane, K. et al. JHDM2A, a JmjC-containing H3K9 demethylase, facilitates transcription activation by androgen receptor. Cell 125, 483–495 (2006)
Whetstine, J. R. et al. Reversal of histone lysine trimethylation by the JMJD2 family of histone demethylases. Cell 125, 467–481 (2006)
Keegan, C. E. & Camper, S. A. Mouse knockout solves endocrine puzzle and promotes new pituitary lineage model. Genes Dev. 17, 677–682 (2003)
Scully, K. M. & Rosenfeld, M. G. Pituitary development, regulatory codes in mammalian organogenesis. Science 295, 2231–2235 (2002)
Olson, L. E. et al. A homeodomain-mediated mechanism for β-catenin-dependent switching events dictates cell lineage determination. Cell 125, 593–605 (2006)
Mayo, K. E. Molecular cloning and expression of a pituitary-specific receptor for growth hormone-releasing hormone. Mol. Endocrinol. 6, 1734–1744 (1992)
Lin, C., Lin, S. C., Chang, C. P. & Rosenfeld, M. G. Pit-1-dependent expression of the receptor for growth hormone releasing factor mediates pituitary cell growth. Nature 360, 765–768 (1992)
Godfrey, P. et al. GHRH receptor of little mice contains a missense mutation in the extracellular domain that disrupts receptor function. Nature Genet. 4, 227–232 (1993)
Maier, M. M. & Gessler, M. Comparative analysis of the human and mouse Hey1 promoter: Hey genes are new Notch target genes. Biochem. Biophys. Res. Commun. 275, 652–660 (2000)
Oswald, F. et al. RBP-Jκ/SHARP recruits CtIP/CtBP corepressors to silence Notch target genes. Mol. Cell. Biol. 25, 10379–10390 (2005)
Zhu, X. et al. Sustained Notch signaling in progenitors is required for sequential emergence of distinct cell lineages during organogenesis. Genes Dev. 20, 2739–2753 (2006)
Sornson, M. W. et al. Pituitary lineage determination by the Prophet of Pit-1 homeodomain factor defective in Ames dwarfism. Nature 384, 327–333 (1996)
Ward, R. D. et al. Role of PROP1 in pituitary gland growth. Mol. Endocrinol. 19, 698–710 (2005)
Gage, P. J., Roller, M. L., Saunders, T. L., Scarlett, L. M. & Camper, S. A. Anterior pituitary cells defective in the cell-autonomous factor, df, undergo cell lineage specification but not expansion. Development 122, 151–160 (1996)
Botz, J. et al. Cell cycle regulation of the murine cyclin E gene depends on an E2F binding site in the promoter. Mol. Cell. Biol. 16, 3401–3409 (1996)
Hu, N. et al. Heterozygous Rb-1 delta 20/+ mice are predisposed to tumors of the pituitary gland with a nearly complete penetrance. Oncogene 9, 1021–1027 (1994)
Lasorella, A., Rothschild, G., Yokota, Y., Russell, R. G. & Iavarone, A. Id2 mediates tumor initiation, proliferation, and angiogenesis in Rb mutant mice. Mol. Cell. Biol. 25, 3563–3574 (2005)
Behringer, R. R., Mathews, L. S., Palmiter, R. D. & Brinster, R. L. Dwarf mice produced by genetic ablation of growth hormone-expressing cells. Genes Dev. 2, 453–461 (1988)
Borrelli, E., Heyman, R. A., Arias, C., Sawchenko, P. E. & Evans, R. M. Transgenic mice with inducible dwarfism. Nature 339, 538–541 (1989)
Scully, K. M. et al. Allosteric effects of Pit-1 DNA sites on long-term repression in cell type specification. Science 290, 1127–1131 (2000)
Williams, T. M. et al. Identification of a zinc finger protein that inhibits IL-2 gene expression. Science 254, 1791–1794 (1991)
Postigo, A. A. & Dean, D. C. ZEB represses transcription through interaction with the corepressor CtBP. Proc. Natl Acad. Sci. USA 96, 6683–6688 (1999)
Fernandes, I. et al. Ligand-dependent nuclear receptor corepressor LCoR functions by histone deacetylase-dependent and -independent mechanisms. Mol. Cell 11, 139–150 (2003)
