Introduction
Food allergies are adverse responses to components within the foods we eat that result in a self-damaging response from our immune system. These components are usually proteins or glycoproteins. The results of this misguided immune reaction can include nausea, vomiting, diarrhea, and, in severe cases, death. Most food allergies are associated with eight foods — milk, eggs, peanuts, tree nuts, fish, shellfish, wheat, and soybeans — although many other foods can also cause allergies. Approximately 8% of the US population under age eighteen suffers from food allergies, and recent reports provide evidence that peanut and tree nut allergies — two of the most severe types of food allergies — are becoming increasingly more common (Branum & Lukacs 2008; Sicherer et al. 2010; Gupta et al. 2011). Whereas milk and egg allergies are often outgrown, peanut and tree nut allergies typically persist throughout adulthood (Fleischer 2007). Indeed, the risk of unintended allergen exposure due to accidental ingestion is a constant fear for individuals with life-threatening food allergies. Our intestines (about 25 feet in length) are the largest immunologic organ in our bodies and would cover roughly 100 square feet if spread out; we are still learning what cells and signaling systems regulate their response to the multitude of different foods and potential pathogens that pass through them.
Several questions related to the causes of food allergies and their treatments are currently the focus of intense investigation. Why do some foods but not others cause an allergic response? What similarities exist between the response to food allergens and potential intestinal pathogens or other intestinal microbial flora? Here, we'll introduce you to an innovative approach researchers have taken to uncover important clues about food allergies and a possible new therapeutic mechanism to prevent them.
Food Allergies and Current Research
Food allergies are a type I hypersensitivity response, which means that they are mediated by IgE antibodies. In this situation, these types of antibodies cause an inflammatory response with the release of vasoactive compounds from basophils and mast cells. Are food allergies associated with genetic or environmental factors? The answer is yes, they are associated with both factors. Indeed, several variables have been associated with food allergies, including genetics; how, when, and in what amount the food is first encountered; geographic influences; methods of food processing; and the type of bacteria that normally reside in our gastrointestinal tract (Maleki 2004; Lack 2008; Hong et al. 2009; Round & Mazmanian 2009; Berin & Sicherer 2011; Vereda et al. 2011).
What makes certain foods allergenic, whereas others are not? The term "allergenicity" reflects the ability of a protein or glycoprotein to induce an allergic reaction exemplified by the production of IgE antibodies. Allergenicity is a reflection of several characteristics. Many food allergens belong to the same protein family and have similar structural features, such as the cupin and prolamin superfamilies. In addition, a protein's ability to survive digestion and pass through the intestines intact can affect how it will act as an allergen.
Although therapy for food allergies remains an active area of research and several methods are under evaluation, strict avoidance is the current standard of care (Boden & Wesley Burks 2011). Recent research has advanced our understanding of food allergies, facilitated the development of guidelines for accurate diagnosis, and allowed the characterization of many food allergens from both plant and animal sources (Jenkins et al. 2005; Sampson 2005; Jenkins et al. 2007).
How have researchers learned about food allergies? Some important findings have come from the development of animal models to study food allergens. This research has clarified our understanding of the mechanisms behind food allergies and has aided in the evaluation of potential new therapies (Dearman & Kimber 2009). For example, researchers have discovered that genetically similar pigs exhibited drastic differences in the bacteria that grow in their gut depending on whether the pigs were housed in hygienic (clean) or in natural environments, and these differences correlated with changes in the expression of immune system–related genes (Mulder et al. 2009). Further, mouse models of peanut or tree nut allergies have been used to characterize changes in cytokines and to evaluate potential test therapies (Kulis et al. 2011; Smarr et al. 2011).
Food Sensitization and Tolerance
Our bodies are conditioned to foods during development, and this process requires interactions between structural and cellular components. These interactions establish a delicate balance that allows individuals to become either tolerant of or sensitized to a given food allergen. The conditioning of the gut lining plays an essential role in developing food tolerance, and a complex array of factors within the gastrointestinal tract serves as the cellular and molecular basis of food allergies (Brandtzaeg 2010).
What are some of these factors? One major factor is the activity of dendritic cells, specialized cells in the gut that act as antigen presenting cells. These cells are important for intestinal immune regulation (Coombes & Powrie 2008). Dendritic cells bind potential allergens and act as reporters. The information they present can initiate a signaling cascade that leads to the production of IgE-specific antibodies by B-cell– and T-cell–priming events. Alternatively, the information presented by dendritic cells can induce tolerance to potential allergens. Dendritic cells migrate from the intestinal lining into lymph nodes of the gut, and this travel is essential for carrying information to develop tolerance (Worbs et al. 2006). Exactly how dendritic cells initiate the decision-making process either to generate an immune response or to induce tolerance is an area of intense interest.
