[Nature India Special Issue: Lighting the way in physics]
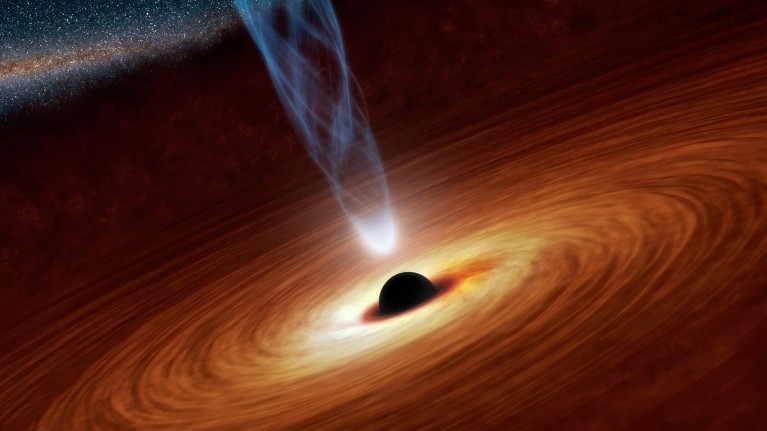
An impression of a disc formed as the dust and gas in the galaxy falls on to a supermassive black hole, attracted by its gravity. Credit: NASA/JPL-Caltech
Albert Einstein arrived at the famous nonlinear relativistic general relativity (GR) theory in November 1915. One year later, working in linear approximation, he proposed the existence of gravitational waves (GW) as one of the theory’s important consequences. In 1918, he calculated – to leading order – the emission of GWs and the corresponding flux of energy far from source in his well-known quadrupole formula for gravitational radiation1.
Computing GW emission beyond the leading order involves dealing with non-linearities of Einstein’s equations and including effects of higher-order velocity. The accuracy of analytical GW emission calculations beyond the leading-order quadrupole formula are labelled by the ‘post-Newtonian’ (PN) order v2/c2 ~ GM/c2r.
Russell Hulse and Joseph Taylor discovered the binary pulsar2 1913+16 in 1974. Discovery of binary systems with strong self-gravity mandated improved approaches to the ‘two-body problem’ requiring theorists to go beyond 1PN or v2/c2 relativistic effects in the equation of motion to 2.5PN or (v5/c5) due to the radiation reaction. High-quality binary pulsar data at that time forced scientists to revisit the approximation methods in GR3 to fix the mathematical shortcomings in the existing approaches4.
In the approach under consideration, the two compact objects in the binary are modelled as point particles since it can be argued that the tidal deformations are of higher order (5PN). The use of delta functions to model point particles in a non-linear theory leads to ultraviolet divergences and specific methods are needed for self-field regularisation. The discovery of 1913+16 and the paradigm shift from narrow band bar detectors to broadband laser interferometric GW detectors also changed the sources from supernovas to coalescing compact binaries (CCB) of neutron stars and black holes.
However, a detailed understanding of detector noise in the relevant frequency band of Hz-kHz indicates that GW from these CCB correspond to the situation of a weak signal buried in strong detector noise. Consequently, one requires techniques such as matched filtering to extract the signal from the noisy data and subsequently determine its parameters. For matched filtering to be efficient, accurate knowledge of the phase of the signal is needed, which as the system becomes more relativistic requires higher order corrections in velocity and hence higher orders in non-linearity GW.
Following funding of the Laser Interferometer Gravitational-Wave Observatory (LIGO) in the United States, work on constructing templates for GW detection were intensely investigated by Kip Thorne’s group at the California Institute of Technology and summarised in an article evocatively titled The last three minutes: issues in gravitational wave measurements of coalescing compact binaries5.
Thorne convened an international meeting in 1994 to brainstorm this issue and highlight the need to address this problem. Luc Blanchet and I were participants at this meeting as was Sanjeev Dhurandhar. Blanchet soon demonstrated the 2PN generation of GW and this led to a collaboration with him, Thibault Damour and me to compute the GW flux to order (v/c)4 beyond the Einstein formula and provide the calculation of 2PN phasing for inspiralling compact binaries6.
The new insight it required was the careful treatment of the cubic non-linearities in Einstein’s equations. The availability of the 2PN equation of motion from the binary pulsar work facilitated the computation of 2PN phasing of CCB by two independent methods7: the multipolar post-Minkowskian and post-Newtonian (MPM-PN) method used above and the direct integration of relaxed Einstein equations (DIRE) by Clifford Will and Alan Wiseman8 in the United States. A global virtual outpost for GW computations critical for the unforgiving GW experiments was coming to life. Data analysis pipelines for the initial detectors could be put in place based on these 2PN results.
The rather quick extension to 2PN phasing mistakenly led one to believe the extension to 3PN, required to deal with the more relativistic binary black hole systems, would be straightforward. Control of this 3PN order turned out to be more formidable due to the unexpected limitation of earlier regularisation methods for the self-field. Only after almost a decade of struggle and by complementing computations using Hadamard regularisation by the use of the gauge invariant dimensional regularisation9 was the problem finally resolved and completed10.
These results are the starting inputs for validating the early phases of the numerical relativity waveforms of CCB and the construction of extensions using the effective one-body and phenomenological approaches to describe merger and ringdown. Thus they play a critical role in the construction of templates for detection and parameter estimation and the basis for tests of gravity (TOG) using GW observations. The MPM-PN work is referred to in Kip Thorne’s Nobel lecture. The PN and TOG publications are quoted in the GW discovery papers11 and were the foundation of the current Indian presence in TOG activity in the LIGO-Virgo collaboration.
Currently MPM-PN is the most successful, since it can deal with all aspects of the required computations: the conservative equation of motion, radiation field at infinity, non-linear effects related to tails, tails of tails and non-linear memory. It has evolved over the last 30 years into a consistent algorithmic approach to analytical GW computations. MPM-PN and numerical relativity are the scaffolds underlying the impressive arch of effective one-body and Phenom template families used for detection, parameter estimation and TOG. The 3.5PN results have been the workhorse for the community until now since the tour de force of 4.5PN phasing was completed only recently12. At 4PN order a new feature arises: infrared regularisation plays a crucial role in addition to self-field regularisation. The 3.5PN phasing, 3PN modes13 for quasi-circular motion were followed by extensions to 3PN evolution14 and modes15 for quasi-elliptical motion.
On the Indian front, this work on source modelling at the Raman Research Institute was complemented by significant contributions to GW data analysis in a group led by Dhurandhar at the Inter-University Centre for Astronomy and Astrophysics. The aspiration to extend this Indian footprint in GW source modelling and data analysis to GW experiments motivated the formation of the Indian Initiative in Gravitational-wave Observations (IndIGO) consortium in 2009. This led to the LIGO-India proposal in 2011, IndIGO presence in the GW discovery and in-principle approval of LIGO-India in 2016 and finally the LIGO-India approval in 2023.