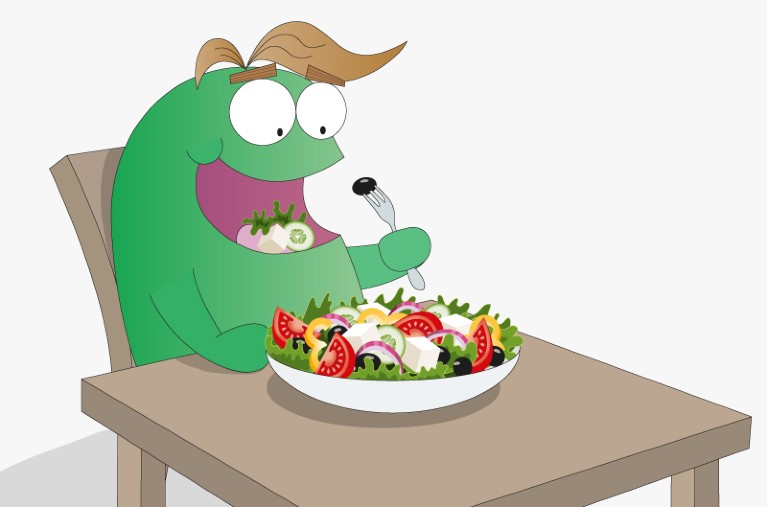
Credit: P. Patenall / Springer Nature Limited
Most complex plant polysaccharides are not digested by humans and enter the colon as a potential food source for the microbiota. But, in the late 1970s, the extent to which gut bacteria could metabolize this dietary fibre was largely unknown.
To bridge this gap, Abigail Salyers and colleagues tested the ability of a wide range of anaerobic bacterial species resident in the human colon to ferment plant polysaccharides as well as intestinal mucins (glycosylated proteins that line the gut epithelium). They found that the bacterial strains had a diverse and inducible ability to break down different substrates, with the largest variety of polysaccharides fermented by Bifidobacterium and Bacteroides species. The researchers proposed that by altering availability of preferred bacterial food sources in the host diet — such as limiting fibre intake — could trigger induction of enzymes capable of degrading the intestinal mucin layer, affecting human health and even colon cancer.
But it was not until 2005 that Jeffrey Gordon’s group demonstrated that a change in diet in a mammal could alter the degradative activity of the colonic microbiota in vivo. By colonizing the gut of germ-free mice with Bacteroides thetaiotaomicron — which Salyers and colleagues had identified as a human symbiont capable of fermenting a wide range of glycan substrates — the researchers tested, in a physiologically relevant setting, the effects of different diets on expression of bacterial genes.
In mice fed a fibre-rich diet, B. thetaiotaomicron genes involved in polysaccharide metabolism were significantly upregulated compared to their expression in bacteria grown in a minimal medium. By contrast, in mice fed a diet devoid of complex polysaccharides, the most highly upregulated bacterial genes were those involved in host glycan degradation. The work confirmed in a vertebrate the differential expression of bacterial enzymes dependent on food source, exposing a flexible network of genes for harvesting glycans based on their availability in the host.
Subsequent work on B. thetaiotaomicron elucidated many of the gene clusters and pathways involved in polysaccharide metabolism that enable bacteria to use the diversity of glycans provided by a mammalian diet and the host itself. This ability to harvest host glycans, such as during fluctuations of a host’s diet, was shown to critically affect survival of B. thetaiotamicron in mono-colonized mice fed a fibre-free diet. Such findings, as well as later studies, underscore the important interplay of host diet and glycan metabolism for gut colonization of human commensals and their persistence in populations over time.
We now know that gut microorganisms harbour thousands of genes involved in catabolism of carbohydrates, but how this enzymatic breadth was acquired remains unclear. One potential source of genetic diversity is horizontal transfer of genes from environmental microorganisms to gut bacteria. In a search for enzymes expressed by a marine Bacteroidetes that degrade sulfated polysaccharides found in edible seaweed (such as nori), porphyranases were identified that had a homologue in a gut bacterium, Bacteroides plebeius. Additional porphyranases and agarases were subsequently identified in the microbiomes of Japanese but not North American individuals. The findings suggest that transfer of genes from marine bacteria on nori was the likely origin of enzymes in the human gut that diversify the ability of bacteria to harvest energy from food sources, such as algal polysaccharides in seaweed, a major dietary component in Japan.
But do the nutrient foraging properties of gut microbiota have a role in human health? Results in mice suggest they may. A fibre-free diet in mice reduced the thickness of the colonic mucus layer, as predicted, and increased susceptibility to disease caused by a mouse enteric pathogen. In another study, the microbial production of short chain fatty acids from dietary fibre influenced mouse lung disease and immune responses. And microbial metabolism of other components of the human diet, such as L-carnitine in meat, has been linked to atherosclerosis.
Much remains to be understood of the impact of diet and the microbiota on human health and disease. But these studies shed light on the dynamic effect of altering host diet on the makeup and behaviour of microorganisms in the gut and other organs, with potential implications for disease modification and treatment.