Another major factor is a set of surface proteins on dendritic cells called C-type lectin receptors (CLRs). CLRs are highly conserved members of the pattern recognition family of proteins that contain carbohydrate recognition domains, and they contribute to dendritic cell–induced tolerance (Zelensky & Gready 2005). Dendritic cell CLRs interact with potential pathogens by binding to their surface carbohydrates, and this interaction is thought to function in antigen processing. It is an important step in the decision to attack or tolerate a foreign antigen, and new evidence suggests that interactions between CLRs and antigens may provide a route for the establishment of oral tolerance to foods.
A Route to Oral Tolerance
Using a molecular trick, Yufeng Zhou and his colleagues found that food allergens disguised as bacteria can prevent food allergy development in a mouse model system (Zhou et al. 2010). How did they make this exciting discovery? They used a carbohydrate modification of a protein (mannosylation) as a method to mimic the molecular surface of a bacterium. Mannose is a carbohydrate residue often found in terminal positions of long sugar chains attached to microbial glycoproteins, such as those in the bacterium that causes tuberculosis (Mycobacterium tuberculosis), but such highly clustered residues of terminal mannose are not common in humans.
Zhou and his colleagues used two test groups of mice in their experiments. They fed the first test group an unmodified form of the protein allergen bovine serum albumin (BSA), which naturally lacks any carbohydrates, and they fed the second test group BSA to which mannose residues were covalently linked (mannosylated BSA). They fed the mice in the two experimental test groups small doses of one of the two types of BSA for several weeks to allow them to become sensitized (allergic) or not to the protein. Following this sensitization period, they fed the mice a larger amount of unmodified BSA as a challenge to see how their immune systems would react. Whereas the mice previously fed the unmodified BSA during the sensitization period had severe allergic responses to the BSA challenge with high IgE levels, the mice fed the mannosylated BSA during the sensitization period had relatively reduced IgE levels and reduced histamine release, and none had severe allergic responses to the subsequent BSA challenge (Figure 1).

The researchers then asked, could dendritic cells in the gut preferentially endocytose (engulf) the mannosylated BSA? They hypothesized that this could be an important step in establishing the decreased allergic response. To test their hypothesis, they used fluorescent labeling of the different forms of BSA coupled with cell-sorting methods and discovered that their hypothesis was indeed the case: dendritic cells from the gut were able to endocytose the mannosylated BSA better than the unmodified BSA.
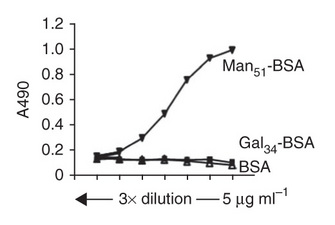
How did the mannosylated BSA cause SIGNR1-expressing dendritic cells to initiate tolerance? To answer this question, they decided to characterize the cytokine expression profiles of the dendritic cells. They showed that treatment of SIGNR1-expressing dendritic cells with mannosylated BSA led to a striking increase in the expression of the cytokine interleukin-10 (IL-10). Next, the researchers suppressed the increase in IL-10 expression by incubating the cells with a blocking anti-SIGNR1 antibody. These results provided evidence that the SIGNR1-dependent pathway was specifically responsible for the release of IL-10.
What role does IL-10 play in the regulation of the immune response? It is actually quite important for the suppression of proinflammatory cytokines primarily associated with macrophages (Cyktor & Turner 2011). The immunosuppressive properties of IL-10 reduce the ability of the immune system to kill pathogens, and IL-10 is important for the control of intracellular bacterial, fungal, and parasitic infections. In some cases, pathogens have developed the ability to exploit IL-10 function to increase their own survival. In addition, researchers have observed an increase in IL-10 expression following pollen allergen immunotherapy that was associated with decreased IgE production (Platts-Mills & Woodfolk 2011).