Perissi, V. & Rosenfeld, M. G. Controlling nuclear receptors, the circular logic of cofactor cycles. Nature Rev. Mol. Cell Biol. 6, 542–554 (2005)
Satijn, D. P. et al. Interference with the expression of a novel human polycomb protein, hPc2, results in cellular transformation and apoptosis. Mol. Cell. Biol. 17, 6076–6086 (1997)
Kagey, M. H., Melhuish, T. A. & Wotton, D. The polycomb protein Pc2 is a SUMO E3. Cell 113, 127–137 (2003)
Wysocka, J. et al. WDR5 associates with histone H3 methylated at K4 and is essential for H3 K4 methylation and vertebrate development. Cell 121, 859–872 (2005)
Ooi, G. T., Tawadros, N. & Escalona, R. M. Pituitary cell lines and their endocrine applications. Mol. Cell. Endocrinol. 228, 1–21 (2004)
Korach, K. S. Insights from the study of animals lacking functional estrogen receptor. Science 266, 1524–1527 (1994)
Chamberlain, E. M. & Sanders, M. M. Identification of the novel player δEF1 in estrogen transcriptional cascades. Mol. Cell. Biol. 19, 3600–3606 (1999)
Lieberman, M. E., Slabaugh, M. B., Rutledge, J. J. & Gorski, J. The role of estrogen in the differentiation of prolactin producing cells. J. Steroid Biochem. 19, 275–281 (1983)
Day, R. N., Koike, S., Sakai, M., Muramatsu, M. & Maurer, R. A. Both Pit-1 and the estrogen receptor are required for estrogen responsiveness of the rat prolactin gene. Mol. Endocrinol. 4, 1964–1971 (1990)
Long, J., Zuo, D. & Park, M. Pc2-mediated sumoylation of Smad-interacting protein 1 attenuates transcriptional repression of E-cadherin. J. Biol. Chem. 280, 35477–35489 (2005)
Acknowledgements
We thank C. Nelson and L.Wang for technical assistance, L. Olson for generating Pitx1 Cre+ mice, Y. Geng for the Ccne1 promoter reporter, W. Herr for the generous gift of anti-WDR5 antibody, A. Gonzalez, J. Liu for advice on reagents, and J. Hightower and M. Fisher for figure and manuscript preparation. M.G.R is an investigator with HHMI. This work was supported by grants from the NIH and NCI to M.G.R., C.K.G. and X.-D.F.
Author information
Authors and Affiliations
Corresponding author
Ethics declarations
Competing interests
Reprints and permissions information is available at www.nature.com/reprints. The authors declare no competing financial interests.
Supplementary information
Supplementary Information
This file contains Supplementary Figures 1-8 with Legends and Supplementary Methods. (PDF 8391 kb)
Rights and permissions
About this article
Cite this article
Wang, J., Scully, K., Zhu, X. et al. Opposing LSD1 complexes function in developmental gene activation and repression programmes. Nature 446, 882–887 (2007). https://doi.org/10.1038/nature05671
Received:
Accepted:
Published:
Issue Date:
DOI: https://doi.org/10.1038/nature05671
This article is cited by
-
Kdm1a safeguards the topological boundaries of PRC2-repressed genes and prevents aging-related euchromatinization in neurons
Nature Communications (2024)
-
iGEM as a human iPS cell-based global epigenetic modulation detection assay provides throughput characterization of chemicals affecting DNA methylation
Scientific Reports (2023)
-
Epigenetic regulation of hybrid epithelial-mesenchymal cell states in cancer
Oncogene (2023)
-
Inhibition of LSD1 induces ferroptosis through the ATF4-xCT pathway and shows enhanced anti-tumor effects with ferroptosis inducers in NSCLC
Cell Death & Disease (2023)
-
Role of Interleukins in Inflammation-Mediated Tumor Immune Microenvironment Modulation in Colorectal Cancer Pathogenesis
Digestive Diseases and Sciences (2023)
Comments
By submitting a comment you agree to abide by our Terms and Community Guidelines. If you find something abusive or that does not comply with our terms or guidelines please flag it as inappropriate.