[Nature India Special Issue: Lighting the way in physics]
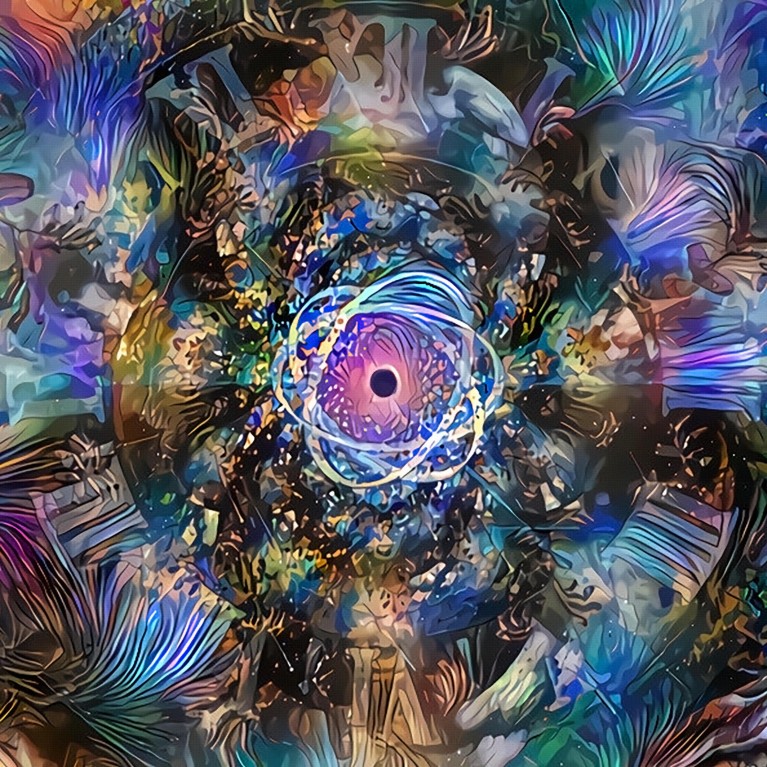
Forces originating from the geometry of quantum mechanics may be useful to make smaller atom traps and better atomic clocks. Credit: Bruce Rolff / Alamy Stock Photo
During a meeting of the Raman Institute Journal Club in the 1980s, my colleague Chandrakant Shukre and I were reviewing papers on quantum physics. Michael Berry from the University of Bristol had discovered a delicate geometrical effect appearing in quantum systems in a slowly changing environment1. The crystallographer Sivaraj Ramaseshan was in the audience and felt a sense of déjà vu. Further investigation revealed that Shivaramakrishnan Pancharatnam’s work on polarised light2 had anticipated Berry’s findings by three decades3.
Quantum mechanics deals with matter waves, which share some features with waves you may see in a pond or hear in a concert hall. When waves meet, they can reinforce each other or cancel each other out. The determining factor is the phase of the waves and whether the two waves are in step or out of step. Berry found that in a cyclic change of the environment there was an extra geometric contribution to the phase. This effect can be detected in interference experiments. The Berry phase has since proved a seminal idea in optics, mechanics and condensed matter physics.
When I was invited to speak about the Berry phase at a meeting on the foundations of quantum mechanics at the Centre for Theoretical Studies, Bangalore, I presented Pancharatnam’s work in the language of differential geometry. I had attended lectures on differential geometry by the distinguished Indian mathematicians Mudumbai Narasimhan and Sundararaman Ramanan who spoke about the theory of fibre bundles. I learnt the geometric interpretation of a connection, which is well known in mathematical circles.
A fibre bundle is a space which is locally a product of two spaces, but it is globally twisted. The simplest example is a Mobius strip. The centre line of the Mobius strip is a circle called the base space and the line perpendicular to it is called the ‘fibre’. The fibre twists around and reverses itself as it goes around the base circle. A ‘connection’ is a rule for comparing points on two fibres. This mathematics applies to quantum physics and polarised optics: the fibres carry the phase information and removing the phase information gives us the base, which is known in physics as the ray space.
During the discussion after my talk, I realised that not everyone in the physics community appreciated the geometric nature of connections. Most physicists think of them as ungainly objects transforming awkwardly under gauge transformations. There was a clear need for a differential geometric treatment of the Berry phase informed by Pancharatnam’s work on the interference of polarised light.
There was also a need for an experimental demonstration of Pancharatnam’s ideas on the interference of polarised light. Pancharatnam had noticed the fine interplay between the connection and the geodesics – the shortest paths – in the base. I suggested to my colleague Rajendra Bhandari that a laser beam passed through optical elements like polaroids to make a geodesic triangle, would reveal the Pancharatnam phase in interference experiments. Bhandari, who had made precision laser measurements on the surface accuracy of a radio telescope, had the required experimental skills to take up the challenge. This resulted in a paper that we submitted to Physical Review Letters (PRL)4. It was well received and was published in 1988.
The theoretical paper on the same topic that we submitted to PRL did not sail as smoothly5. There was opposition from within as well as without. As I was preparing the paper, there was a vocal minority of my colleagues demanding to know what was ‘new’ in the paper. One said it was full of ‘mathematical jargon’ and contained nothing of substance. One of the referees was scathing and dismissive and the paper was deemed unsuitable for publication. I replied with a point-by-point rebuttal. One of the problems was that the referee was unfamiliar with Pancharatnam’s work. After some verbal fencing, the paper was accepted, published and began to have an impact. It was especially well received in Italy where there is a strong tradition in differential geometry. The paper has been reprinted in a curated collection – Geometric Phases in Physics – translated into Italian and over the years it has floated serenely into the good graces of the wider scientific community – so much so that it is sometimes cited even when it need not be. This success stimulated several research groups in India to start working on the geometric phase.
Looking back, the work brings out a continuity in scientific research between the Raman era and the subsequent phase of the Raman Research Institute. Pancharatnam worked with Chandrasekhara Venkata Raman in the 1950s, and his ideas were rooted in experiments rather than fibre bundles. His thinking was both extremely precise and general. It was mathematics expressed in chiselled prose. Such clarity often leads to general perspectives far beyond the original context. The theoretical paper draws on this tradition and combines it with the modern differential geometric tradition of the Tata Institute of Fundamental Research where Narasimhan and Ramanan studied. Both traditions are firmly rooted in Indian soil. In fact, the modern differential geometric approach enables one to go beyond Pancharatnam's original ideas and offers a much more general and larger perspective.
In my work, I try to maintain a harmonious balance of abstract geometric ideas with concrete experiments. The elegance of mathematical abstraction combined with experimental demonstration weaves rigour and relevance firmly together. The same mathematics describe the geometry of quantum mechanics and purely classical problems. It can be used to describe the arcane art of parallel parking and the amazing grace and ability of falling cats to right themselves.
More recently in an investigation into the theory of closure invariants in radio astronomy6, we suggest the use of the geometry of special relativity to glean uncorrupted information from noisy data to enable polarised imaging of black holes in the event horizon telescope. I have also suggested that the forces originating from the geometry of quantum mechanics may be useful to make smaller atom traps and better atomic clocks7.