[Nature India Special Issue: Lighting the way in physics]
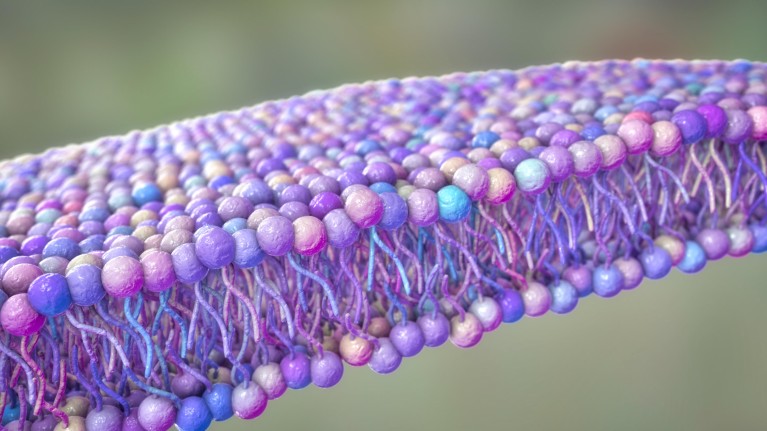
The phospholipid bilayer that forms the membrane around all living cells. Credit: Kateryna Kon/SPL/Getty Images
The cell membrane is the site of many vital processes and an important subject of research in cell biology. It also poses many interesting questions to physicists broadly concerning shape, organisation and interactions between its constituents.
The highly influential Fluid Mosaic model of the cell membrane, by S. J. Singer and G.L. Nicolson (Science, 1972) proposed that the cell membrane is a lipid bilayer, made up of mainly phospholipids and cholesterol, forming a 2D homogeneous fluid in which integral proteins and carbohydrates are embedded. Within the membrane, molecules were assumed to diffuse around thermally, under the influence of short-range intermolecular forces. This broad picture continues to be the basis of understanding several aspects of membrane behaviour. Subsequently , K. Simmons and E. Ikonen (Nature, 1997), proposed a variant of the above known as the Raft model, where the cell membrane forms lateral functional domains enriched in long-chain saturated fatty acids and cholesterol. These models are fundamentally based on equilibrium thermodynamics.
In our study and in earlier ones by our group, we demonstrated that these equilibrium models were insufficient to understand the spatiotemporal organisation of a large class of lipid-tethered proteins on the cell membrane, e.g. glycosylphosphatidylinositol-anchored proteins (GPI-APs), an upper leaflet membrane protein, known to associate with sphingolipids and cholesterol. The model we proposed was a radical departure from the earlier equilibrium ones, and viewed the cell membrane as being driven by active, out-of-equilibrium forces arising from its interaction with the adjoining cortical actin and myosin (Gowrishankar, K. Cell 149, 1353–1367, 2012), in addition to the usual thermodynamic forces.
This Active Composite model was a result of two independent streams of investigation. One was our long standing collaboration with Satyajit Mayor’s group at the National Centre for Biological Sciences (NCBS), who were using novel fluorescence-based assays to understand the local organisation of endocytosis of GPI-APs. The second was our theoretical investigations in the development of Active Soft Matter and Active Membranes.
Our first contribution, following a rather detailed analysis of the fluorescence data of GPI-APs, was to show that the GPI-APs, while associating with sphingolipids and cholesterol, were organised in a mixture of monomers and tight nanoclusters, containing around 4-5 molecules. This was fundamentally different from the perception of `Rafts’ that was popular amongst scientists at that time. In addition, we found that the fraction of GPI-APs in nanoclusters was independent of the total number, thus apparently violating equilibrium mass-action. These findings appeared in Cell, 2004, and marked the beginning of a fruitful collaboration between the experimental cell biology group at NCBS and the theoretical soft matter group at RRI.
Next, by deploying novel fluorescence anisotropy techniques and analyses methods, we studied the kinetics of aggregation and fragmentation of these nanoclusters and their spatial distribution. The nanoclusters appeared to show strong correlations with regions of contractile actin. Analysis of the kinetics suggested that the nanoclusters showed high and constant rates of aggregation and fragmentation at temperatures above 20°C, which reduced dramatically below this temperature. Further, the rates were sensitive to actin perturbations, strongly suggesting that the upper leaflet protein was sensing the dynamics of the underlying actin cortex. This study appeared in Cell, 2008.
By 2006, our independent work on active systems was already preparing us to think in terms of an active cell membrane driven by actomyosin stresses. We therefore started building up the elements of such a theoretical model, together with coming up with predictions that could be experimentally tested (or falsified!).
In our model (Cell, 2012), we viewed the cell surface as an Active Composite of a multi-component, asymmetric cell membrane juxtaposed with a thin actomyosin cortex. The actomyosin cortex just adjacent to the cell membrane comprises of short actin filaments (“formin”-nucleated) and Myosin minifilaments, that bind to and drive the actin filaments with the help of adenosine triphosphate (ATP), resulting in active currents and stresses at the cell membrane. These could be studied using a coarse grained hydrodynamic framework in terms of the concentration and orientation of actomyosin. The resulting patterns at steady state showed a variety of defect structures, including actin asters. By coupling the dynamics of actomyosin to membrane components such as GPI-APs, we showed that the formation of actin asters in the cortex could drive their nano-clustering.
This clustering is a uniquely active phenomenon and occurs in the absence of any attractive interactions between the clustered components. Introducing an active noise in the hydrodynamic equations lead to a constant fragmentation and aggregation of asters and hence of nanoclusters. The theory made several predictions on the kinetics of fragmentation-aggregation of nanoclusters, and the statistics of density fluctuations, including their extreme statistics, all of which were verified in experiments presented in the same paper.
This picture of active clustering of membrane molecules has subsequently been verified in in-vitro reconstitution experiments carried out in our group (PNAS, 2016). Our study, while putting forth two new ideas, namely the involvement of actin and myosin in the organisation of cell surface molecules, and the importance of nonequilibrium (active) forces in driving and maintaining that organisation at nanoscales, also opened the door to many outstanding questions, which we and others have pursued over the years.
The current picture of the Cell surface that emerges from these studies is the following : the cell surface is a nonequilibrium, active system built from a composite of an asymmetric multicomponent membrane and multicomponent actomyosin cortex. The cortex applies active stresses and currents on the inner leaflet of the cell membrane. Specific molecules on the inner leaflet of the cell membrane, such as Phosphatidyl Serine (PS), respond to these active stresses and transmit them to long-chain saturated lipids, such as GPI-APs, on the outer leaflet via transmembrane interactions that involves cholesterol and sphingolipids. These active stresses drive molecular clustering (proteins and lipids) at both nano and meso scales, giving rise to an Active Emulsion. This continues to be an active field of research.