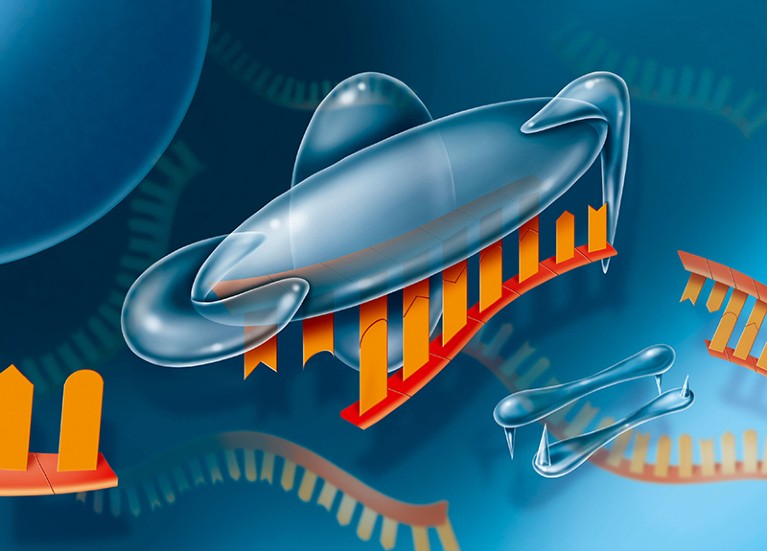
Credit: BSIP SA / Alamy Stock Photo
By the late 1990s, many instances of post-transcriptional gene silencing (PTGS) in plants had been reported. The phenomenon was known to be a defence mechanism that could target endogenous sequences (for example, transposable elements), transgenes or viral RNAs. When Fire and Mello described RNA interference in Caenorhabditis elegans (Milestone 4), it was immediately obvious that PTGS is the plant equivalent of RNA interference.
There are different types of PTGS in plants. Originally reported in petunia, PTGS induced by a transgene with homology to an endogenous gene leads to ‘co‑suppression’ of both the transgene and the endogenous gene (Milestone 2). Transgene-induced PTGS, however, can also occur when the transgene has no homology to endogenous sequences, causing silencing of only the transgene. Systemic PTGS is first triggered locally, then spreads to other tissues. Finally, PTGS can also be mediated by viral RNAs. In all these types of PTGS, cellular accumulation of RNAs with homology to either a transgene or to viral sequences is suppressed, suggesting that the silencing mechanism requires sequence specificity, but the silencing trigger remained elusive.
In 1992, Richard Jorgensen proposed that ectopic pairing of homologous DNA might initiate PTGS. This model was supported by subsequent studies, and antisense RNAs were suggested to initiate silencing by forming a duplex with the targeted mRNA, thereby facilitating its degradation or translation inhibition. However, no antisense RNA molecules with sizes comparable to typical mRNAs had been detected.
In 1999, Hamilton and Baulcombe hypothesized that the antisense RNA trigger might be too short for detection by standard RNA analysis techniques. Therefore, they designed experiments to specifically hunt for short antisense RNAs in the different types of PTGS in plants. Strikingly, they detected 25‑nucleotide (nt) antisense and sense RNAs in two tomato lines that exhibited transgene co‑suppression of the ACO gene. These RNAs were absent in control plants. In tobacco plants carrying a GUS transgene without sequence homology to endogenous sequences, 25‑nt antisense RNAs were also specifically detected in plants that exhibited PTGS. Furthermore, by inoculating a single leaf of a GFP-transgenic Nicotiana benthamiana plant with Agrobacterium tumefaciens containing GFP sequences in a T‑DNA vector, the researchers observed systemic silencing of GFP and detected 25‑nt GFP antisense RNAs in all affected tissues. Finally, in N. benthamiana infected by potato virus X, 25‑nt antisense RNAs were also found only in infected leaves. Thus, 25‑nt antisense RNAs were present in all types of PTGS examined.
As 25‑nt RNAs are long enough to confer sequence specificity, but are short enough for transport through plasmodesmata, Hamilton and Baulcombe proposed that these small RNAs are the likely triggers of PTGS. However, they did not know at the time how the small RNAs were generated. The antisense character of the 25‑nt RNA species and its presence in RNA-virus-infected cells indicated that these short RNAs were generated from an RNA template rather than a DNA template. Only a little while later, scientists working in animal systems reported that long double-stranded RNA (dsRNA) is processed into 21–23‑nt dsRNA fragments that guide mRNA cleavage in vitro (Milestone 7). In vivo evidence followed, demonstrating that long dsRNAs are cleaved to about 25 nt and that the antisense strand triggers mRNA degradation by pairing to target mRNAs. Together, these studies established low-molecular-weight RNAs as the trigger of PTGS and RNA interference, and showed that pairing of the antisense strand with the target mRNA mediates mRNA degradation, but the molecular machinery involved remained to be discovered.