[Nature India Special Issue: Lighting the way in physics]
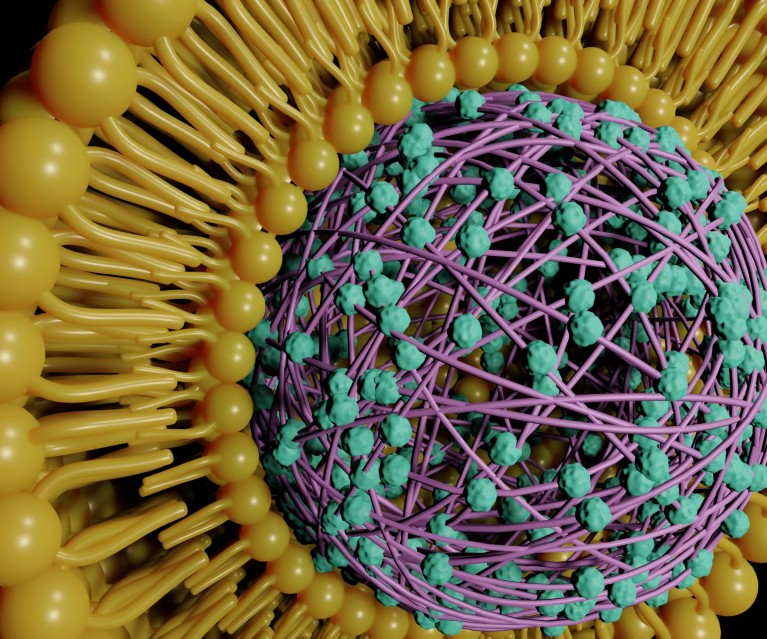
Lipid-coated polymeric nanoparticles for drug delivery. Credit: iStock / Getty Images Plus
Soft materials are constituted by weakly interacting macromolecules that are easily deformed by thermal stresses at room temperature. Some examples of common soft materials include: colloidal suspensions, such as ink and paint; detergents; emulsions, such as mayonnaise and butter; gels; foams; and pastes.
Even though these materials appear to be disparate, Pierre-Gilles de Gennes, the winner of the Nobel Prize for physics in 1991, pointed out that all soft materials possess structural complexity and mechanical flexibility. These features result in intriguing behaviours such as spontaneous self-assembly of macromolecules into fragile structures and thinning and thickening of viscosity under shear.
Colloidal suspensions comprise microscopic particles that stay uniformly distributed in a continuous medium due to their incessant temperature-induced jiggling (known as Brownian motion). Granular suspensions, in comparison, are constituted of larger ‘non-Brownian’ particles.
Since 2005, researchers at the Rheology and Light Scattering (RheoDLS) lab at the Raman Research Institute have studied the following aspects of soft materials through experimental techniques: modelling suspensions as scaled-up versions of atomic matter; setting up laboratory experiments to mimic geophysical phenomena such as landslides and river delta formation; and implementing table-top experiments to study non-equilibrium flows and pattern formation. Research emerging from the lab can be used in therapeutic, industrial and personal care applications.
The science of soft materials is strongly rooted in interdisciplinary ideas and employs concepts of physics, chemistry and biology to design and understand complex materials. Soft materials such as liposomes and polymer-based micelles have been shown to be efficient vehicles for the transport of therapeutic agents such as drugs and genes. Drugs packaged within the cores of nano-sized ‘soft’ micellar capsules can even be delivered to specific targets (E.V. Batrakova and A.V. Kabanov, J. Control. Release 130, 98; 2008).
Rajib Basak, a former member of the RheoDLS lab, and Ranjini Bandyopadhyay, principal investigator, explored the use of self-assembled micellar systems of colloidal sizes as biocompatible nanocarriers for drug molecules in a study published in 2013 (Langmuir 29, 13, 4350–4356; 2013). They encapsulated painkilling and antibiotic drugs — aspirin, ibuprofen and erythromycin — in spherical micelles formed by triblock copolymer Pluronic macromolecules at room temperature.
Pluronic is amphiphilic — this means that each Pluronic macromolecule possesses water-loving (hydrophilic) and water-hating (hydrophobic) portions. While the two-sided blocks of polyethylene oxide (PEO) are hydrophilic, the polypropylene oxide (PPO) block in the middle remains hydrophobic over a large temperature range. At high enough temperatures, the central PPO block becomes very hydrophobic and the PEO chains dehydrate significantly. This drives the association of Pluronic macromolecules into spherical micelles with hydrophobic PPO cores and hydrophilic PEO coronas. Since drug molecules are hydrophobic, they solubilise easily within the micellar cores. Basak and Bandyopadhyay’s research systematically assessed the properties of the individual drug-encapsulated micellar nanocarriers and demonstrated that environmental temperature and solution pH serve as efficient knobs to control the uptake of drugs in Pluronic micelles and their subsequent release and delivery.
Electron micrographs of individual Pluronic micelles showed globular shapes and sizes of 60–70 nm. The micelles remained globular though their sizes increased when drug molecules were incorporated in the micellar cores. Dynamic light scattering experiments verified these results and revealed a decrease in the critical micellisation temperature (CMT) in the presence of drugs. Below the CMT, the micellar nanocarriers decompose into individual amphiphilic molecules and deliver the encapsulated drugs. Fluorescence measurements with aromatic pyrene probe molecules pointed to enhanced water penetration in the micellar cores with decreasing temperature. This work suggested two strategies for releasing drugs inside micellar nanocarriers – by decreasing the temperature below 15oC or by increasing the pH of the solution medium. Cooling the target site or releasing an agent to marginally increase the pH at the target can therefore trigger drug delivery.
Incorporating drugs in the hydrophobic cores of Pluronic micelles enhances the former’s solubility and circulation times. The nanocarriers synthesised in the Basak and Bandyopadhyay study can be sterilised using standard filtration methods and delivered into the bloodstream using intravenous injection.
The study by Batrakova and Kabanov reported that drug-encapsulated Pluronic nanocarriers can be delivered across the blood-brain and intestinal barriers, and toxicity studies showed that Pluronic has low in vivo toxicity and can be safely used in human subjects at low concentrations (R.M. Ottenbrite and R. Javan. in Encyclopedia of Condensed Matter Physics; 2005). A Pluronic-based platform for drug delivery therefore holds enormous promise in the field of nanomedicine.