The Interplay between Dendritic Cells and T Cells
Although the discovery of the SIGNR1-associated increase in IL-10 expression provided new insights into how these dendritic cells initiate tolerance, Zhou and his colleagues carried out additional experiments to further characterize the immune-regulatory signaling events and the role of T cells. They determined that culturing T cells with the SIGNR1-expressing dendritic cells exposed to mannosylated BSA caused the T cells to differentiate into regulatory type 1 T cells with elevated IL-10 and interferon-gamma (IFN-γ) expression levels. What role might IFN-γ play in this process? IFN-γ has immunoregulatory activity and is important for protection against intracellular bacterial and viral pathogens. In line with the results of their earlier experiments, Zhou and his colleagues found that the elevated levels of IL-10 and IFN-γ expression in T cells were greatly reduced when the dendritic cells were incubated with an anti-SIGNR1 antibody. Further, by testing mice deficient for the IL-10 or IFN-γ protein, they learned that the IL-10 and IFN-γ proteins were required for the tolerance-inducing response of the mannosylated BSA.
Next, the researchers asked, could regulatory type 1 T cells isolated from tolerant mice confer tolerance to sensitive mice? Remarkably, when the researchers transferred regulatory type 1 T cells isolated from tolerant mice (treated with mannosylated BSA) to sensitive mice, the cells induced tolerance to unmodified BSA in the previously sensitive mice. This illuminating result showed that these cells are able to retain and transmit the essential information required for tolerance to the allergenic protein.
When combined with findings from other research, the data presented by Zhou and his colleagues suggest a model whereby the binding and activation of the SIGNR1 receptor on dendritic cells by mannose-modified proteins initiate a signaling pathway that can induce tolerance to the bound proteins (Rabinovich 2010) (Figure 3). Although this research provides important clues about a potential route for the development of oral tolerance to food allergens, several questions remain. For example, does the human homolog of SIGNR1, called DC-SIGN, function in the same manner to promote tolerance? What crucial components of the SIGNR1 pathway allow oral tolerance, rather than sensitization, to develop? Can the SIGNR1-dependent pathway be tailored to induce tolerance to other food allergens but still permit the inflammatory pathways for pathogens to remain intact?

Summary
References and Recommended Reading
Berin, M. C. & Sicherer, S. Food allergy: Mechanisms and therapeutics. Current Opinion in Immunology 23, 794–800. (2011). doi: 10.1016/j.coi.2011.08.010
Boden, S. R. & Wesley Burks, A. Anaphylaxis: A history with emphasis on food allergy. Immunological Reviews 242, 247–257 (2011). doi: 10.1111/j.1600-065X.2011.01028.x
Brandtzaeg, P. Food allergy: Separating the science from the mythology. Nature Reviews Gastroenterology and Hepatology 7, 380–400 (2010). doi: 10.1038/nrgastro.2010.80
Branum, A. M. & Lukacs, S. L. Food allergy among U.S. children: Trends in prevalence and hospitalizations. NCHS Data Brief, 1–8 (2008).
Coombes, J. L. & Powrie, F. Dendritic cells in intestinal immune regulation. Nature Reviews Immunology 8, 435–446 (2008). doi: 10.1038/nri2335
Cyktor, J. C. & Turner, J. Interleukin-10 and immunity against prokaryotic and eukaryotic intracellular pathogens. Infection and Immunogy 79, 2964–2973 (2011). doi: 10.1128/IAI.00047-11
Dearman, R. J. & Kimber, I. Animal models of protein allergenicity: Potential benefits, pitfalls and challenges. Clinical and Experimental Allergy 39, 458–468 (2009). doi: 10.1111/j.1365-2222.2008.03194.x
Fleischer, D. M. The natural history of peanut and tree nut allergy. Current Allergy and Asthma Reports 7, 175–181 (2007). doi: 10.1007/s11882-007-0018-y
Gupta, R. S. et al. The prevalence, severity, and distribution of childhood food allergy in the United States. Pediatrics 128, e9–e17 (2011). doi: 10.1542/peds.2011-0204
Hong, X., Tsai, H. J., & Wang, X. Genetics of food allergy. Current Opinion in Pediatrics 21, 770–776 (2009). doi: 10.1097/MOP.0b013e32833252dc
Jenkins, J. A., Breiteneder, H., & Mills, E. N. Evolutionary distance from human homologs reflects allergenicity of animal food proteins. Journal of Allergy and Clinical Immunology 120, 1399-1405 (2007). doi: 10.1016/j.jaci.2007.08.019
Jenkins, J. A. et al. Structural relatedness of plant food allergens with specific reference to cross-reactive allergens: An in silico analysis. Journal of Allergy and Clinical Immunology 115, 163–170 (2005). doi:10.1016/j.jaci.2004.10.026
Kindt, T. J., Goldsby, R. A., & Osborne, B. A. Kuby Immunology, 6th ed. New York, NY: W. H. Freeman, 2007.
Koppel, E. A. et al. Identification of the mycobacterial carbohydrate structure that binds the C-type lectins DC-SIGN, L-SIGN and SIGNR1. Immunobiology 209, 117–127 (2004). doi: 10.1016/j.imbio.2004.03.003
Kulis, M. et al. Single-tree nut immunotherapy attenuates allergic reactions in mice with hypersensitivity to multiple tree nuts. Journal of Allergy and Clinical Immunology 127, 81–88 (2011). doi:10.1016/j.jaci.2010.09.014
Lack, G. Epidemiologic risks for food allergy. Journal of Allergy and Clinical Immunology 121, 1331–1336 (2008). doi: 10.1016/j.jaci.2008.04.032
Maleki, S. J. Food processing: Effects on allergenicity. Current Opinion in Allergy and Clinical Immunology 4, 241–245 (2004). doi: 10.1097/00130832-200406000-00018
Mulder, I. E. et al. Environmentally-acquired bacteria influence microbial diversity and natural innate immune responses at gut surfaces. BMC Biology 7, 79 (2009). doi: 10.1186/1741-7007-7-79
Numazaki, M. et al. Cross-linking of SIGNR1 activates JNK and induces TNF-alpha production in RAW264.7 cells that express SIGNR1. Biochemical and Biophysical Research Communications 386, 202–206 (2009). doi: 10.1016/j.bbrc.2009.06.011
Platts-Mills, T. A. & Woodfolk, J. A. Allergens and their role in the allergic immune response. Immunological Reviews 242, 51–68 (2011). doi: 10.1111/j.1600-065X.2011.01021.x
Rabinovich, G. A. A sweet path toward tolerance in the gut. Nature Medicine 16, 1076–1077 (2010). doi: 10.1038/nm1010-1076
Round, J. L. & Mazmanian, S. K. The gut microbiota shapes intestinal immune responses during health and disease. Nature Reviews Immunology 9, 313–323 (2009). doi: 10.1038/nri2515
Sampson, H. A. Food allergy — accurately identifying clinical reactivity. Allergy 60, 19–24 (2005). doi: 10.1111/j.1398-9995.2005.00853.x
Sicherer, S. H. et al. US prevalence of self-reported peanut, tree nut, and sesame allergy: 11-year follow-up. Journal of Allergy and Clinical Immunology 125, 1322–1326 (2010). doi: 10.1016/j.jaci.2010.03.029
Smarr, C. B. et al. Antigen-fixed leukocytes tolerize Th2 responses in mouse models of allergy. Journal of Immunology 187, 5090–5098 (2011). doi: 10.4049/jimmunol.1100608
Srivastava, V. et al. Toll-like receptor 2 and DC-SIGNR1 differentially regulate suppressors of cytokine signaling 1 in dendritic cells during Mycobacterium tuberculosis infection. Journal of Biological Chemistry 284, 25532–25541 (2009). doi: 10.1074/jbc.M109.006221
Takahara, K. et al. C-type lectin SIGNR1 enhances cellular oxidative burst response against C. albicans in cooperation with dectin-1. European Journal of Immunology 41, 1435–1444 (2011). doi: 10.1002/eji.200940188
Taylor, P. R. et al. The role of SIGNR1 and the beta-glucan receptor (dectin-1) in the nonopsonic recognition of yeast by specific macrophages. Journal of Immunology 172, 1157–1162 (2004).
Vereda, A. et al. Peanut allergy: Clinical and immunologic differences among patients from 3 different geographic regions. Journal of Allergy and Clinical Immunology 127, 603–607 (2011). doi: 10.1016/j.jaci.2010.09.010
Worbs, T. et al. Oral tolerance originates in the intestinal immune system and relies on antigen carriage by dendritic cells. Journal of Experimental Medicine 203, 519–527 (2006). doi: 10.1084/jem.20052016
Zelensky, A. N. & Gready, J. E. The C-type lectin-like domain superfamily. FEBS Journal 272, 6179–6217 (2005). doi: 10.1111/j.1742-4658.2005.05031.x
Zhou, Y. et al. Oral tolerance to food-induced systemic anaphylaxis mediated by the C-type lectin SIGNR1. Nature Medicine 16, 1128–1133 (2010). doi:10.1038/nm.2201